1. Introduction
- Introduction
Acute ocular burns can cause severe damage to the ocular surface ensuing in reduced visual function. One such manifestation is the ischemia of the limbal and perilimbal vasculature leading to damage to the limbal epithelial stems cells and to the surrounding niche that supports them. This can result in limbal stem cell deficiency (LSCD) which can cause significant visual morbidity [1]. Hence, accurate detection of this entity in the acute phase is essential to institute appropriate therapy. However, the presence of ischemia is often made based on subjective clinical signs and precise estimation of the same in an inflamed eye is challenging [2]. The use of the angiography feature of the optical coherence tomography (AS-OCTA) can help overcome this disadvantage by providing an objective tool to confirm the presence of ischemia [3]. Additionally, an AS-OCTA can also help quantify the changes in the vasculature.
- Principle of Optical Coherence Tomography Angiography (OCTA)
AS-OCTA generates images by obtaining serial b scans and then isolating the dynamic signals with the use of decorrelation algorithms. These signals result from the movement of red blood cells within vessels and by separating them form the surrounding static signals, the image of the vasculature is obtained. OCTA devices use different algorithms which include the full spectrum or split spectrum amplitude decorrelation angiography (FS or SSADA), optical microangiography (OMAG), intensity ratio analysis (OCTA-RA), etc. [4]. The present OCTA machines are Fourier-domain based and consist of the spectral domain {AngioVue (Optovue, Inc., Fremont, CA, USA), Angioscan (Nidek Co., Ltd., Gamagori, Aichi, Japan)} and swept-source OCTA systems {Triton DRI-OCT (Topcon Corporation, Tokyo, Japan), PLEX Elite Prototype 9000 (Carl Zeiss Meditec, Dublin, CA, USA)}. The AngioVue system has the highest resolution along with a rapid acquisition speed of 3–4 s per scan [5]. Thus, this device has the least amount of motion artifacts. The images from AS-OCTA yield results comparable to conventional dye based angiography [6–9].Furthermore, the inter-device values also correlate with each other [10].
- Comparison with conventional angiography
Ocular burns can present with a myriad of clinical manifestations in the acute phase. The grade of injury and the extent of ocular structures involved often determines the type and severity of the chronic sequelae that ensue. Limbal stem cell deficiency (LSCD) is one such chronic feature that can cause significant visual morbidity due to the corneal scarring and vascularization that occurs in its end stages [1]. The development of this pathology is usually heralded by the presence of limbal ischemia in the acute phase of the disease [2]. The detection and grading of this finding can help identify those individuals who are at a higher risk of developing LSCD. This cohort of patients can then be subjected to regular monitoring which will facilitate early identification and treatment of LSCD to prevent its complications and restore visual function. AS-OCTA does not require a dye for obtaining images and thus this circumvents the disadvantages and side effects associated with the commonly used dyes such as fluorescein and indocyanine green [11,12]. These include injection associated discomfort, nausea, vomiting, anaphylaxis, etc. [13,14]. Also, by avoiding the use of a dye, procuring images from AS-OCTA is significantly faster and the procedure can be repeated if required. Leakage form inflamed vessels may obscure ischemia in dye-based angiography and this drawback is not present in AS-OCTA [15].Since OCTA uses infrared light, vessels beneath hemorrhages can be visualized (Figure 1) [16,17].
