The use of sunscreens is a recommended practice to protect skin from solar-induced damage. Around 30 UV filters can be used in sunscreen products in the European Union. However, low photostability and putative toxicity for humans and environment have been reported for some UV filters. Therefore, it is important to develop new UV filters with improved safety profile and photostability. Over the last two decades, nearly 200 new compounds have revealed promising photoprotection properties. The explored compounds were obtained through different approaches, including exploration of natural sources, synthetic pathways, and nanotechnology. Almost 50 natural products and around 140 synthetic derivatives have been studied aiming the discovery of novel, effective, and safer future photoprotective agents. Herein, we provide the reader with an overview about UV filters’ challenges and prospects, offering a forward-looking to the next-generation of UV filters.
1. Introduction
Sunlight has several beneficial effects in human, such as the production of vitamin D, and induction of β-endorphin expression, which improve well-being
[1]. However, excessive sunlight exposure is responsible for photo-induced skin damage, namely solar sunburn, hyperpigmentation, photoaging, skin photosensitisation, and skin cancer
[12], when protective measures, namely the use of sunscreen and the use of adequate clothes and accessories, are not adopted
[13]. Photoprotective measures are more ancient than the first sunscreen’s appearance in the 1900s, and ancient civilisations used plant extracts to protect their skin from sunburns for a long time
[4]. Many of the chemical compounds present in natural extracts with photoprotective properties are now part of sunscreens
[2]. In fact, most of UV filters are inspired in natural products, specifically of botanical, animal, or mineral origin
[25]. In 1928, benzyl salicylate was discovery for its photoprotective action against UVB radiation, but it was only commercialised in 1935 in the first sunscreen “Ambre Solaire”
[26][7]. Later, almost 50 years, avobenzone and its derivatives appeared as the first UV filters that ensure protection against UVA radiation
[38]. Currently, in Europe, there are a total of 29 approved UV filters
[49], complying with the regulations that ensure their effectiveness and safety for humans. UV filters can be classified concerning their ability to absorb the UV radiation (UVR), as UVA, UVB or broad-spectrum UV filters (UVA and UVB)
[510]. Additionally, these products can also be branched into organic or inorganic, where organic filters are only capable to absorb the UVR, while inorganic filters can reflect and scatter the UVR
[610]. In recent decades, the safety of UV filters for humans and environment has been called into question. In fact, many studies have confirmed the detection of UV filters in human biological samples
[711][812] and in marine organisms
[913][1014][1115][1216], thus confirming the hypothesis of UV filter-derived toxic effects. The presence of particular chemical moieties in UV filter structures confers intrinsic toxicity
[1317][1418]. Photoinstability occurs for UV filters that photoisomerise or photodegrade, and consequently can generate toxic photodegradation products and loss of photoprotective action
[1519]. Therefore, over the last two decades, new natural products from botanical and marine sources
[1620][1721][1822] and synthetic derivatives
[1623][1924][2025][2124] have been investigated, along with the use of nanotechnology approaches
[626] as strategies to find new, more effective, safer and more stable UV filters.
2. Challenges
The main challenges associated with the UV filters present in sunscreens are their photoinstability, environmental impact, and human toxicity. As mentioned before, these are some points to consider in the development of new effective and safer photoprotective agents.
The stability of sunscreens is an essential requisite to ensure
the photoprotection and safety. UV filters absorb UVR and enter in excited energetic levels
[2227]. Then, the energy is released, and the chemical molecule returns to its initial energetic level. During this process, some UV filters undergo photoisomerisation and even irreversible cleavage of bonds
[2328]. The mechanisms associated with this phenomenon include the formation of photodegradation products, which can negatively influence the sunscreen’s effectiveness. These degraded derivatives could also be toxic due to interaction with the constituents present in cells and/or damage the DNA. Moreover, they can also affect the stability of the other ingredients present in the formulation
[2228][24].
Several approaches could be used in order to ameliorate the photostability of photoprotective agents, such as the introduction of antioxidants
Several approaches could be used in order to ameliorate the photostability of photoprotective agents, such as the introduction of antioxidants , encapsulation , multiple association of UV filters , and the addition of quenching molecules [2934], and the addition of quenching molecules in the sunscreen’s formulation [3035] in the sunscreen’s formulation [3136].
.
