It is estimated that 90% of brain injuries fall into the category of mild traumatic brain injury (TBI). Mild TBI and concussion can have heterogeneous symptoms and serious consequences that develop over time with unpredictable levels of recovery. The diagnosis may not be made for multiple reasons, including due to patient hesitancy to undergo neuroimaging and inability of imaging to detect minimal damage. Biomarkers of nerve damage measured in blood plasma are increasingly promising as the sensitivity and accuracy of quantification improves. These biomarkers could fill the gap, making detection, diagnosis and prognosis easier and more precise, but the time needed to send blood to a laboratory for analysis made this impractical until point-of-care measurement became available. A handheld blood test is now on the market for diagnosis of concussion based on the specific blood biomarkers glial fibrillary acidic protein (GFAP) and ubiquitin carboxyl terminal hydrolase L1 (UCH-L1). Other blood biomarker assays are in development.
1. Introduction
Traumatic brain injury (TBI) is a neurophysiologic event experienced by an estimated 1.5 million Americans every year according to the Centers for Disease Control and Prevention (CDC)
[1]. Mortality across all TBI severities is approximately 3%. While TBI is well known to cause cognitive, motor, and behavioral symptoms, both acutely and as long-term complications, its morbidity is difficult to quantify. Although most TBI is characterized as mild, even these less severe head injuries can bring long-term sequelae
[2]. Understanding the severity of TBI and establishing proper diagnostic methods for TBI is critical for the patient’s functional recovery and for structuring a personalized rehabilitation plan
[1][2]. Traumatic axonal injury (TAI) comes with high variability in type and degree of neurological impairment, with injury location a significant determinant of prognosis
[3]. TAI refers specifically to damage to axons in the white matter that causes microscopic pathologic brain lesions and manifests as axonal tearing, axonal swelling, and impairment of transport along the axon
[4][5]. This type of damage comes about due to the rotational and acceleration/deceleration movements of the head during TBI
[6]. TAI has been documented at autopsy in persons who have sustained mild TBI and died of other causes
[7]. Newer imaging techniques allow diagnosis of TAI in mild TBI.
2. Current Modes of Mild TBI Evaluation
2.1. Glasgow Coma Scale
Early and accurate TBI diagnosis is important for the detection of any brain injuries that may lead to long-term issues. However, the heterogeneity of TBI signs and symptoms makes characterization difficult. A tool that is often used clinically is the Glasgow Coma Scale (GCS), which sums scores from three categories including best eye response, best verbal response, and best motor response to yield a score between 3 and 15, with scores between 13 and 15 considered a mild TBI
[8]. Although the GCS is a solid basic classifier of TBI severity, it is not perfect and may lead to underestimation of TBI damage
[9].
2.2. Imaging by Computed Tomography
Various imaging methods such as computed tomography (CT) and magnetic resonance imaging (MRI) can be used promptly to determine structural damage
[10]. Non-contrast CT is one modality for diagnosing TBI that is available in acute settings
[11]. In the emergency department, clinicians can decide whether to perform a non-contrast brain CT after completing various tests of neurocognitive function, visuomotor function, and balance
[12]. CT is often normal in mild TBI and is largely used to visualize the presence and location of both extra-axial and intra-axial hemorrhages
[13][14][15]. The development of novel computer-aided diagnosis systems that utilize non-contrast axial CT brain images to detect hematoma, raised intracranial pressure, and midline shift can be useful for the early management of TBI
[16][17].
Widely used CT-based classification systems for standardized interpretation of brain images include the Helsinki, Rotterdam, and Stockholm systems. Rotterdam scores are determined by the status of the basal cisterns, whether there is a midline shift, the presence of an epidural mass lesion, and the detection of intraventricular or subarachnoid hemorrhage
[18][19]. The Stockholm score uses midline shift as a continuous variable, a separate scoring for traumatic subarachnoid hemorrhage, and is the only scoring system that takes diffuse axonal injury into consideration
[20]. The Helsinki scoring system looks at lesion size and type, whether there is intraventricular hemorrhage and the condition of suprasellar cisterns
[21].
