Global rise of infections and deaths caused by drug-resistant bacterial pathogens are among the unmet medical needs. In an age of drying pipeline of novel antibiotics to treat bacterial infections, antimicrobial peptides (AMPs) are proven to be valid therapeutics modalities. Direct in vivo applications of many AMPs could be challenging; however, works are demonstrating encouraging results for some of them. In this review article, we discussed 3-D structures of potent AMPs e.g., polymyxin, thanatin, MSI, protegrin, OMPTA in complex with bacterial targets and their mode of actions. Studies on human peptide LL37 and de novo-designed peptides are also discussed. We have focused on AMPs which are effective against drug-resistant Gram-negative bacteria. Since treatment options for the infections caused by super bugs of Gram-negative bacteria are now extremely limited. We also summarize some of the pertinent challenges in the field of clinical trials of AMPs.
1. Introduction
At present, antibiotics are the major drugs administered to eliminate infectious diseases caused by bacteria and other microbes. However,
theour dependence on the frontline antibiotics has now been challenged by the steady rise of bacterial antimicrobial resistance (AMR) problems. Although, the emergence of antibiotic resistance pathogens is a natural phenomenon
[1][2][3][1,2,3]. Penicillinase, a bacterial enzyme that hydrolyzes penicillin antibiotic, was identified even before the therapeutic approval of penicillin
[1][2][3][1,2,3]. Notably, resistant bacterial strains could be isolated within few years of the introduction of an antibiotic, for example the first methicillin resistant
S. aureus (MRSA) was detected in 1961 only two years after methicillin was introduced
[1][2][3][1,2,3]. Overuse and misuse of antibiotics over the decades have now been escalated to the occurrence of drug resistant, multi-drug-resistant (MDR) and extremely drug-resistant (XDR) bacterial pathogens. Bacterial AMR has been recognized as one of the major health issues of this century. Commissioned by the UK government, the O’Neill report made a thorough analysis of AMR and provided an estimate of 10 million deaths/year by 2050
[4]. In 2019, Centers for disease control and prevention (CDC) of USA indicated 2.8 million of antibiotic-resistant infections resulting in 35,000 annual deaths
[5]. A very recent comprehensive analyses on the global burden of bacterial antimicrobial resistance in 2019 has reported 4.95 million deaths associated with bacterial AMR including 1.27 million more deaths attributable to bacterial AMR
[6]. The six leading bacterial pathogens
Escherichia coli,
Staphylococcus aureus,
Klebsiella pneumoniae,
Streptococcus pneumoniae,
Acinetobacter baumannii, and
Pseudomonas aeruginosa have caused 929,000 deaths attributable to AMR and 3.57 million fatalities associated with AMR in 2019
[6]. In addition, AMR-associated deaths are found to be prevalent due to infections caused by six other pathogens namely
Mycobacterium tuberculosis,
Enterococcus faecium,
Enterobacter spp.,
Streptococcus agalactiae or group B
Streptococcus,
Salmonella typhi, and
Enterococcus faecalis [6]. Among bacterial AMR, infections caused by Gram-negative strains are the most difficult to be cured
[7][8][9][7,8,9]. Indeed, the World Health Organization (WHO) emphasizes lack of potent drug candidates due to limited discovery of new antibiotics. Most antibiotics are simply modifications of existing drugs and are ineffective against resistant Gram-negative bacteria
[10]. As a matter of fact, Gram-negative bacteria are intrinsically more impervious to several conventional antibiotics compared to Gram-positive bacteria. A trove of frontline antibiotics e.g., vancomycin, rifampicin, erythromycin, novobiocin etc., which can kill Gram-positive bacteria are not used for the treatment of Gram-negative infections
[7][8][9][10][11][12][13][7,8,9,10,11,12,13]. Permeability barrier of the outer membrane LPS and porins can significantly restrict entry of large scaffold antibiotics to the bacterial targets
[7][8][9][10][11][12][13][7,8,9,10,11,12,13]. Hence, there is an urgent need for the discovery and development of novel drugs which will be effective in the treatment of bacterial AMR particularly drug-resistant Gram-negative bacteria.