However, the diagnosis of ischemia in the acute phase is based on subjective clinical findings which are often difficult to discern in an inflamed eye [2]. Thus, the adoption of diagnostic tools can provide an objective method of confirming ischemia of the limbal vasculature and assessing its progression. An anterior segment optical coherence tomography (AS-OCT) is usually available in most anterior segment practices and provides rapid, high-definition images of sections of the cornea with relative ease [3]. The utility of its angiography feature (AS-OCTA) has recently been explored to detect ischemia in acute burns as it can provide a real time in-vivo image of the limbal and perilimbal vasculature [4][5]. The use of image processing software has also aided in objectively quantifying the degree of vascular changes observed [6]. 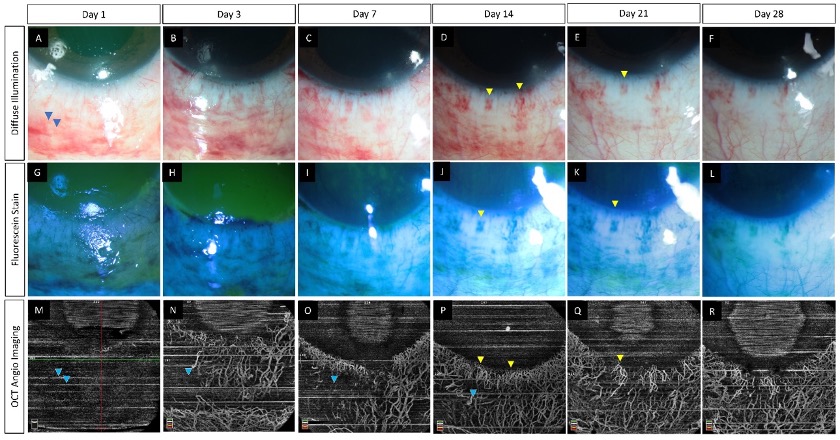
2. Principle and Technology of Optical Coherence Tomography Angiography (OCTA)
2.1. Principle
Optical coherence tomography (OCT) measures the signal of backscattered low coherence light incident on the area of interest and uses it to construct sectional and en-face images of different structures of the eye [7][8]. The angiography images are created by obtaining multiple b-scans of the same section over a period of time and assessing the difference in the signal of each scan [7]. This difference is attributed to the movement of erythrocytes within the blood vessels, and by removing the signal from the static surrounding structures, an angiography image is produced by isolating the temporal changes in these dynamic signals [9][10]. Several algorithms are employed to facilitate the same which include the full spectrum or split spectrum amplitude decorrelation angiography (FS or SSADA), optical microangiography (OMAG), intensity ratio analysis (OCTA-RA), etc. They assess the change in the phase of the signal, its amplitude, or both [10].
In SSADA, smaller spectral bands of light are created from the full spectrum and individual analysis is carried out in each band and then averaged [11]. This contrasts with FSADA where the full spectrum is analyzed as a whole. Thus, the SSADA produces images that have a better ratio of signal to noise. However, this is at the expense of axial resolution and this disadvantage is overcome by the OMAG and OCTA-RA algorithms. The former uses both phase and amplitude differences for its images while the latter is independent of the decorrelation principle and assesses the maximum and minimum signal intensity measured between two frames [12][13][14].
2.2. Technology
Broadly, the OCT machines can be classified as time-domain and Fourier-domain machines [4]. The current OCTA machines are all Fourier-domain based and consist of the spectral domain {AngioVue (Optovue, Inc., Fremont, CA, USA), Angioscan (Nidek Co., Ltd., Gamagori, Aichi, Japan)} and swept-source OCTA systems {Triton DRI-OCT (Topcon Corporation, Tokyo, Japan), PLEX Elite Prototype 9000 (Carl Zeiss Meditec, Dublin, CA, USA)}. The swept-source OCTAs have a longer wavelength (1050 nm) which improves the depth of structures imaged [15]. This, in combination with a faster speed of scan acquisition (200,000 scans/s), helps improve the resolution and provides a wider field of view [4]. Additionally, the eye tracking software of PLEX Elite is unique to anterior segment devices and can help decrease the artifacts produced [4]. However, the images procured by the AngioVue system have the highest axial and lateral resolution of 5 and 15 μm, respectively [9]. Its speed of acquisition is also the highest at 3–4 s per scan because of which the motion artifacts generated with this device are the least. In comparison with dye-based angiography, both AngioVue and Angioscan machines were found to be comparable, yielding quantifying parameters that correlated well with each other [16][17][18][19]. Although the inter-device values correlate well with each other, the absolute values were found to be greater with AngioVue, implying that these inter-system values cannot be compared with each other [20]. AS-OCTA can not only confirm the presence or absence of vasculature but can also reliably and accurately detect changes in vessel caliber [6].
3. Comparison with Fluorescein Angiography (FA) and Indocyanine Green Angiography (ICG)
The most important factor that sets OCTA apart from its counterpart vessel-imaging modalities is its non-invasive nature as it is not dependent on the use of a dye [21][22]. This obviates the injection-associated trauma and dye-associated risks such as nausea, vomiting, and, rarely, anaphylaxis [23][24]. By avoiding the use of an external dye, the time taken for the angiography is significantly decreased with the use of OCTA which is especially desirable in patients with painful disorders such as acute burns. The test can be repeated multiple times without any need for recurrent dye injections. Additionally, the ischemic zones may be obscured by leakage of the dye from the inflamed vessels in these eyes and this disadvantage is circumvented with OCTA [25].