There has been growing concern about the missed identification of mild TBI and the risk of developing intracranial lesions that could require neurosurgical intervention
[22]. Even when there is no need for surgery and no imaging abnormality, between 10 and 30 % of mild TBI patients experience persistent post-concussion symptoms lasting 3 months or more that interfere with quality of life and day-to-day functioning
[23]. Protocols for managing mild TBI are a work in progress, with an increasing focus on brain injury biomarkers for prognostic utility
[24]. Although some of these biomarkers correlate with mortality and outcome, further research on their clinical utility needs to be conducted
[25][26].
2.3. Magnetic Resonance Imaging
MRI is a noninvasive imaging technique that uses non-ionizing electromagnetic radiation to provide useful information on brain anatomy and structure, while functional MRI can go further by capturing changes in brain function, blood flow, and connectivity
[27][28][29]. MRI has a higher sensitivity than CT and is useful in mild TBI to detect subtle findings such as small lesions, contusions, and intracranial bleeds
[30].
TAI cannot be perceived with CT, and sensitivity is low for standard MRI. However, an MRI technique known as diffusion tensor imaging, which uses the diffusion of water molecules as they move along the nerves to visualize and trace the fiber tracts in white matter, can detect TAI
[31]. The microstructural damage detected by diffusion tensor imaging and reflecting TAI is believed to be a key factor in predicting prolonged symptoms after concussion or mild TBI
[32].
Figure 1 summarizes the key factors involved in TBI assessment.
Figure 1. Major components in the assessment of TBI severity. Red area represents site of injury.
2.4. Evaluating Concussion: Emphasis on Sports-Related Concussion
Those who participate in sports are known to be vulnerable to concussion, and this affords a unique opportunity for the collection of pre- and post-concussion data and monitoring. Sports-related concussions are a special situation because they have been the focus for the development of protocols for evaluation and practices on return to play post-concussion
[33]. Sports-related concussions are frequent and tend to occur in younger populations, including adolescents and children
[34]. They are considered a mild form of TBI, and the lack of consensus on the diagnostic criteria of sports-related concussion contributes to a poor pathophysiological understanding of the injury
[35].
The Canadian CT Head Rule is a validated clinical decision algorithm designed to reduce unnecessary CT scan utilization after head injury
[36][37] The five high-risk criteria on which the score is based are as follows: GCS (score lower than 15 at 2 h post-injury), skull fracture (open or depressed), signs of basal skull fracture, vomiting 2 or more times, aged 65 years and above.
3. Point-of-Care Cognitive Tests for Mild TBI
Point-of-care cognitive testing assessment methods for mild TBI that do not involve a blood draw are readily available and often employed. The King–Devick is a well-known, inexpensive, easy-to-administer test often used on athletes
[38][39]. The test takes only minutes to complete and measures rapid number reading and naming, which broadly indicates the state of cognitive function, visual–motor coordination, and language. Symptom checklists such as the Post-Concussion Symptom Scale and the SCAT as well as brief paper-and-pencil tests such as the Standardized Assessment of Concussion are also easy to administer
[40][41]. The Montreal Cognitive Assessment (MoCA), which contains 12 individual tasks grouped into seven cognitive domains: (1) visuospatial/executive; (2) naming; (3) attention; (4) language; (5) abstraction; (6) memory, and (7) orientation, has also been used to screen TBI patients
[42][43].
Computerized testing is also being employed, and there is flexibility in the administration of this type of evaluation as it may be done either supervised or unsupervised
[44]. The CogState Brief Battery (CBB) is a computerized test that measures four core cognitive domains: processing speed, attention/vigilance, visual learning, and executive function
[45][46].
4. Blood Biomarkers for Mild TBI
The Food and Drug Administration (FDA) has given clearance to Abbott’s Alinity i TBI test, a commercially available blood test to evaluate and triage concussion patients in an acute setting. What does this really mean for clinical medicine? The FDA previously approved Abbott’s i-STAT TBI Plasma test in 2021
[47][48]. The purpose of these tests is to screen patients aged 18 years and above who present with an acute head injury and deter-mine without delay in which patients a CT scan of the brain is warranted.
Although the radiation-sparing and expense-saving goals aspired to are commendable and consequential, the execution has suffered hindrances, mostly based on the entrenched nature of CT usage in emergency departments. Despite approval of Abbott’s i-STAT TBI Plasma test, it is unclear whether emergency department physicians have changed their use of head CTs. Resistance to the adaptation of blood biomarkers for concussion evaluation may be a major obstacle. Whether or not Abbott’s Alinity i TBI test or any blood biomarker can transform mild TBI assessment is not yet known
[49][50].
