The functionality exclusive to the surface of the pores enables the use of these arrays in technical applications, such as the site-specific immobilization of proteins, essential to avoid hampering the activity of the protein which is beneficial to the specific selective adsorption of protein [43,88][1][2]. The protein orientation plays a critical role if protein microarrays are to be used as quantitative tools in biomedical research or clinically important protein-based nanomedicines [89][3]. For example, the surfaces prepared could be of potential interest as 3D cell culture platforms, that have been otherwise obtained via tedious multi-step procedures using lithographic techniques. The cell adhesion and proliferation on HCP films can be controlled by polymer film surface chemistry, which can be directed into the pores by functionalizing the pore-interior with glycopolymers or proteins [48,90,91][4][5][6]. This can enhance their proliferation and retain spherical morphology. Moreover, the specific patterning of metal or semiconductor materials could be applied in photonics, since the photonic band structure depends on the spatial periodic structures and the refractive indices of the dielectric materials in the structure [92][7].
2.1. Pore-Selective Functionalization of HCP Films by Self-Assembly
2. Pore-Selective Functionalization of HCP Films by Self-Assembly
A great deal of previous research into pore-selective functionalization has focused on the self-assembly of block-co-polymers or amphiphilic materials, and Pickering emulsion of NPs
[1,2,32,46][8][9][10][11]. Traditionally, pore-selective functionalization of HCP films is performed using a simple physical phenomenon, i.e., self-assembly of functional groups which are usually hydrophilic
[93][12]. These involve physical phenomenon which was driven by the stabilization of interfacial tension at polymer solution/aqueous droplet interfaces
[94][13]. If the film-forming polymer is amphiphilic or the polymer has a hydrophilic functional group in the hydrophobic polymer chain, the hydrophilic functional group will orient in the direction of the top surface of the film, to interact with humidity
[81][14]. Therefore, the distribution probability of the hydrophilic group is higher at the top surface including the pore surface of the film while the hydrophobic polymer chain is highly distributed at the bottom surface of the film. The schematic representation of the self-assembly process demonstrating the possible mechanism is shown in
Figure 31.
Figure 31. A sequence of stages of pore-selective functionalization by self-assembly of amphiphilic polymer. (a) Polymer solution containing amphiphilic polymer in a volatile solvent under humid conditions; (b) condensation of water droplets due to cooling of surface temperature; (c) close packing of water droplets and self-assembly of hydrophilic part of the amphiphilic polymer around the water droplets; (d) formation of pore-functionalized HCP film after drying.
An innovative work was performed by Boker et al. by combining self-assembly and Pickering emulsions
[86][15]. They were able to functionalize tri-n-octylphosphine oxide (TOPO) modified cadmium selenide (CdSe) NPs inside the pores of the HCP film. TOPO was used to stabilize the CdSe NPs and self-assemble around the interface of the water droplet/polymer solution during the BF self-assembly process, as shown in
Figure 42. This method opens a simple route for the pore-selective immobilization of ligands or proteins having a wide variety of functional groups, curated for targeted applications in sensors, separation, or catalysis. Min et al. succeeded in the pore-selective functionalization of poly (acrylic acid) (PAA) in the PS HCP film by using a block copolymer of PS-b-PAA
[87][16]. The block copolymer self-assembled around the water droplet during the BF process and the carboxylic group arranged inside the pores due to its amphiphilic property. The carboxylic acid (–COOH) groups inside the pores bonded with the hydrazide group of the biotin derivative for the patterning of proteins inside the pores, as shown in
Figure 53. By the functionalization of PAA inside the pores, a range of other chemistries can be applied to pore-selectively immobilize proteins or other molecules of interest. For example, using this technique, Liang et al. successfully fabricated glucose-responsive HCP film used for self-regulated and controlled insulin release, as shown in
Figure 6 [95]4 [17]. The use of PAA to functionalize pores holds a lot of potential as multi-functional drugs, such as antimicrobial or anti-inflammatory molecules, could also be loaded onto the porous HCP film for direct drug delivery via skin
[95][17]. Rodríguez-Hernández and co-workers effectively prepared antibacterial and antifouling materials selectively functionalized at the pore cavity for selective cell culturing