The concern about the negative impact of UV filters on environment and organisms is growing day-by-day. The research community is trying to find alternatives and solutions to minimise the risks posed by these actual emergent pollutants
[3237]. The impact that sunscreen agents could have in organisms is relevant, namely in marine organisms. Recently, several studies revealed the dangerous and noxious effects of UV filters towards diverse aquatic species, such as mussels
[938][33][3439], algae
[3540], crustaceans
[3640], corals
[3741][3842][3943][40], sea urchins
[1140], fish
[4144][42][43], and even in dolphins
[4445]. There are two ways of studying the toxicological effects in marine organisms: by determining the concentration of UV filters in a specific organism, by collecting the marine organism in the environment, or through the organism’s exposure to a specific range of UV filters’ concentrations and subsequently verifying the effects
[1216].
In the next sections, the possible effects that UV filters may have in human beings and in the environment, namely in marine organisms, will be described. Figure 1 depicts the main human systems and marine organisms that suffer the negative impact of the UV filter’s toxicity.
Figure 1. Resume of the main systems affected by UV filter’s toxicity.
The occurrence of UV filters in different locations worldwide with concentration values between ng/L and µg/L has been studied in lakes, seawaters, sediments, rivers, estuaries, and in aquatic organisms
[4546]. In fact, the harmful effects of UV radiation leaded to an extensive production and use of photoprotective products during vacancies, which resulted in an increase in UV filters present in an environment, namely in aquatic ecosystems
[46].
It is noteworthy mentioning that the presence of these compounds in marine waters, will after some time deposit in sediments, contributing for the bioaccumulation in both marine and terrestrial organisms, through absorption, abiotic and biotic degradation, hydrolysis, and photolysis [46][48][49]. The contamination of terrestrial environment could also occurred by wastewaters discharges, disposal of product packages in inappropriate locations, and even in indoor dust that drives to an environment issue, contaminating both land and terrestrial organisms
[4647]. Bioaccumulation and toxicological effects are the main issues associated with marine contamination by UV filters that could induce the persistence of these emergent contaminants through the food chain
[4647].
Considering the relevance of this topic, herein some studies reporting the bioaccumulation and negative impact of UV filters in marine organisms are discussed.
3. Prospects
Several improvements in sunscreens were made in recent decades, particularly aiming to obtain new UV filters with increased photoprotective effectiveness, photostability, environmental and human safety, and improved sensory properties
[5048]. With this purpose, many sources have been explored by the scientific community, namely extracts and natural products isolated from both terrestrial and marine sources and new synthetic derivatives.
3.1. Nature as a Source of Potential Photoprotective Agents and UV Filters
Nature has been widely used for many decades, as the main source for the discovery of new bioactive compounds. Considering skin care applications, several botanical and marine organisms’ extracts with photoprotective and antioxidant effects have been reported. Additionally, some natural products isolated from these sources have proved to be promising bioactive compounds.
Every day, plants are exposed to UVR, which increases their resistance to the noxious UV rays. As a result of this natural resistance, secondary metabolites with diversified scaffolds possessing UV photoprotective and antioxidant properties are produced, specially terpenoids, anthocyanins, flavonoids, carotenoids, and phenolic acids
[1620]. Algae, cyanobacteria, bacteria, and marine fungi are some of examples of marine organisms that produce secondary metabolites with photoprotective effects through UV filter and antioxidant activity. In
Table 1, natural extracts and metabolites (36–56) of botanical and marine sources with photoprotective and antioxidant activities are presented.
to be the most promising compounds obtained from botanical extracts, considering their good photostability, photoprotective, antioxidant potential, and non-cytotoxic profile at the concentrations mentioned. Interestingly, the presence of hydroxyl groups is a common structure feature for the most promising secondary metabolites with antioxidant activity. Considering marine-derived metabolites, MAAs, palythine (41), asterina-330 (42), shinorine (43), porphyra-334 (45), and scytonemin (44) have an excellent protective ability against UVR, being 44 an UVA/UVB absorber. Comparing both botanical and marine natural sources, marine-derived extracts and metabolites possess improved ability to protect against photo-induced damage. The antioxidant potential and easily introduction in cosmetic formulations are the main strengths of botanical extracts and metabolites. Despite all the advances on analytic techniques, it is not always possible to identify the active metabolite. Moreover, the isolation and purification of the botanical and marine natural products with photoprotective activity is a time-consuming process, being obtained in a low amount, which is a major drawback for obtaining compounds to be further explored for skin care applications. Therefore, some of these natural products were used as lead compounds to obtain synthetic derivatives with promising photoprotective effects.
Table 1. Natural extracts and metabolites of botanical and marine sources with photoprotective and antioxidant activity.