In the era of bacterial AMR and the drying pipelines of new small molecule antibiotics, antimicrobial peptides (AMPs) provide certain hopes to fight against MDR pathogens
[14][15][16][14,15,16]. AMPs, also termed as host defense peptides (HDPs), are evolutionarily conserved arsenals of all organisms to fend microbial invasion
[17][18][19][17,18,19]. These molecules constitute an integral component of innate immune system in higher organisms. AMPs are often synthesized as large precursor proteins and are released upon digestion caused by host proteases
[17][18][19][17,18,19]. Most AMPs are bequeathed with an impressive broad spectrum of anti-pathogenic activity including Gram-negative and Gram-positive bacteria, fungi, viruses, and parasites
[20][21][22][20,21,22]. In addition, activities akin to immunomodulatory, anti-inflammatory, wound healing, and biofilm inhibition/eradication are also known for some of them
[20][21][22][20,21,22]. By contrast to conventional antibiotics, antibacterial cell killing of AMPs largely emanate from the disruption of membrane structures. The so called “membranolytic” activity occurs due to the formation of amphipathic structures while in complex with negatively charged bacterial membranes
[23][24][25][23,24,25]. Mechanistic actions of AMPs based on plasma membrane disruption processes are proposed e.g., barrel stave, toroidal pore, and carpets
[23][24][25][23,24,25]. Cationic AMPs are often able to permeabilize anionic outer membrane LPS which is a necessary step as a part of the mode of action toward Gram-negative bacteria
[26][27][28][29][30][26,27,28,29,30]. Concomitantly, AMPs can act as potentiators of conventional antibiotics to kill Gram-negative bacteria
[11][31][32][33][34][11,31,32,33,34]. Atomic resolution structures of some of the potent AMPs in complex with outer membrane LPS have revealed correlations with anti-bacterial activity
[35][36][37][38][39][40][35,36,37,38,39,40]. Importantly, activity of AMPs toward antibiotic resistant bacteria is providing an excellent therapeutic avenue for the further development of antimicrobials
[41][42][43][44][45][41,42,43,44,45]. Emergence of bacterial resistance to AMPs has been found to be limited presumably due to unfavorable evolutionary pressure to change membrane compositions
[46][47][46,47]. AMP-derived analogs, AMP-conjugated polymers, and AMP nanoparticles are among the robust candidates as therapeutics against drug-resistant bacteria
[48][49][50][48,49,50].
2. Polymyxins
Polymyxins are a family of cyclic lipopeptides isolated as early as 1947 from spore-forming Gram-positive bacteria