The use of light in the infrared wavelength by the OCTA provides an added advantage as the device can image vessels beneath hemorrhages from superficial conjunctival vessels [6][26]. This is of particular significance in eyes with acute burns as these traumatic sub-conjunctival hemorrhages (SCH) are common and may mask areas of ischemia when viewed via conventional angiographic techniques. However, shadow artifacts can also be seen in AS-OCTA images due to these hemorrhages. This contrast has been depicted in Figure 1 where in the inferior area the vasculature is obscured by the SCH. At the same time in the region abutting the inferior limbus, the vasculature is clear despite the presence of the SCH. One possible explanation for this discrepancy can be the relative position of the SCH when compared to the vessels. Very superficial and large hemorrhages may block the view of all the underlying vessels as opposed to deeper SCH which may allow the imaging of vessels overlying the hemorrhage.
. (A–R) Serial monitoring with slit lamp photographs (without and with fluorescein staining) along with anterior segment optical coherence tomography angiography (AS-OCTA) imaging in an eye with acute chemical burns. (A–L) These images show the progressive healing of the corneal and conjunctival epithelial defects in the inferior part of the eye which is complete by day 7. (M–R) AS-OCTA images show a blocked signal in the initial three visits due to the subconjunctival hemorrhages inferiorly (blue arrow heads) with clear delineation of the vasculature following the resolution of the hemorrhages. The hemorrhages abutting the limbus, however, have not affected the vascular signal (yellow arrow heads).
With the segmentation feature of the OCTA, the exact depth of a lesion can also be determined [6,18,19]. In comparison to traditional angiography, OCTA is limited by its relatively smaller field of view and by its inability to assess leakage and differentiate between low flow areas and ischemic regions [3,14,20,21]. Since OCTA images are dependent on relative change in position, the movement of the patients’ head or eyes can result in artifacts.
4. Normal Limbal Vasculature on OCTA
4.1. Clinical Anatomy
The anterior roots of the episcleral arterial circle derived from the anterior ciliary arteries supply the limbus [22]. The arterioles from this plexus form capillary bed which drains into a venous plexus. The venules from this plexus become tributaries of episcleral collecting veins which coalesce with the emissary's veins [22,23].
4.2. OCTA of Normal Limbus
Since OCT machines typically image the retina and the choroid, special adaptor lenses are needed for anterior segment angiography [21,24]. Proper patient positioning and cooperation are required to ensure good quality images with minimal artifacts. By improving patient comfort, topical anesthetic agents may facilitate the same. Images are captured from all quadrants by scanning in different gaze positions of the eye [16]. Normal limbal vessels are seen as hairpin loops arcade extending up to 2 mm into the cornea (Figure 2). Segmentation studies have revealed radial superficial vessels with a Y-shaped flow pattern in the deeper layers [18].
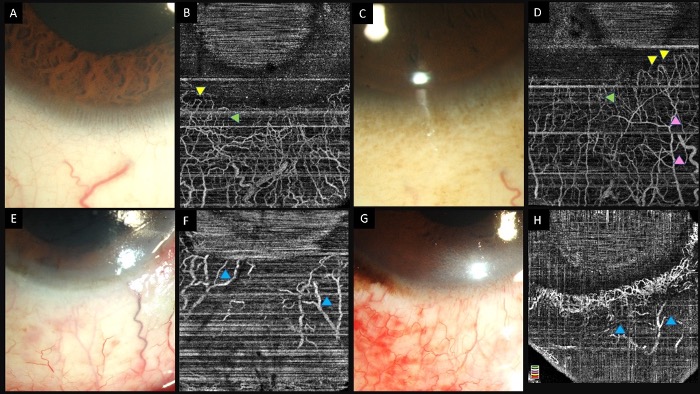
Figure 2. (A–D) Collage of images of the slit lamp and anterior segment optical coherence tomography angiography images of eyes with normal limbal and perilimbal vasculature. Hairpin loop vessels are noted at the corneal end of the limbus (yellow arrowheads). A combination of both Y-shaped (pink arrowheads) and radial vessels (green arrowheads) are noted in the angiography images. (E–H) Slit lamp and OCTA images in eyes with chemical injury illustrating ischemic zones in both angiographies with distortion of the limbal vasculature (blue arrowheads).