Organism and Species |
Main Identified Secondary Metabolites |
Activity |
Values |
References |
Botanical Extracts and Metabolites |
Methanolic extract of grape seeds (from Village Farm and Winery; Nakhon Ratchasima, Thailand) |
(+)-catechin (36) and (-)-epicatechin (37) (determined by HPLC)
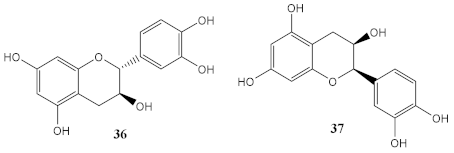 |
Photoprotective (% cell viability) |
At 25 μg/mL 10 J/cm2 (110%) 20 J/cm2 (68%) |
[5133] |
Photodegradation |
36 = 35.1%; 37 = 31.3% Combination with UV filter: 36 (4.6%); 37 (7.0%) |
Hydroethanolic extract of Vitis vinifera L. |
Flavonoids, phenolic compounds, procyanidins, among others (determined by HPLC) |
Antioxidant (DPPH) at 1mg/mL |
707.00 ± 0.03 µmol/g (pH = 5) 1098.00 ± 0.01 µmol/g (pH = 7) |
[5234] |
Photoprotection |
SPF = 20–76 λc = 360–381 nm (pH = 5) |
Ethanolic commercial extract of olive leaves |
20% of oleuropein (38)
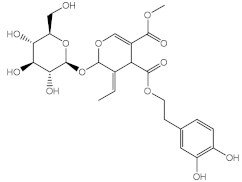 |
Antioxidant (DPPH) |
38: IC50 = 11.75 ± 1.01 μg/mL Extract: IC50 = 13.8 ± 0.8 μg/mL |
[5335] |
Photoprotective |
λmax = 376 nm SPF = 22 |
Ethanolic Extract of varied Lippia species (L. brasiliensis, L. rotundifolia, L. rubella and L. sericea) |
Phenols and flavonoids |
Antioxidant (DPPH) |
IC50 = 0.604 mg/mL |
[5436] |
Photoprotective |
SPF = 1.7–7.6 (formulation with 10% of the extract) λc = 375 nm |
Ethanolic extract of Amazonian Cecropia obtusa leaves |
Polyphenols |
Antioxidant |
IC50 = 1.63 µg/mL (DPPH) IC50 = 0.34 µg/mL(O2−) IC50 = 0.55 µg/mL(1O2) |
[5537] |
Photoprotective |
SPF = 16 |
Cytotoxicity (HaCaT keratinocyte cell line) |
At 20 µg/mL: cell viability = 100% |
Ethanolic extract of Acacia catechu heartwood |
- |
Photoprotective |
SPF = 24–30 |
[5638] |
Hydroalcoholic extract of five wild Brazilian bamboo species (Chusqueaspp., Aulonemia aristulata, and Merostachys pluriflora) |
Phenolic compounds |
Antioxidant (DPPH) |
IC50 = 137.55–260 μg/mL |
[5739] |
Photoprotective |
SPF (before irradiation) = 34–86 SPF (after irradiation) = 14–44 |
Dichloromethane/acetone (1:1) extract from Lasallia pustulata |
Lichenic metabolites, being gyrophoric acid (39) identified by HPLC
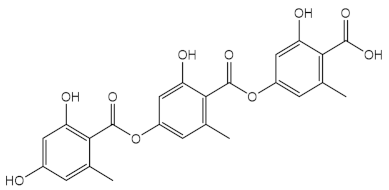 |
Antioxidant (DPPH) |
25 % at 500 µg/mL |
[5840] |
Photoprotective |
λmax = 300 nm SPF = 5.03 |
Cytotoxicity (HaCaT keratinocytes cell line) |
IC50 = 168 ± 33 µg/mL (before radiation) IC50 > 200 µg/mL (after radiation) |
Wood powder |
- |
Photoprotective |
SPF = 11 (formulation) SPF = 37 (formulation + 5% of wood powder) |
[5941] |
Ethanolic extracts of Alpinia galanga, Curcuma longa and Aloe vera |
Flavonoids, phenols and terpenoids |
Photoprotective |
SPF = 18.2 (extract of C. longa) λmax = 290 nm (C. longa) SPF = 15.1 (A. galanga) λmax = 290 nm (A. galanga) |
[6042] |
Coconut oil |
High quantity of saturated fatty acids |