Bacillus polymyxa [51]. Polymyxins are produced from non-ribosomal peptide synthetase system (NRPS) employing enzymes PmxA, PmxB, and PmxC
[52]. The chemical structures of polymyxins, polymyxin B (B1, B2), and polymyxin E (colistin), vary slightly from each other (
Figure 1A,
Table 1). The cyclic structures of both polymyxin B (PMB) and polymyxin E (PME) contain four cationic diamino-butyric acid (Dab) at positions 4, 5, 8, and 9 and a polar residue Thr 10
[53]. PMB contains an aromatic residue D-Phe6, whereas the 6th position in PME is substituted with residue D-Leu.
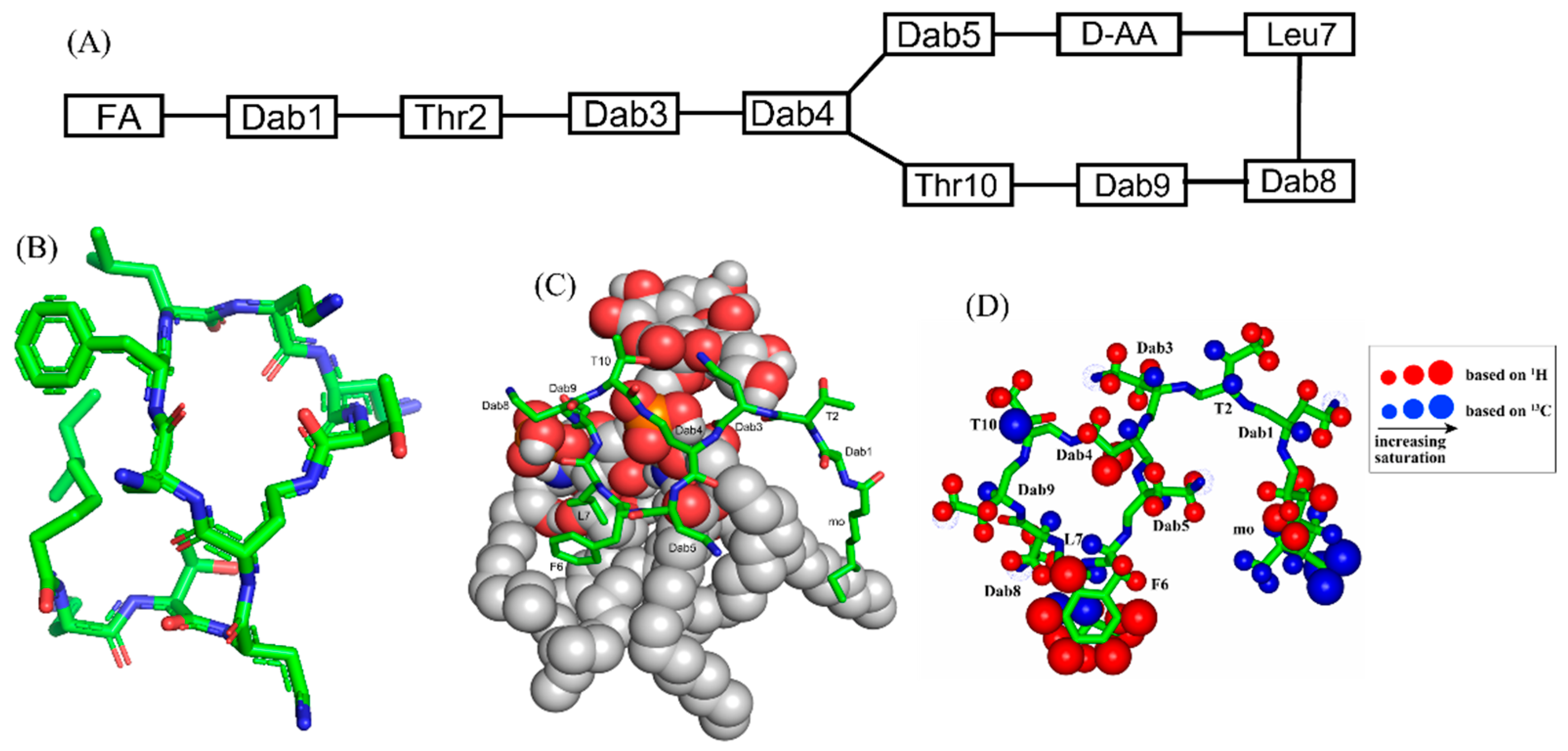
Figure 1. (
A) The primary structure of PMB. Both PMB and PME contains cationic Dabs at positions 4, 5, 8, and 9 along with a polar Thr10 residue in their cyclic structures. (
B) 3-D structure of PMB represented by a stick model
[35]. (
C) The docked structure of PMB-LPS complex shows that the cyclic region of PMB (stick model) binds to lipid A moiety of LPS (space filling model) predominantly by ionic/salt bridge interactions. (
D) Summary of the epitope mapping of PMB in complex of LPS based on
1H 1D STD experiments (red color) and natural abundance
13C-
1H HSQC experiments (blue color)
[54]. The structure of PMB is represented as solid stick. The protons are depicted as sphere of different sizes based on determined STD values. (
D) is reproduced from reference 54 upon permission obtained from the publisher.
Table 1.
Polymyxin B, colistin, and the novel polymyxin based analogs with improved efficacy in animal infection models [51] a,b
.
Compound |
FA |
Sequence |
Polymyxin B (PMB) |
Methyloctanoyl/methylheptanoyl |
Dab1-Thr2-Dab3-cy[Dab4-Dab5-DPhe6-Leu7-Dab8-Dab8-Thr10] |
Polymyxin E (PME, Colistin) |
Methyloctanoyl/methylheptanoyl |
Dab1-Thr2-Dab3-cy[Dab4-Dab5- | DLeu6 | -Leu7-Dab8-Dab8-Thr10] |
FADD002 |
Octanoyl |
Dab1-Thr2-Dab3-cy[Dab4-Dab5- | DAda6 | -Leu7-Dab8-Dab8-Thr10] |
FADD287 |
Octanoyl |
Dab1-Thr2- | Dap3 | -cy[Dab4-Dab5- | DLeu6 | - | Abu7 | -Dab8-Dab8-Thr10] |
CA284 |
( | S | )-1-(2-methylpropyl)-piperazine-2-carbonyl+ |
Thr1-Dab2-cy[Dab3-Dab4-DPhe-Leu6-Dab7-Dab8-Thr9] |
SPR206 |
(3 | S | )-4-amino-3-(3-chlorophenyl)butanoyl |
Thr1-Dab2-cy[Dab3-Dab4-DPhe-Leu6-Dab7-Dab8-Thr9] |
MicuRx-12 |
3-(2,2-dimethyl-butanoyloxy)-propanoyl (ester bond) |
Dab1-Thr2-Dab3-cy[Dab4-Dab5-DPhe6-Leu7-Dab8-Dab8-Thr10] |
NAB379 |
Octanoyl |
Thr1- | DSer2 | -cy[Dab3-Dab4-DPhe5-Leu6-Dab7-Dab8-Thr9] |
NAB815 |
Octanoyl |
Dab1-Thr2- | DThr3 | -cy[Dab4-Dab5-DPhe6-Leu7-Abu8-Dab8-Thr10] |
a Amino acyl residues that differ from those in polymyxin B are marked in Red,
b Abu, aminobutyryl; Ada, aminodecanoyl; Dap, diaminopropionyl; cy, cyclic region indicated with brackets; Dab, diaminobutyryl; FA, fatty acyl.
