1. Plant Growth Promotion and Yield Improvement in Rice by PNSB
Auxins are plant growth hormones that are produced by plants naturally. Indole-3-acetic acid (IAA) is an auxin that assists in plant development and regulates several plant growth and behavioral processes throughout the plant’s life cycle
[1][27]. Among other bacteria, four
purple non-sulfur bacteria (PNSB)PNSB strains (KK415, TN110, TN217, and TN414) also produce IAA
[2][37], which assists in plant growth and development. IAA produced by PNSB helps to activate cell roots and plant mineral uptake
[2][37], stimulates root formation and seed germination, enhances fructification and vegetative growth, improves biosynthesis of compounds and photosynthesis, and assists in coordinating plant growth under stress conditions
[3][4][5][6][7][8][38,39,40,41,42,43].
Few studies were done on rice to examine the potential of PNSB inoculation on crop growth and yield. A study by Kobayashi & Haque indicated that rice cultivated with
Rhodopseudomonas capsulatus (
R. capsulatus) supplied as PNSB powder to soil with 0.5 g of N, P, and K once during the reproductive phase increases the grain yield of rice
[9][44]. The study indicates that the grain yield increase was due to the production of uracil and proline by the PNSB, whereas the soil detoxification by the PNSB led to an increase in soil fertility, thus improving plant growth. Another study on rice using
R. capsulatus and
Azotobacter vinelandii (
A. vinelandii), with 600 mg and 60 mg protein, respectively, in hydroponic growing media, shows that the flowering and panicle formation was shortened to 100 days after germination with increased size and volume of root hairs
[10][45].
Yoshida et al. inoculated
R. capsulatus in compost at a final concentration of 10
9 cells g
−1 twice in a field trial, resulting in an increased rice yield and ear number
[11][46]. Soaking rice seedlings for 30 min in
R. capsulatus cell suspension increases dry weight, plant height, grain yield, and straw and grain nitrogen content
[12][47]. Studies have also shown that inoculating the
R. capsulatus in the roots of rice seedlings in the hydroponic plant production system increases the shoot dry weight, shoot height, shoot and root nitrogen content, and root number, and decreases the root length and dry weight
[13][48]. On the other hand, a pot experiment by Harada et al. shows that inoculation of PNSB into the waterlogged paddy field soils increases rice grain yield, with the higher grain yield achieved by the combined application of PNSB and rice straw
[14][49]. When rice seeds were coated with an
R. capsulatus cell suspension (10
8 CFU ml
−1) in 10% (
w/v) gum Arabic solution, it led to an increase in shoot weight and height, straw nitrogen concentration, number of productive tillers, grain yield, number of grains per panicle, and grain nitrogen concentration
[15][50].
Finally, inoculating PNSB through a foliar application on rice crop plants under field conditions of a tropical climate significantly improves growth and yield even under cooler temperatures and low light conditions
[16][6]. This effect was shown in the recent study done by Shan et al., indicating that the inoculation of
R. palustris through foliar application under field conditions improves rice crop tiller number, leaf chlorophyll content, lodging resistance, root length, root dry weight, productive tillers per plant, average grain per plant, grain yield, grain weight, and harvest index.
These studies revealed that PNSB inoculation on plants or soil improves soil fertility and photosynthesis rates, thereby improving plant growth and yield. However, PNSB inoculation alone has little to no effect on plant growth and yield, and as such, fertilizer application is necessary to achieve improved results
[15][50]. Combining
R. capsulatus with chemical nitrogen fertilizers enhances the usage efficiency of synthetic nitrogen fertilizers. Likewise, combining
Rhodopseudomonas palustris spp (
R. palustris) of PNSB with
Bacillus subtilis (
B. subtilis) can further enhance rice yields
[17][51] due to the synergetic effects of their co-inoculation on plant growth. The
R. plaustris is an important strain of PNSB that is used as a biofertilizer
[18][52], while
B. subtilis is a biological control agent that protects plants from phytopathogenic organisms
[19][53]. Their combined effects help to improve the growth of various crop plants. However, excessive crop yield losses due to abiotic stress are a major threat to agriculture
[20][54], and findings have shown that the inoculation of PNSB on crops can help alleviate both plant biotic and abiotic stresses
[21][28].