Photoprotective |
λmax = 205 nm (coconut oil) λmax = 320 nm (coconut oil + BP-3) |
[6143] |
Resveratrol (40) and ethanolic extract of green tea |
Resveratrol (40)
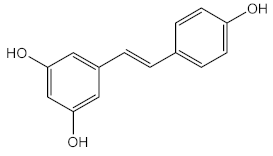 |
Antioxidant (DPPH) |
IC50 = 38.67–85.44 % (resveratrol) IC50 = 37.41–77.50 % (green tea extract) |
[6244] |
Photoprotective |
λmax = 310 nm (40) λmax = 270 nm (green tea) SPF = 9.35 (40) SPF = 14.59 (green tea extract) SPF = 16.91 (40 and green tea extract) |
Marine Organisms Extracts and Metabolites |
Methanolic extract of red macroalgae Curdiea racovitzae and Iridaea cordata |
MAAs, with major quantity of palythine (41), asterina-330 (42), and shinorine (43)
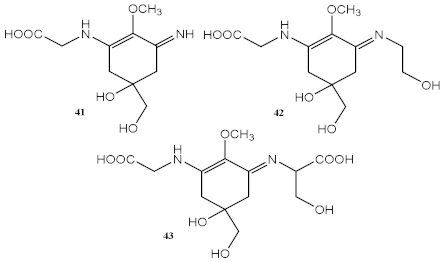 |
Antioxidant (DPPH) |
IC50 = 970.00 μg/mL (C. racovitzae) IC50 = 2960.00 μg/mL (I. cordata) |
[6345] |
Photoprotective |
λmax = 320 nm (both) λc = 356 nm (C. racovitzae) λc = 347 nm (I. cordata) |
Cytotoxicity (HaCaT keratinocytes cell line) |
At 1 mg/mL % cell viability = 89 (C. racovitzae) % cell viability = 73 (I. cordata) |
Ethanolic extract of brown macroalgae Sargassum cristafolium |
Palythine (41) |
Photoprotective |
λc = 370 nm |
[6449] |
Methanolic extract red alga Corallina pilulifera |
- |
Antioxidant (DPPH) |
At 200 mg/mL: 80% scaveging activity |
[6550] |
Metabolite from extracts of cyanobacteria Stigonema sp., Scytonema sp. and Lyngbya sp. |
Scytonemin (44)
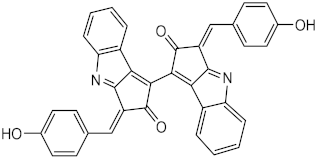 |
Photoprotective |
λmax = 252, 278, 300, 386 nm |
[6651] |
Metabolites from aqueous methanolic extract of cyanobacteria Microcystis aeruginosa |
MAAs shinorine (43) and porphyra-334 (45)
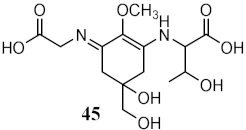 |
Photoprotective |
λmax = 334 nm |
[6752] |
Metabolites from ethyl acetate extract of marine fungi Penicillium echinulatum |
Quinolinic Alkaloids
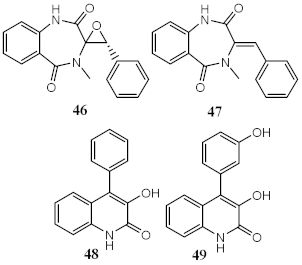 |
Photoprotective |
λmax = 287 (48) λc = 335 nm (48) λmax = 330 (49) λc = 334 nm (49) |
[6853] |
Phototoxicity (HaCaT keratinocytes cells) |
Reduction of ROS (43%) at 200 µg/mL (49) |
Metabolites from dichloromethane/methanol (2:1) extract of algae Bostrychia radicans -associated fungi Annulohypoxylon stygium |
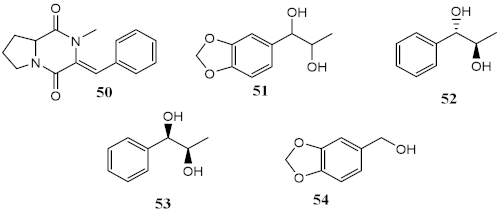 |
Phototoxicity (3T3 murine fibroblasts) |
PIF = 1.00 (50 and 51) PIF = 5.2 (54) |
[6954] |
Metabolite from ethanolic extract of plant Thalassia testudinum |
Thalassiolin B (55)
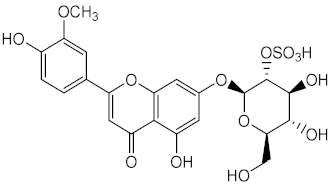 |
Antioxidant (DPPH) |
IC50 = 100 μg/mL |
[7055] |
Repair of Acute UVB-Damaged Skin |
Skin damage suppression (with 55 at 240 μg/cm2) = 90% |
Platyfish Xiphophorus metabolite |
Melanin (56)
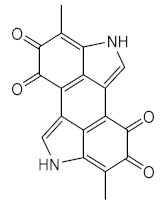 |
Photo-repair of the skin |
Stimulate the production of melanin, which reduced the formation of pyrimidine dimers. |
[7156] |