The linear tri-peptide segment of PMB and PME consists of residues Dab1-Thr2-Dab3. The sidechain amine group (NH
2) of residue Dab4 forms a peptide-like covalent bond with the carboxylate group of residue Thr10 conferring 23-atoms heptacyclic ring. Residue Dab1 of PMB and PME is acylated with fatty acids either methyloctanoyl (MeC8) or methylhaptanoyl (MeC7) named as variants PMB1/PME1 or PMB2/PME2, respectively. Polymyxins are highly effective antibiotic peptides against many Gram-negative strains including
E. coli,
P. aeruginosa,
K. pneumoniae,
A. baumannii. However, clinical use of polymyxins was abandoned in sixties due to observed nephrotoxicity upon discovery of safer new antibiotics
[55]. In recent years, despite the known toxicity, polymyxins are reintroduced in clinical usage as a last resort drug option for the hard-to-treat infections of multi-antibiotic-resistant Gram-negative bacteria
[51][56][57][58][59][51,56,57,58,59]. Optimal dosages and guidelines are recommended for the administration of polymyxins that may potentially reduce acute kidney injury
[56][57][58][59][56,57,58,59]. In this respect, PMB could be considered as a suitable therapeutic option in comparison to PME or colistin methanesulphonate. As PMB demonstrates a lower level of nephrotoxicity and can be retained at a higher plasma level required of the treatment of pathogenic infections
[60]. However, non-toxic analogs of PMB with high anti-bacterial activity are essential and some analogs of PMB were tested in clinical trials (
Table 1). Among these derivatives, NAB739 and NAB815, developed by Vaara and Northern Antibiotics are well characterized and were found to be less nephrotoxic with the ability to kill drug-resistant Gram-negative bacteria
[51][58][61][51,58,61]. Notably, in these analogs two cationic Dab residues, Dab1 and Dab3, were either substituted or deleted achieving lowered toxicity. SPR206, in phase I clinical trial by Spero therapeutics, a more potent analog, contains a shorter linear part and a different composition of fatty acyl chain
[51][58][61][51,58,61]. SPR741 a unique derivative of PMB which lacks much of the anti-bacterial activity, however, acts as a strong antibiotics potentiator. Design of SPR741 is akin to PMB nonapeptide (PMBN) which is known to sensitize outer membrane LPS of Gram-negative bacteria
[58][59][58,59]. SPR741 has passed phase I clinical trial and now in further development.
3. β-Sheet AMPs: Protegrins and Thanatin
β-sheet or β-hairpin AMPs are well-folded even in the absence of bacterial membrane, whereas helical AMPs often lack folded conformations in free solution. The stable β-sheet structures are often supported by inter-strand disulfide bond(s). Protegrins (PGs) constitute 16–18-residue long Arg rich AMPs identified from leucocyte of pig (
Figure 2). The β-hairpin structures in PGs are maintained by two antiparallel β-strands with disulfide bonds between residues Cys6-Cys15 and Cys8-Cys13. Broad spectrum antibacterial activity under physiological salt solutions had drawn considerable attention for therapeutic developments of PGs
[62][63][68,69]. Where PG-1 represents the well-studied member of the group. PG-1 displayed low minimal inhibitory concentration (MIC), ranging between 0.5 to 5 μM, against several strains of Gram-negative and Gram-positive bacteria
[62][63][64][65][68,69,70,71]. Iseganan or IB367 is a synthetic variant of PG-1 developed by IntraBiotics Pharmaceuticals through extensive structure activity studies or SAR
[66][72]. IB367 was subjected to several phases of clinical trials targeting number of conditions e.g., oral mucositis in cancer patients, prevention of ventilator associated pneumonia, cystic fibrosis and mycoses
[67][68][73,74]. However, the status of these clinical trials of iseganan at present is unknown.