4.3. Qualitative Assessment of Limbal Vasculature
The presence of ischemia on AS-OCTA is assessed based on the areas which show no vascular signal. A qualitative assessment obviates the need for post-acquisition processing and is perhaps preferred for routine clinical practice. Both vasospasm and ischemia may manifest as lack of notable vasculature, however on serial monitoring the two can be differentiated. As the vasospasm resolves, the vascular signal reverts to normal on the subsequent scans (Figure 3).
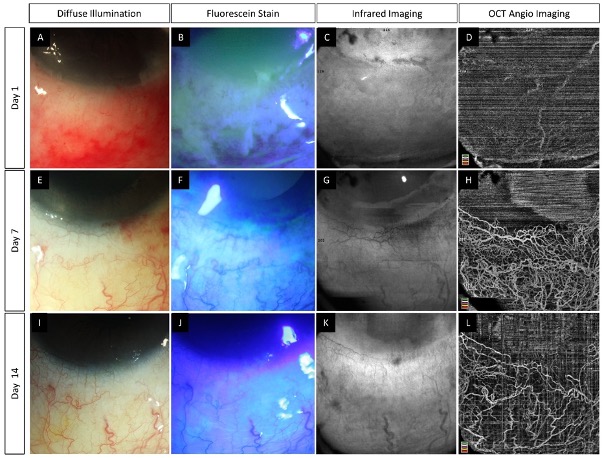
(A–L) Slit lamp photographs taken without and with fluorescein staining along with infrared and anterior segment optical coherence tomography angiography (AS-OCTA) images depicting the course of healing in an eye with acute chemical burns. An inferior corneal and conjunctival epithelial defect is noted (A,B) at presentation which heals over the course of two weeks (E,F,I,J). (D) AS-OCTA image taken at presentation shows a lack of vessels in the inferior half of the eye. (H,L) A progressive increase is noted in the number of visible vessels suggesting the presence of vasospasm in the initial images which has reversed in the subsequent visits.
4.4 Quantitative Assessment of Limbal Vasculature
External image processing software such as Fiji (National Institutes of Health, Bethesda, MD, USA) and MATLAB (MathWorks, Inc., Natick, MA, USA) software are employed to quantitatively assess ischemia [10,16,25]. Within the software, the area to be studied is converted into a greyscale image and the artifacts present are removed manually or by signal thresholding [10,16,26]. A binary image is then generated which can be skeletonized by using single pixel width to represent the vessel diameter [27]. The different indices used to assess vasculature in AS-OCTA have been presented in table 1. Of these, vessel density is most commonly used in the context of ocular burns. The extent of limbal disruption occurring following an acute burn injury can be assessed directly and graded based on the severity of damage [28].
Table 1.
Summary of various vessel-related parameters used for quantifying limbal vasculature and their reported ranges.
Indices
|
Method of Calculation [29,30]
|
Vessel density (%)
|
Area occupied by the vessels/ Total area of the background
|
Vessel length density (%)
|
Skeletonized vessel pixel area/ Total pixel count
|
Vessel diameter index
|
Total vessel area in binarized image/ Total vessel area in skeletonized image
|
Fractal dimension
|
Box counting method
|
5. Need for OCTA in Acute Ocular Burns
The inflamed ocular structures in acute burns may preclude accurate assessment of ischemia [2]. The need for surgical intervention such as tenonplasty is based on the degree of ischemia in the acute phase. The site of advancement of the Tenon’s layer also requires prior knowledge of the exact extent of the ischemia. AS-OCTA can facilitate this decision making by identifying the patients who truly require the procedure and by localizing the area of non-perfusion. Also, the development of LSCD occurs in the area of the ischemia and AS-OCTA can be used to estimate the probable severity of this sequelae (Figure 4).