The handheld point-of-care analyzer needed for Abbot’s i-STAT TBI Plasma test is expensive and may not be on hand in many hospitals
[51][52]. Further, a study by Larcher et al. found that its results for some blood measures had a level of imprecision that should cause caution in interpretation
[53].
While there are robust data in the medical literature, a huge investment in medical education might be necessary to alter standard emergency department practice patterns. Convenience is also critical. The CT scanner is already situated in the hospital, and the radiology staff remains on-site with scan interpretation accessible in person or by virtual means. There is no new investment in equipment or personnel needed. The results are intuitively reassuring; if the radiologist clears the scan, the patient can be discharged.
What is the new hope presented with the recent approval of Abbott’s Alinity i TBI test? The new test can be performed on Abbott’s Alinity i laboratory instrument, as an alternative to the previous i-STAT TBI Plasma test. The Alinity i instrument is in wide use in hospitals within and beyond the USA for its ability to measure a variety of hormones, antigens, and other compounds using a chemiluminescent microparticle immunoassay
[54].
The two biomarkers measured in the simple Alinity i TBI blood test are glial fibrillary acidic protein (GFAP), the key intermediate filament protein in astrocytes, and ubiquitin C-terminal hydrolase L1 (UCH-L1), a neuronal enzyme that marks proteins for degradation. Both of these are released by the brain into the peripheral blood when the brain is injured
[55][56][57].
5. Potential Expanded Use of Point-of-Care Biomarker TBI Testing
Persons who have suffered a TBI and reach an emergency department or other facility equipped with the analyzer within the delimited time period are considered candidates for Abbott’s new Alinity i TBI test. The biomarker levels in these patients could serve as a source of valuable data, whether or not the CT scan is obtained in tandem. The presence or levels of GFAP and UCH-L1 cannot reliably discriminate between patients who will improve rapidly versus those with prolonged recovery time who may continue to experience troubling neurologic symptoms [58]. Currently, there is no test with that capability [59][60]. This poses a challenge, especially as a majority of TBI patients have no follow-up scheduled. A positive blood test might suggest that follow-up care is indicated, but scholars propose that TBI biomarker data obtained where healthcare is provided such as the Alinity i TBI test can give the medical community so much more. Analysis and integration of data aggregated in emergency departments performing TBI biomarker testing could produce a massive database that would allow the development of a predictive model so that those at the highest risk for poor recovery could be targeted for early intervention. Sports provide a unique advantage in data collection with an opportunity to obtain preseason baseline tests so that within-player change in plasma biomarkers with TBI could be accurately captured [61].
In addition, the lack of effective treatment for mild TBI beyond rehabilitation and symptomatic relief makes the idea of applying the Alinity i TBI test data to acquire a more complete understanding of damage development very appealing [62][63]. This type of understanding is necessary if a breakthrough is to come, whether through the repurposing of current drugs or the application of new gene-expression-altering technologies to interrupt a program of neuronal destruction set in motion by the head injury [64] (Figure 2).