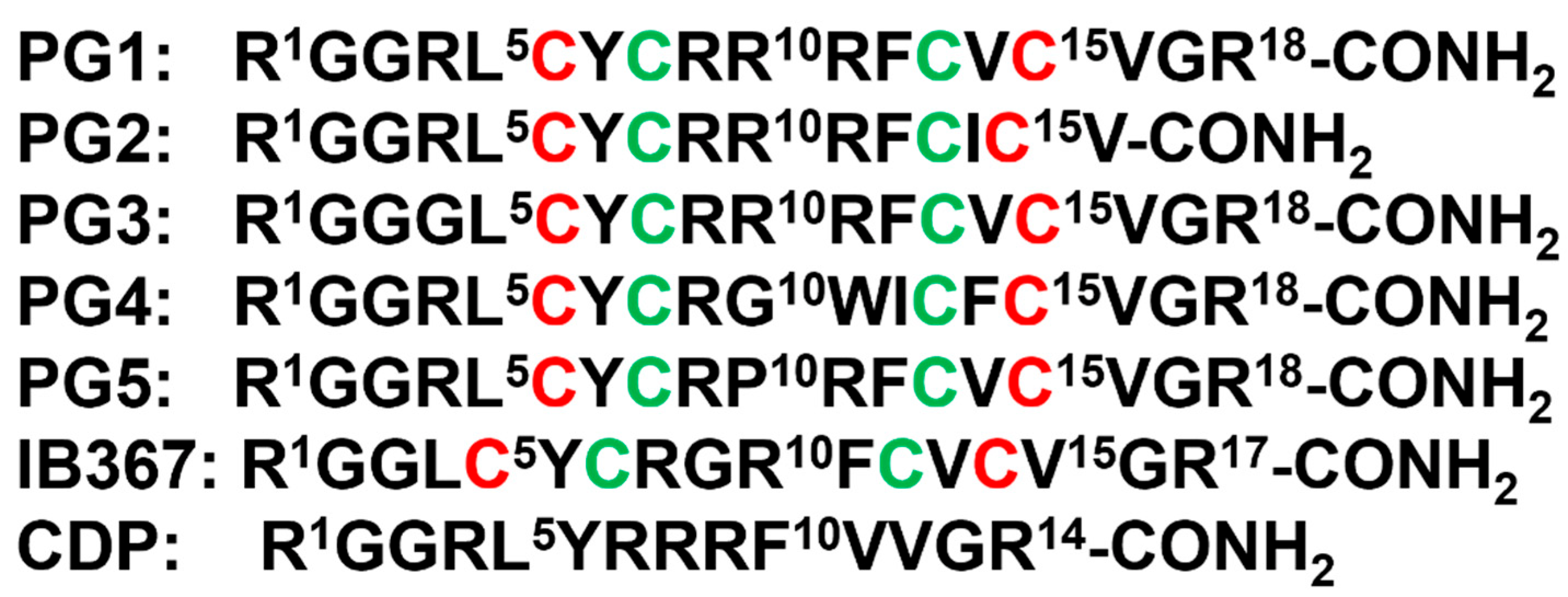
Figure 2. Amino acid sequence of protegrins and its derivatives. The disulfide bonds are formed between Cys6-Cys15 and Cys8-Cys13 residues, depicted by red and green color, respectively.
4. Outer Membrane Protein Targeting Antibiotics (OMPTA)
OMPTA defines a new class of peptides or peptide mimetics which may be highly effective in killing MDR Gram-negative bacteria
[69][70][71][93,94,95]. As a mode of action, OPMTA binds both to LPS and outer membrane proteins resulting in specific Gram-negative activity. In one of these endeavors, protegin-1 was utilized as a starting template generating series of peptidomimetics from designed libraries. In particular, 14-residue long backbone cyclized β-hairpin peptides were developed with inclusion of conserved L-Pro1 and D-Pro14 dipeptide motif
[72][96]. Active peptides were screened from these libraries which specifically inhibited only strains of
P. aeruginosa with MIC value as low as 0.008 μg/mL
[72][96]. One of the candidate peptides termed murepavadin was tested in clinical trials for potential treatment for pneumonia
[73][97]. Murepavadin binds to outer-membrane β-barrel protein LptD one of the components of LPS transport machinery
[72][96]. However, the exact site of binding of murepavadin or any other related peptides to LptD is not known. As such, LptD has a large, conserved C-terminal domain embedded in LPS outer membrane and a relatively short variable N-terminal at the periplasmic domain
[74][98]. The specific
P. aeruginosa killing activity of murepavadin over other Gram-negative bacteria may be conferred by binding with the periplasmic domain of LptD. Recently, the phase II clinical trial of murepavadin was halted due to occurrence of acute kidney disease and further preclinical development of the peptide has been undertaken
[75][99].