2. PNSB Helps Alleviate Plant Stress in Rice
The production of reactive oxygen radicals higher than the usual amount in plants is a result of the stresses caused by environmental changes
[22][23][24][25][26][55,56,57,58,59]. As a plant goes through abiotic stresses caused by air pollution, drought, extreme temperature change, heavy metals, herbicides, nutrient deficiencies, salinity, and UV radiation, they accumulate reactive oxygen species (ROS)
[27][28][29][30][31][60,61,62,63,64], which are free radicals and non-radical molecules
[32][65]. These include hydrogen peroxide (H
2O
2), hydroxyl radicals (OH), perhydroxyl radical (HO
2), and superoxide radical anions (O
2−)
[1][33][27,66]. Antioxidant enzymes, such as ascorbate peroxidase, catalase, glutathione reductase, and superoxide dismutase, detoxify the overproduced ROS. However, under extreme saline conditions, the antioxidant enzyme activity is reduced
[1][27], leading to the overproduction of ROS
[34][67] and causing damage to lipids, proteins, macromolecules, and carbohydrates
[33][66]. Therefore, these antioxidant enzymes need to be provided to plants externally, or their synthesis needs to be promoted
[1][27] for direct scavenging of free radicals to increase antioxidative defenses. One of the sustainable ways to promote plant growth and productivity even under abiotic stress conditions is by using microbial biostimulants
[20][54].
The 5-aminolevulinic acid (5-ALA) is one of the promising biostimulants
[35][68] and vital antioxidant promoters
[36][69] that shields the photosynthesis apparatus during stress conditions
[37][70] by acting as a shielding mechanism against ROS. The 5-ALA boosts the tolerance of plants toward salinity
[38][39][40][71,72,73], temperature, drought, low-light stress
[41][74], and biodegradable herbicides
[42][43][75,76] (
Figure 12). On the other hand, as a growth regulator, 5-ALA also regulates plant growth and development at different growth stages
[44][77]. The exogenous application of 5-ALA also assists in chlorophyll accretion, thereby increasing photosynthetic activity in plants
[39][45][72,78]. The 5-ALA is also responsible for enhancing the production of the essential cofactors of CO
2 fixation—ATP and NADPH
[37][70]—and microorganisms can be an important source of 5-ALA for plant production due to the higher price of the commercially produced 5-ALA
[39][72]. The PNSB species
R. palustris, Rhodovulum, and
R. sphaeroides produce 5-ALA
[2][39][46][37,72,79], a plant growth regulator that assists in plant growth and yield and alleviates various abiotic stresses in plants
[47][80].
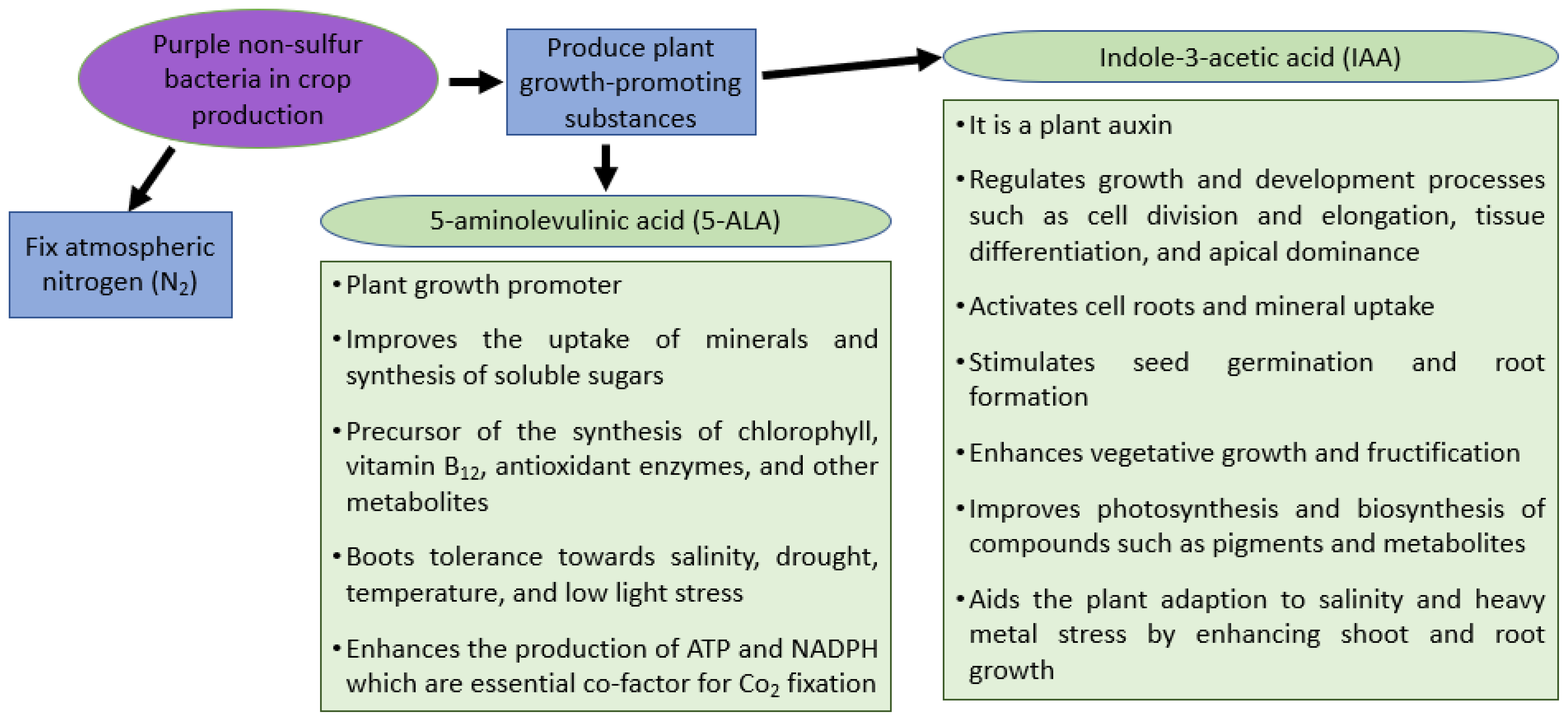
Figure 1. The plant growth-promoting substances produced by the purple non-sulfur bacteria to enhance plant growth and yield.