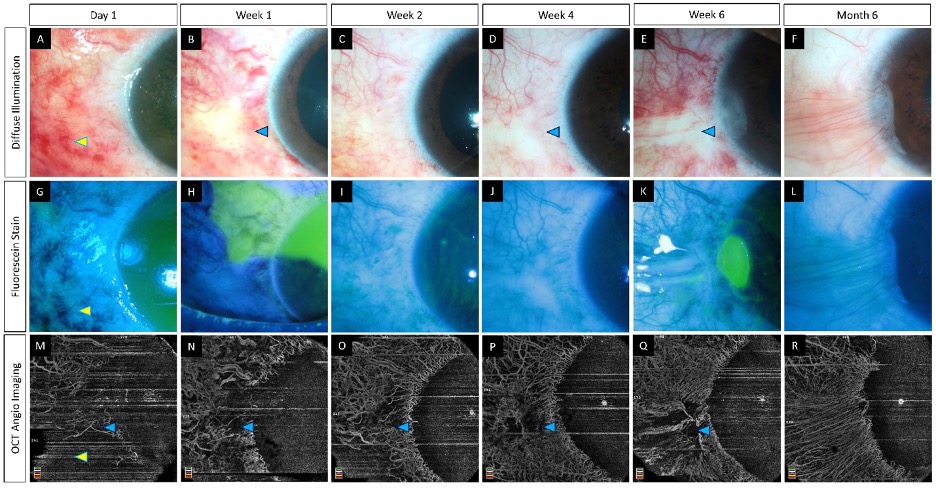
Figure 4. (
–
R) Serial monitoring with slit lamp photographs (without and with fluorescein staining) along with anterior segment optical coherence tomography angiography (AS-OCTA) imaging in an eye with acute chemical burns. (
) Serial imaging with slit lamp photographs (without and with fluorescein staining) and optical coherence tomography angiography imaging in an eye with acute chemical burns. (
–
L) These images show the progressive healing of the corneal and conjunctival epithelial defects in the inferior part of the eye which is complete by day 7. (
) These images depict a total corneal epithelial defect with an adjacent conjunctival defect at presentation, which decreased in size and ultimately healed after 2 weeks. (
–R) The corresponding angiography images illustrate an initial masked signal due to the sub-conjunctival hemorrhages (yellow arrowheads) which recovered with the resolution of the hemorrhages. Persistent ischemia is noted in the limbal area abutting the cornea (blue arrowheads) with epithelial healing issues in the adjacent cornea at the 6th week visit. A partial LSCD is noted in the same area at the 6th month follow-up (F).
5.1. Animal Studies
In a rabbit model of chemical injury, a significant decrease was observed in the vessel density within 24 hours of the injury [31]. This indicates that AS-OCTA has sufficient sensitivity to detect acute phase changes. Additionally, the change in vessel density correlated with the severity of the injury. A classification system was devised by the authors which incorporated ischemia as assessed by AS-OCTA. In a mice model, both OCTA and FA were used to study the limbal and corneal vasculature in acute burns [32]. Both devices yielded comparable results and nascent limbal vessels were detected within 4 days of the injury.
5.2. Human Studies
Fung et al. reported an underestimation of the extent of ischemia on clinical examination when compared to that estimated by OCTA [17]. They also found that the extent of ischemia was lesser on AS-OCTA when compared to the size of the conjunctival epithelial defect. A differential ischemia was depicted in the superficial conjunctival and the deeper intrascleral vessels [17]. A good inter-grader agreement has been described for the assessment of ischemia on AS-OCTA while the same was not true for clinical examination [28]. A strong positive correlation has been demonstrated between the extent of limbal ischemia and visual acuity at 3 months [17]. While the location of the LSCD has been found to correspond with the ischemic area on OCTA, the same correlation with limbal staining is absent [17,28].
6. Future Directions
The potential of AS-OCTA in the assessment of ischemia in acute burns is indubitable however, the actual technology is still nascent. Current AS-OCTA devices employ retinal software algorithms and customizing these for anterior segment imaging will go a long way in improving the output from the images. Software that can facilitate features such as eye tracking and the differentiation between low flow areas and ischemia will help build a standardized platform for the assessment of ischemia in ocular burns. Incorporation of this assessment into existing classification systems will help improve the prognosticating system for acute ocular burns.