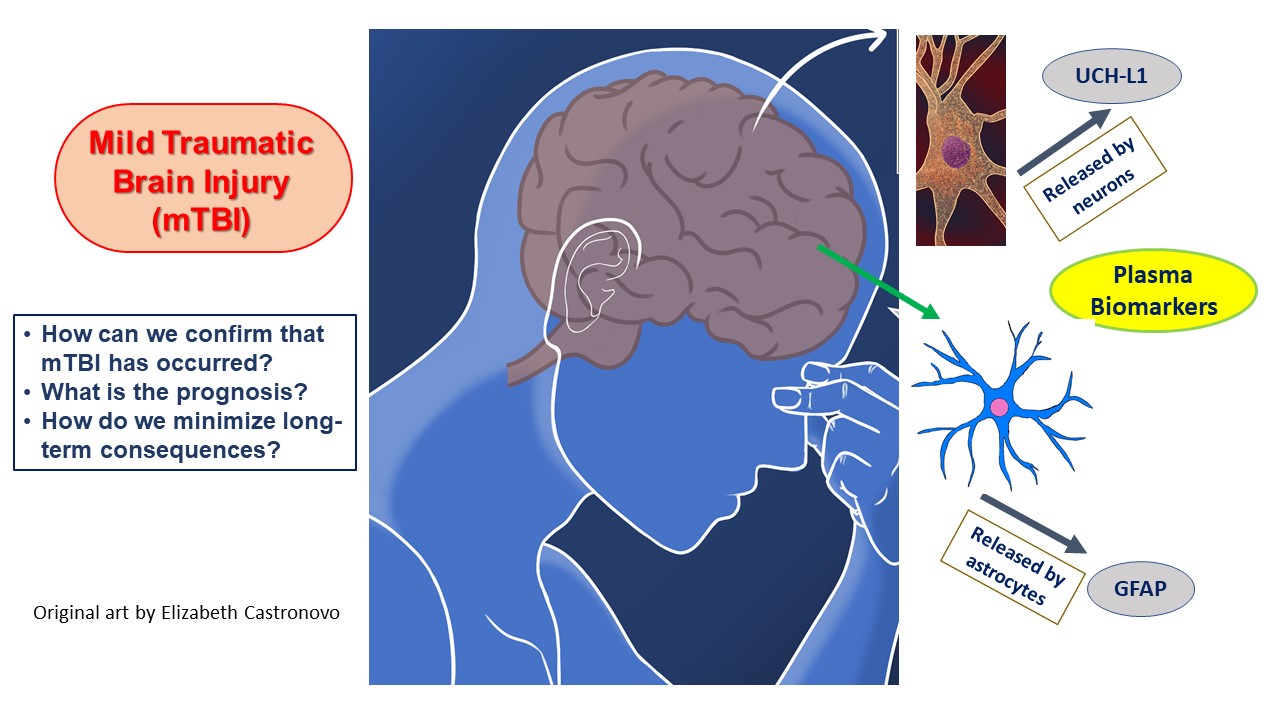
Figure 2. The blood biomarkers utilized in the Alinity i TBI test and delineates the major issues involved in mild TBI diagnosis and management.
Yet another consideration in biomarker use in TBI is the lack of any validated assessment for those under age 18. The absence of representation of the pediatric concussion patient is a major gap since the developing brain is particularly susceptible to injury and prolonged sequelae [65][66]. In older persons, baseline levels of biomarkers are higher, and discriminative capability may be less or of shorter duration after injury [60][67][68]. In persons with Alzheimer’s disease, GFAP levels may be elevated [69]. The need to devise age-appropriate TBI biomarker tests is clear.
The value of the Alinity I test in TBI patients who present after the recommended testing window is somewhat in question. Although biomarker levels lose their ability to distinguish who should receive imaging, that does not preclude using the data to develop a model that will give us prognostic information concerning the chance that long-term neurologic consequences will occur [47][70][71].
6. Conclusions
There is not a clear consensus on diagnostic assessments for TBI, and CT scans are often used because of their availability in acute settings. As described, CT scans are useful for visualizing the presence and location of hemorrhages but are not as sensitive as MRIs for axonal injury visualization. It is also unclear how clinically useful these neuroimaging techniques are for long-term prognosis and quality of life. Since delaying the diagnosis of mild TBI can lead not only to prolonged recovery time, but also to premature return-to-activity and dangerous repeat concussion, it is important to refine blood biomarkers and apply them in real world settings
[72]. Abbott’s Alinity i TBI test may be beneficial in evaluating common biomarkers released upon brain injury such as GFAP and UCH-L1. The newfound increasing availability of Abbott’s Alinity i laboratory instrument contrary to the previous i-STAT TBI Plasma test demonstrates the potential cost-effectiveness of the test and its usefulness in detecting lasting brain damage. This development brings a new opportunity for TBI research to grow and make useful conclusions for long-term physiological and neurocognitive prognosis. The ultimate goal would be to use aggregate data to pinpoint those mild TBI patients with the highest risk of long-term debilitating neurologic problems and then implement mitigating actions to avert these consequences. Finally, scholars may be able to move beyond blood biomarkers to even less invasive salivary biomarkers of concussion. This is in early stages of study with interest in using salivary microRNA measures to evaluate mild TBI
[73][74].
This entry is adapted from the peer-reviewed paper 10.3390/diagnostics13213330