Darobactin, a seven residue (WNWSKSF) peptide, was first isolated from nematode symbiont bacteria
Photorhabdus khanii HGB1456. Non-ribosomally synthesized darobactin contains usual sidechain-sidechain covalent crosslinking between indole ring of W1 and β-carbon of W3, β-carbon of K5 and indole ring of W3
[76][100]. Darobactin can kill several Gram-negative bacteria strains in infection animal model but was not effective to tested Gram-positive bacteria. As a mode of action, darobactin directly binds to the outer-membrane protein BamA which is the central component of BamABCDE complex. Binding of darobactin to BamA inhibited chaperon function of the outer membrane protein causing bacterial cell death. BamA/darobactin complex revealed β-sheet like binding of the peptide with the β1-strand of the β-barrel structure of BamA
[76][77][100,101].
5. MSI Peptides
The first AMP isolated from the anuran family is magainin which was discovered from the skin of a female African clawed frog (
Xenopus laevis) by Michael Zasloff in 1987
[78][79][102,103]. Members of the magainin family (magainin-1, magainin-2, and PGLa) are cationic peptides which do not assume any preformed secondary structure in free solution but adopt amphipathic α-helical conformations in membranous environments
[80][81][104,105]. Magainins are non-hemolytic and non-cytotoxic host defense peptides that however display potent activity against broad range of bacteria, fungi, and protozoa
[14][79][14,103]. Magainin family is made up of two closely similar peptides (magainin-1 and magainin-2), each of which has 23 amino acids and differs by two substitutions at 10th and 22nd position of its primary structure (
Figure 35A)
[78][79][102,103]. It was also found that magainin-2 has comparatively higher activity than magainin-1. Following this discovery, numerous works were done using magainin-2 and its derivatives to understand activity, structure, and mechanism of action. Through extensive SAR analysis, Zasloff and colleagues at Magainin Pharmaceuticals (now Genaera Corporation) synthesized a 22-residue-long cationic MSI-78 peptide that demonstrated higher potency and greater selectivity to microbial cells compared to human red blood cells
[80][82][104,106]. MSI-78 exhibited a broad spectrum of potent antimicrobial activities against both Gram-positive and Gram-negative bacteria including the pathogens associated with the diabetic foot infections (DFI)
[82][106]. The MIC
50 and MIC
90 against all organisms tested from DFI were 16 and 32 μg/mL, respectively
[83][107]. Although MSI-78 peptide (known as pexiganan or Locilex) showed promising outcomes against several in vitro, in vivo, and pre-clinical studies, the Food and Drug Administration (FDA) of USA finally denied the new drug application (NDA) for its topical administration as a result of a deficit over a traditional antibacterial medication