[5,80][18][19]. They used blends of a diblock copolymer in a homopolymer matrix to precisely control the chemical functional groups inside the pores to obtain a selective surface for adhesion to mammalian cells while preventing bacterial contamination as shown in
Figure 75. These methods used reverse microemulsion technique
[95][17], or blends of a homopolymer (i.e., PS) and a block copolymer (polystyrene-b-poly (dimethylaminoethyl methacrylate)
[5][18] which demonstrated the effective functionalization of pores in HCP polymer films. However, the experimental conditions and concentration of amphiphilic materials or NPs should be carefully controlled for pore-specific functionalization. For instance, the use of block copolymers such as PS-b-PAA
[87][16], poly N-dodecylacrylamide-co-N-isopropylacrylamide)
[96][20] needs precise length control of the block monomers to control the surface wettability, and further functionalization with bioactive molecules
[87][16] or for NP deposition
[86][15].
Figure 42. (
a) Cross-sectional diagram of nanoparticle assembly at a water droplet–solution interface during the breath-figure formation. (
b) SEM images of the surface of an NP-decorated BF film. (
c) TEM images of cross-sections through porous PS film. Reprinted with permission from ref.
[86][15]. Copyright 2004 Nature Materials.
Figure 53. (
a) Synthetic approach to a streptavidin microarray with immobilization of protein selectively in the pores. (
b) Confocal microscopy images of PS-PAA honeycomb-structured porous films after modification with biotin and streptavidin. Reprinted with permission from ref.
[87][16]. Copyright 2008 Advanced Materials.
Figure 64. (
a) CLSM images of PBA-PAA/PS porous film after their immersion in ARS dye solution. (
b) SEM image of porous PS film loaded with insulin aggregates after immersion in 0.8 M NaCl aqueous solution at pH 5.3 at 25 °C. (
c) Scheme of the capture of glucose and the glucose-responsive release of insulin aggregates. Reprinted with permission from ref.
[95][17]. Copyright 2015 Journal of Materials Chemistry B.
Figure 75. (
a) Schematic representation of the chemical distribution of the blends of PS and PS-b-PPEGMA (75:25 wt% blend) at the surface. Reprinted with permission from ref.
[80][19]. Copyright 2016 ACS Applied Materials & Interfaces. (
b) Scheme of the strategy for selective cell culture. Mammalian cells interact only adhere to the top surface, while the bacteria may enter inside the pores due to size differences and get affected by antimicrobial functional groups. (
c) Cell adhesion tests using (actin staining (red), and Hoechst (blue)) after 96 h of culture on the fabricated film. Reprinted with permission from ref.
[5][18]. Copyright 2017 ACS Applied Materials & Interfaces.
2.3. Pore-Selective Functionalization of HCP Films Accompanying Chemical Reaction
3. Pore-Selective Functionalization of HCP Films Accompanying Chemical Reaction
A new BF process accompanying in situ interfacial chemical reactions has been introduced as an emerging field for the pore-selective functionalization of HCP films. When we examine the BF mechanism in more detail, one can observe the formation of an aqueous droplet/polymer solution interface during the pore formation process. This interface was used for pore functionalization by the process of interfacial chemical reactions. The interfacial chemical reactions are performed by taking the reactants in two or more different immiscible phases and allowed to react at the interface, the reaction potential along with the concentration gradient continuously drives the reactants toward the interface, thereby carrying the reaction to completion or high product yield at the interface. The most common interfaces used in interfacial reactions are two immiscible liquids or solid and liquid
[100][25]. In the BF process, an aqueous droplet/polymer solution interface can be found, as illustrated in
Figure 119, which can be employed to perform interfacial chemical reactions for pore-selective functionalization of HCP films, i.e., functionalization could be obtained only in the pores of HCP film. The modified BF method is briefly described as follows: (a) polymer solution containing reactant A in a volatile solvent under humid conditions, (b) condensation of water droplets containing reactant B at the polymer surface; after the close packing of water droplets and self-assembly of reactant A around the water droplets, (c) formation of product C at the polymer solution/water droplet interface due to the interfacial reaction between reactant A and reactant B, and finally after the evaporation of water droplets and drying of the film (d) pore-functionalized HCP film was obtained, as shown in
Figure 119.