Kantha et al. studied rice inoculated with
R. capsulatus under salt stress conditions and concluded that using PNSB product can reduce the inhibition of rice husk carrier and rice straw and increase root dry weight, root length, shoot dry weight, and plant height
[48][81]. On the other hand, Kantachote et al. applied 0.75 kg ha
−1 of
R. palustris spp of PNSB product fortnightly during the vegetative stage and weekly during the reproductive and maturation phase of rice, revealing that the PNSB has the potential to mitigate salt stress conditions in rice and increase the grain yield and grains per panicle
[49][82].
Moreover, toxic molecules such as amines, hydrogen sulfide, etc., found in soil are metabolized by the PNSB, leading to soil detoxification, thereby helping improve soil fertility and plant growth conditions
[9][44] (
Figure 23). PNSB uses a variety of mechanisms, such as absorption on extracellular polymeric substances (EPS) bound to the outer surface of the cells, within cell accumulation, conversion of toxic to non-toxic compounds through redox transformation, and conjugation in the siderophores, to alleviate stress caused by heavy metals
[2][50][51][52][37,83,84,85].
Figure 2. Summary of the benefits purple non-sulfur bacteria provides to the rice ecosystem when applied to soil or foliar.
A study by Nookongbut et al. reveals that the
R. palustris spp of PNSB can reduce arsenic (As) stress in rice crops by reducing As accumulation, leading to an increased shoot and dry root weight, increase in shoot height, and enhanced photosynthesis rate
[51][84]. The study also concluded that reducing As accumulation in plants makes it possible for rice farmers to produce safer rice for consumption from contaminated paddy fields. Another study showed that the
R. palustris spp of PNSB has the potential to immobilize the lead (Pb) and cadmium (Cd) metals
[53][86], which otherwise, when present in excessive amounts, can inhibit photosynthesis and respiration, thereby reducing rice crop productivity
[54][87]. The study by Yoshida et al. showed that
R. capsulatus spp of PNSB inoculation increases rice yield and ear number and can decrease the damage from hydrogen sulfide (H
2S)
[11][46].
So far, studies on abiotic stress mitigation using PNSB show impressive results. However, more work is needed to prove these results under different growing conditions and soil types. Since rice is grown under field conditions, the performance of this bacteria in mitigating biotic and abiotic stresses should also be studied under field conditions. PNSB has also shown other benefits to the environment and human health, such as the potential to reduce methane (CH4) emissions from the paddy fields, helping ease the problem of global warming (Figure 23).
3. PNSB Inoculation Reduces CH4 Emissions from Rice Fields
Two common problems of waterlogged rice fields (organic or saline) are low productivity and CH
4 emissions
[49][82]. Carbon dioxide (CO
2) and CH
4 are among the most significant greenhouse gasses since the 20th century, contributing to global warming and climate change. As indicated earlier, CH
4 holds a 20–25-times greater potential for global warming than CO
2. Waterlogged rice fields are among the most important anthropogenic sources of CH
4, with around 60 Tg of CH
4 year
−1 contributing to global CH
4 emissions
[55][88].
The study by Kantachote et al. showed that applying 0.75 kg ha
−1 of
R. palustris spp of PNSB on rice fortnightly during the vegetative stage and weekly during the reproductive and maturation phase increased not only the grain yield and grains per panicle but also decreased the CH
4 flux
[49][82]. The PNSB competes with the methane-producing bacteria for a similar substrate and lowers the CH
4 emission by suppressing the methanogens population in the rice field. However, only a single study has been conducted to assess the effectiveness of PNSB in mitigating the CH
4 emission from rice fields; therefore, further research is needed to verify these results.