7. Summary
Limbal ischemia is a serious consequence of severe ocular burns and can result in debilitating sequelae. Identification of this finding based on clinical examination alone in the acute phase is unreliable and thus, tools such as AS-OCTA provide an invaluable avenue for its objective assessment and quantification. The device is not dependent on the use of a dye and provides high-definition images with ease. Vessel density is the most commonly used index for the quantification of ischemia and provides values with correlate with the severity of damage. Further studies can help establish diagnostic and quantifying AS-OCTA derived parameters for the management of acute ocular burns.
R) AS-OCTA images show a blocked signal in the initial three visits due to the subconjunctival hemorrhages inferiorly (blue arrow heads) with clear delineation of the vasculature following the resolution of the hemorrhages. The hemorrhages abutting the limbus, however, have not affected the vascular signal (yellow arrow heads).
The infrared wavelength of light also allows for better patient comfort and has a good safety profile as well [27]. Localization of the exact depth of a vascular lesion is now feasible because OCTA allows segmentation of different layers of the imaged vessels [16][28][29]. This is in contrast to FA and ICG which generate a composite two-dimensional image. The major drawback of OCTA when compared to the traditional angiography tools lies in its inability to identify the flow characteristics and thus, patterns of leakage, the difference between afferent and efferent systems, etc. cannot be captured with OCTA [30][31]. In the context of ocular burns, this is relevant as images from OCTA may not distinguish vasospasm from true ischemia and thus caution must be exercised during interpretation of OCTA scans. Furthermore, the field of view is larger in FA and ICG and multiple scans would be required by OCTA to cover the same surface area [3][24]. As OCTA images are derived from the motion of blood cells, this process can be confounded by motion originating from other sources, such as movements of the patient’s eyes or head. Although the eye tracking feature of retinal OCTA can counteract these artifacts, its incorporation in anterior segment images has not been completely established.
eferences
- Haagdorens, M.; Van Acker, S.I.; Van Gerwen, V.; Ní Dhubhghaill, S.; Koppen, C.; Tassignon, M.-J.; Zakaria, N. Limbal Stem Cell Deficiency: Current Treatment Options and Emerging Therapies. Stem Cells Int 2016, 2016, 9798374, doi:10.1155/2016/9798374.
- Kam, K.W.; Patel, C.N.; Nikpoor, N.; Yu, M.; Basu, S. Limbal Ischemia: Reliability of Clinical Assessment and Implications in the Management of Ocular Burns. Indian J Ophthalmol 2019, 67, 32–36, doi:10.4103/ijo.IJO_945_18.
- Ayres, M.; Smallwood, R.; Brooks, A.M.; Chan, E.; Fagan, X. Anterior Segment Optical Coherence Tomography Angiography. J Vis Commun Med 2019, 42, 153–157, doi:10.1080/17453054.2019.1631122.
- Lee, W.D.; Devarajan, K.; Chua, J.; Schmetterer, L.; Mehta, J.S.; Ang, M. Optical Coherence Tomography Angiography for the Anterior Segment. Eye and Vision 2019, 6, 4, doi:10.1186/s40662-019-0129-2.
- Munk, M.R.; Giannakaki-Zimmermann, H.; Berger, L.; Huf, W.; Ebneter, A.; Wolf, S.; Zinkernagel, M.S. OCT-Angiography: A Qualitative and Quantitative Comparison of 4 OCT-A Devices. PLoS One 2017, 12, e0177059, doi:10.1371/journal.pone.0177059.
- Devarajan, K.; Di Lee, W.; Ong, H.S.; Lwin, N.C.; Chua, J.; Schmetterer, L.; Mehta, J.S.; Ang, M. Vessel Density and En-Face Segmentation of Optical Coherence Tomography Angiography to Analyse Corneal Vascularisation in an Animal Model. Eye and Vision 2019, 6, 2, doi:10.1186/s40662-018-0128-8.
- Ang, M.; Cai, Y.; MacPhee, B.; Sim, D.A.; Keane, P.A.; Sng, C.C.A.; Egan, C.A.; Tufail, A.; Larkin, D.F.; Wilkins, M.R. Optical Coherence Tomography Angiography and Indocyanine Green Angiography for Corneal Vascularisation. British Journal of Ophthalmology 2016, 100, 1557–1563, doi:10.1136/bjophthalmol-2015-307706.