Figure 119. A sequence of stages of pore-selective functionalization by self-assembly accompanied by chemical reaction. (a) polymer solution containing reactant A, (b) condensation of water droplets containing counter reactant B, (c) functionalizing of product C at the pore by the interfacial reaction of reactant A and reactant B, (d) formation of pore-functionalized HCP film after drying.
For convenience, the functionalization methods of HCP films by interfacial chemical reactions were divided into two types based on the humid conditions, i.e., water as a reactant and water containing another material as a reactant.
2.3.1. Pore-functionalization by BF process using water as a reactant
3.1. Pore-Functionalization by BF Process Using Water as a Reactant
For the method, a component reactive to water was added in the polymer solution such as titanium chloride
, [101[26][27],
102] or tin chloride
[28],
[103] and cast under humid conditions. This favored a chemical reaction to happen at the aqueous droplet/polymer solution interface and resulted in their respective products at the pores as shown in
Figure 120. Li et al. fabricated composite film with hemispherical or mushroom-like titanium oxide (TiO
2) microparticles lying in the pores of an HCP PS film which can be used with or without the polymer matrix
, as shown in
Figure 113. Similarly, Shin et al. reported the fabrication of PS HCP films with TiO
2 NPs filled pores with slight modification in the BF process
[29].
[104] Here, the micro-water droplets were used as a reactive template, and titanium butoxide (Ti(C
4H
9O)
4) was poured into the partially dried PS solution during the BF process. The (Ti(C
4H
9O)
4) reacts with micro-water droplets and forms TiO
2 NPs in the pores shown in
Figure 142. In most of these reports, the aqueous droplets were used as micro-reactors for the preparation of metal hydroxides/oxides with specific shapes but not for the functionalization of pores in HCP film, given in
Table 2.
LikFor example, using it as template for the fabrication of microdome selectively containing TiO
2 NPs
, which may have potential applications in different fields of electronic
optoelectronic
, and anti-reflective surfaces
[30].
[105] In another example, water droplets were used as polymerization initiators for the polymerization of acrylate monomer to obtain polyacrylate HCP film
[31].
[106] The aggregation of polymer around the water droplets triggered the polymerization of alkylcyanoacrylate, and the –OH heads of the formed chains were anchored on the water droplets and the growing chains persisted at the water–solution interface forming a pore-selective HCP film
, as shown in
Figure 153.
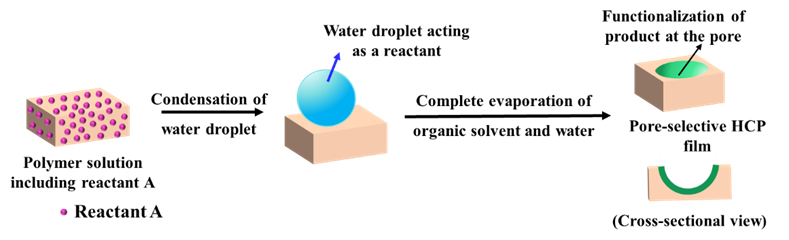
Figure 120. Pore-selective functionalization of HCP films by using water as a reactant. The polymer solution containing a reactant reactive to water is cast under humid conditions for the reaction to occur at the interface of polymer solution/water droplet to achieve pore-selective functionalization.