[84][108]. In 2014, Dipexium Pharmaceuticals initiated another phase III clinical trial using pexiganan and ofloxacin (an FDA approved fluoroquinolone antibiotic) in a comparative clinical study for the same use. However, the trial reported a failure in 2017 since the peptide showed unsatisfactory results at sub-inhibitory concentrations against the tested organisms
[85][109]. It is noteworthy to mention that the Locilex (pexiganan topical cream 0.8%) received the advisory from European Medicines Agency (EMA) in 2015 for human and clinical use against DFI.
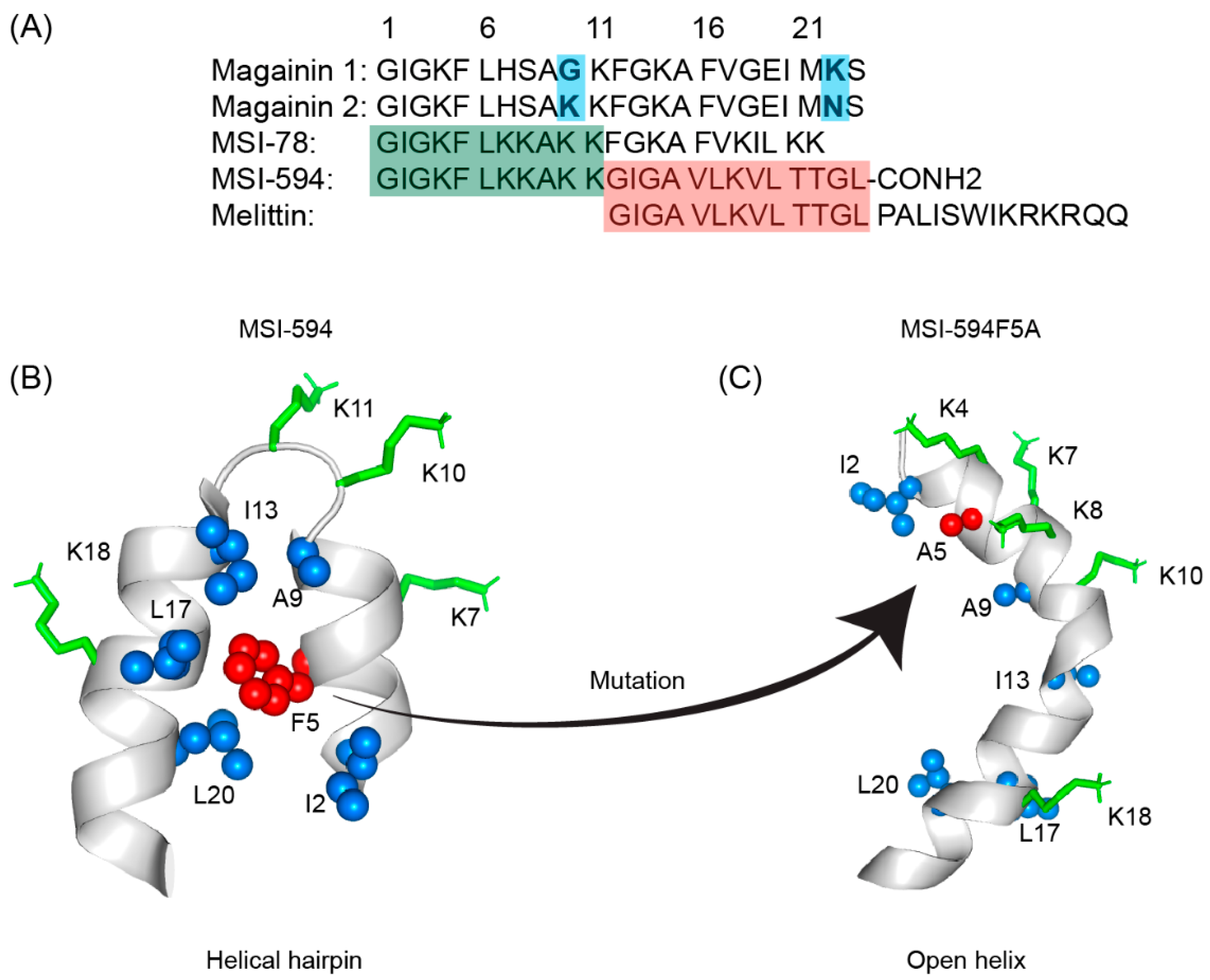
Figure 35. (
A) Amino acid sequences of magainin 1, magainin 2, MSI-78, MSI-594, and melittin. The sequence of MSI-594 was derived from MSI-78 and melittin, as indicated by the green and maroon boxes, respectively. (
B) MSI-594 adopted helical hairpin or helix-loop-helix structure in presence of LPS, while (
C) mutation of Phe5Ala converted the helical hairpin structure to open helix conformation in the same environment
[86][87][121,122].
6. LL-37
LL-37 is a cationic α-helical host defense peptide of human that belongs to cathelicidin antimicrobial peptide (CAMP) family
[88][89][126,127]. LL-37 is produced by many types of epithelial cells, as well as leukocytes such as monocytes, T cells, B cells, and NK cells and mostly stored in the lysosomes of macrophages and polymorphonuclear leukocytes (PMNs)
[89][127]. All cathelicidin AMPs are synthesized as preproproteins (18 KDa) containing a highly conserved N-terminal domain (13.5 KDa) and a vastly diverse antimicrobial domain at the C-terminus (4.5 KDa)
[89][127]. The N-terminal domain is typically 94–114 amino acids long and shares sequence homology with cathelin, a cysteine protease inhibitor identified in swine neutrophils. The C-terminus of human cathelicidin comprises 37-residue long LL-37 peptide (
Figure 46A)
[90][128]. The mature, functional form of the peptide is released after proteolytic cleavage of the signal and cathelin domains
[90][91][128,129]. In 1995, three scientific groups discovered the LL-37 peptide based on the study of highly conserved “cathelin” domain
[89][92][127,130]. Beside the cell proliferation, immunomodulation, and other signaling roles, the cationic LL-37 peptide (LLGDFFRKSKEKIGKEFKRIVQRIKDFLRNLVPRTES) shows excellent activity against a broad range of Gram-positive and Gram-negative pathogens
[93][94][131,132]. Since the LL-37 peptide possess several promising therapeutic potentials including the induction of angiogenesis, the ProMore Pharma in Poland completed the phase IIb clinical trial of LL-37 for treatment of venous leg ulcers
[94][95][132,133].