- Hong, J.; Zhu, W.; Zhuang, H.; Xu, J.; Sun, X.; Le, Q.; Li, G.; Wang, Y. In Vivo Confocal Microscopy of Conjunctival Goblet Cells in Patients with Sjogren’s Syndrome Dry Eye. Br J Ophthalmol 2010, 94, 1454–1458, doi:10.1136/bjo.2009.161059.
- Stanzel, T.P.; Devarajan, K.; Lwin, N.C.; Yam, G.H.; Schmetterer, L.; Mehta, J.S.; Ang, M. Comparison of Optical Coherence Tomography Angiography to Indocyanine Green Angiography and Slit Lamp Photography for Corneal Vascularization in an Animal Model. Sci Rep 2018, 8, 11493, doi:10.1038/s41598-018-29752-5.
- Ang, M.; Devarajan, K.; Das, S.; Stanzel, T.; Tan, A.; Girard, M.; Schmetterer, L.; Mehta, J.S. Comparison of Anterior Segment Optical Coherence Tomography Angiography Systems for Corneal Vascularisation. Br J Ophthalmol2018, 102, 873–877, doi:10.1136/bjophthalmol-2017-311072.
- Kuckelkorn, R.; Remky, A.; Wolf, S.; Reim, M.; Redbrake, C. Video Fluorescein Angiography of the Anterior Eye Segment in Severe Eye Burns. Acta Ophthalmol Scand 1997, 75, 675–680, doi:10.1111/j.1600-0420.1997.tb00629.x.
- Cai, Y.; Alio Del Barrio, J.L.; Wilkins, M.R.; Ang, M. Serial Optical Coherence Tomography Angiography for Corneal Vascularization. Graefes Arch Clin Exp Ophthalmol 2017, 255, 135–139, doi:10.1007/s00417-016-3505-9.
- Xu, K.; Tzankova, V.; Li, C.; Sharma, S. Intravenous Fluorescein Angiography-Associated Adverse Reactions. Can J Ophthalmol 2016, 51, 321–325, doi:10.1016/j.jcjo.2016.03.015.
- Anijeet, D.R.; Zheng, Y.; Tey, A.; Hodson, M.; Sueke, H.; Kaye, S.B. Imaging and Evaluation of Corneal Vascularization Using Fluorescein and Indocyanine Green Angiography. Investigative Ophthalmology & Visual Science 2012, 53, 650–658, doi:10.1167/iovs.11-8014.
- Ang, M.; Tan, A.C.S.; Cheung, C.M.G.; Keane, P.A.; Dolz-Marco, R.; Sng, C.C.A.; Schmetterer, L. Optical Coherence Tomography Angiography: A Review of Current and Future Clinical Applications. Graefes Arch Clin Exp Ophthalmol 2018, 256, 237–245, doi:10.1007/s00417-017-3896-2.
- Patel, C.N.; Antony, A.K.; Kommula, H.; Shah, S.; Singh, V.; Basu, S. Optical Coherence Tomography Angiography of Perilimbal Vasculature: Validation of a Standardised Imaging Algorithm. Br J Ophthalmol 2020, 104, 404–409, doi:10.1136/bjophthalmol-2019-314030.
- Fung, S.S.M.; Stewart, R.M.K.; Dhallu, S.K.; Sim, D.A.; Keane, P.A.; Wilkins, M.R.; Tuft, S.J. Anterior Segment Optical Coherence Tomographic Angiography Assessment of Acute Chemical Injury. Am J Ophthalmol 2019, 205, 165–174, doi:10.1016/j.ajo.2019.04.021.
- Akagi, T.; Uji, A.; Huang, A.S.; Weinreb, R.N.; Yamada, T.; Miyata, M.; Kameda, T.; Ikeda, H.O.; Tsujikawa, A. Conjunctival and Intrascleral Vasculatures Assessed Using Anterior Segment Optical Coherence Tomography Angiography in Normal Eyes. Am J Ophthalmol 2018, 196, 1–9, doi:10.1016/j.ajo.2018.08.009.
- Giarratano, Y.; Bianchi, E.; Gray, C.; Morris, A.; MacGillivray, T.; Dhillon, B.; Bernabeu, M.O. Automated Segmentation of Optical Coherence Tomography Angiography Images: Benchmark Data and Clinically Relevant Metrics. Transl Vis Sci Technol 2020, 9, 5, doi:10.1167/tvst.9.13.5.