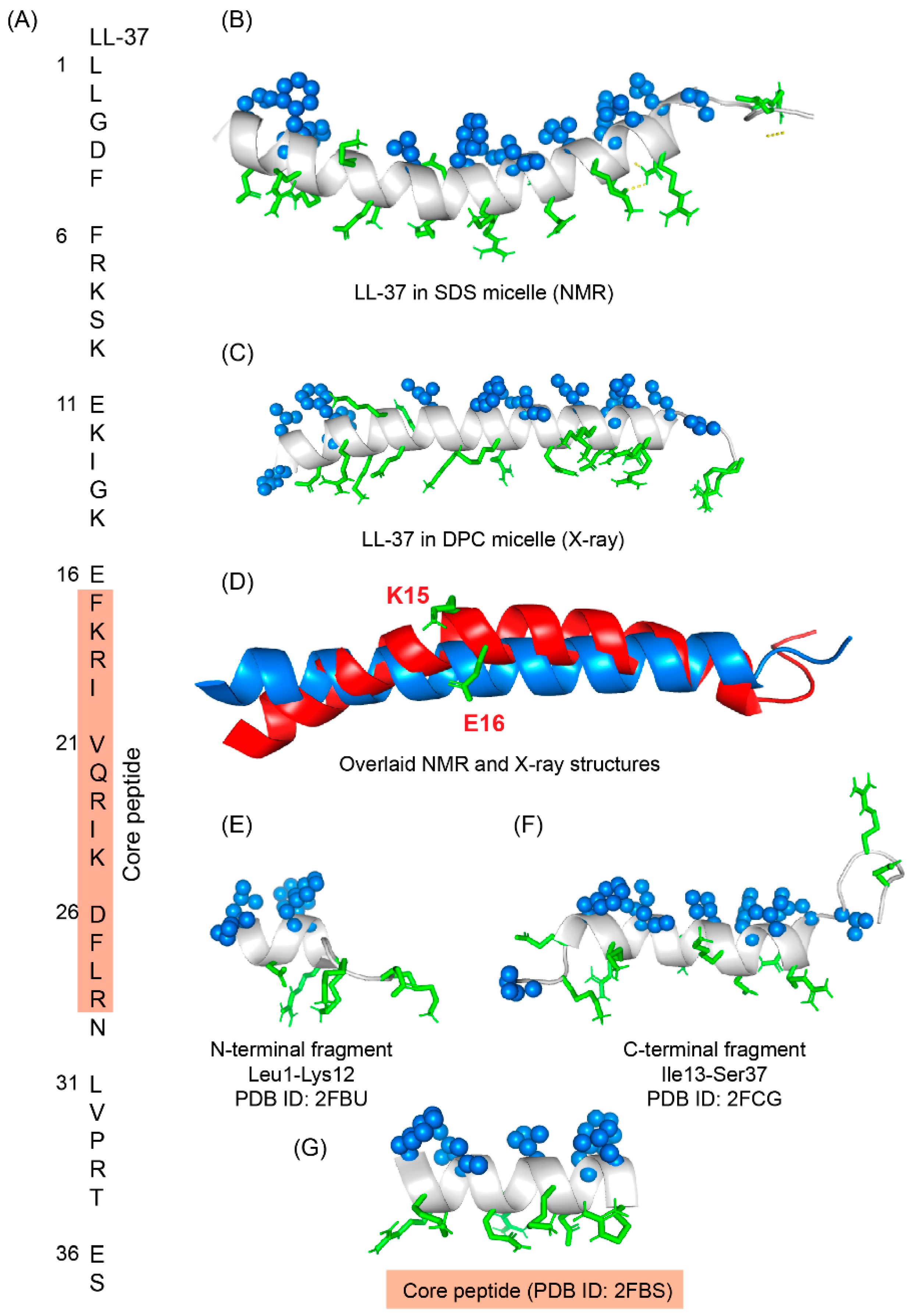
Figure 46. (
A) Amino acid sequence of LL-37 peptide; core peptide is highlighted by maroon-colored box. (
B) LL37 adopted a twisted amphipathic α-helical conformation in presence of SDS micelle (PDB: 2K6O)
[96][134]. (
C) In presence of DPC micelle, the peptide obtained a straight helical conformation as seen in the X-ray crystallographic analysis (PDB: 5NMN)
[97][135]. (
D) The overlaid pdb of NMR and crystallographic structures showed that the SDS bound LL-37 has a kink at residues Lys15 and Glu16. (
E–
G) The N-terminal, C-terminal, and core peptide fragments also obtained an amphipathic conformation in presence of SDS micelle
[98][136].