- Siddiqui, Y.; Yin, J. Anterior Segment Applications of Optical Coherence Tomography Angiography. Semin Ophthalmol 2019, 34, 264–269, doi:10.1080/08820538.2019.1620805.
- Luo, M.; Li, Y.; Zhuo, Y. Advances and Current Clinical Applications of Anterior Segment Optical Coherence Tomography Angiography. Front Med (Lausanne) 2021, 8, 721442, doi:10.3389/fmed.2021.721442.
- Meyer, P.A. The Circulation of the Human Limbus. Eye (Lond) 1989, 3 ( Pt 2), 121–127, doi:10.1038/eye.1989.19.
- Wang, Y.; Chodosh, J. Angiography of the Limbus and Cornea. Int Ophthalmol Clin 2019, 59, 19–29, doi:10.1097/IIO.0000000000000283.
- Brunner, M.; Romano, V.; Steger, B.; Vinciguerra, R.; Lawman, S.; Williams, B.; Hicks, N.; Czanner, G.; Zheng, Y.; Willoughby, C.E.; et al. Imaging of Corneal Neovascularization: Optical Coherence Tomography Angiography and Fluorescence Angiography. Investigative Ophthalmology & Visual Science 2018, 59, 1263–1269, doi:10.1167/iovs.17-22035.
- Ang, M.; Baskaran, M.; Werkmeister, R.M.; Chua, J.; Schmidl, D.; Aranha dos Santos, V.; Garhöfer, G.; Mehta, J.S.; Schmetterer, L. Anterior Segment Optical Coherence Tomography. Progress in Retinal and Eye Research 2018, 66, 132–156, doi:10.1016/j.preteyeres.2018.04.002.
- Varma, S.; Shanbhag, S.S.; Donthineni, P.R.; Mishra, D.K.; Singh, V.; Basu, S. High-Resolution Optical Coherence Tomography Angiography Characteristics of Limbal Stem Cell Deficiency. Diagnostics (Basel) 2021, 11, 1130, doi:10.3390/diagnostics11061130.
- Durbin, M.K.; An, L.; Shemonski, N.D.; Soares, M.; Santos, T.; Lopes, M.; Neves, C.; Cunha-Vaz, J. Quantification of Retinal Microvascular Density in Optical Coherence Tomographic Angiography Images in Diabetic Retinopathy. JAMA Ophthalmol 2017, 135, 370–376, doi:10.1001/jamaophthalmol.2017.0080.
- Ang, M.; Foo, V.; Ke, M.; Tan, B.; Tong, L.; Schmetterer, L.; Mehta, J.S. Role of Anterior Segment Optical Coherence Tomography Angiography in Assessing Limbal Vasculature in Acute Chemical Injury of the Eye. British Journal of Ophthalmology 2021, doi:10.1136/bjophthalmol-2021-318847.
- Ang, M.; Cai, Y.; Tan, A.C.S. Swept Source Optical Coherence Tomography Angiography for Contact Lens-Related Corneal Vascularization. J Ophthalmol 2016, 2016, 9685297, doi:10.1155/2016/9685297.
- Stanga, P.E.; Tsamis, E.; Papayannis, A.; Stringa, F.; Cole, T.; Jalil, A. Swept-Source Optical Coherence Tomography AngioTM (Topcon Corp, Japan): Technology Review. Dev Ophthalmol 2016, 56, 13–17, doi:10.1159/000442771.
- Tey, K.Y.; Gan, J.; Foo, V.; Tan, B.; Ke, M.Y.; Schmetterer, L.; Mehta, J.S.; Ang, M. Role of Anterior Segment Optical Coherence Tomography Angiography in the Assessment of Acute Chemical Ocular Injury: A Pilot Animal Model Study. Sci Rep 2021, 11, 16625, doi:10.1038/s41598-021-96086-0.
- Luisi, J.; Kraft, E.R.; Giannos, S.A.; Patel, K.; Schmitz-Brown, M.E.; Reffatto, V.; Merkley, K.H.; Gupta, P.K. Longitudinal Assessment of Alkali Injury on Mouse Cornea Using Anterior Segment Optical Coherence Tomography. Transl Vis Sci Technol 2021, 10, 6, doi:10.1167/tvst.10.3.6.