Table 2. Summary of reports using one reactant in polymer solution and water from the humid atmosphere as another reactant.
Reactant A in the Polymer solution
|
Humid conditions
|
Product/Functionalization in pore
|
Ref.
|
Titanium tetrachloride
|
Water
|
Titanium dioxide
|
[101]
|
Titanium tetrachloride
|
Water
|
Titanium dioxide
|
[102]
|
Tin tetrachloride
|
Water
|
Tin dioxide
|
[103]
|
Titanium butoxide
|
Water
|
Titanium dioxide NPs
|
[104]
|
Titanium n-butoxide
|
Water
|
Titanium dioxide
|
[107]
|
Titanium tetraisopropoxide
|
Water
|
Titanium dioxide
|
[108]
|
Alkylcyanoacrylate
|
Water
|
PACA
|
[106]
|
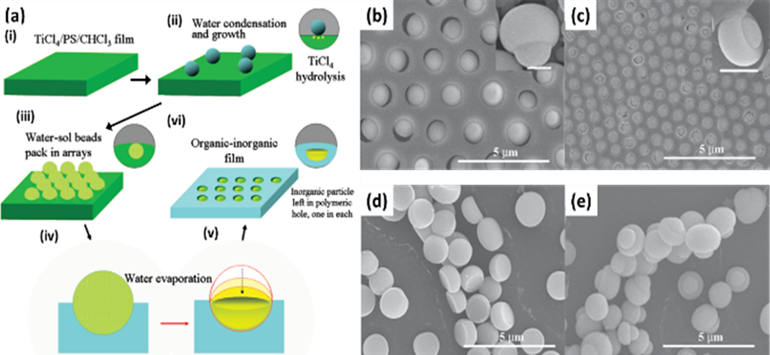
Pore-selective functionalization of HCP films by using water as a reactant. The polymer solution containing a reactant reactive to water is cast under humid conditions for the reaction to occur at the interface of polymer solution/water droplet to achieve pore-selective functionalization.
Figure 131.
a
) Scheme for the formation of highly ordered asymmetrical inorganic particle/polymer composite films by BFs method. (
b,
c
) SEM images of the obtained composite film from the TiCl
4/ PS/CHCl
3
solution with different concentrations of TiCl
4
c
d-
e
2 microparticles after calcination of the composite film at 450 °C for 3h. Reprinted with permission from ref [102]. Copyright 2011 Journal of the American Chemical Society.
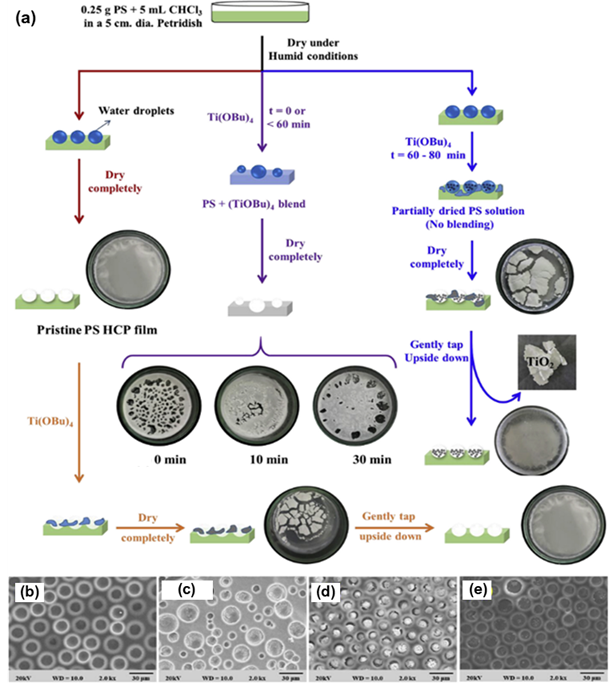
microparticles after calcination of the composite film at 450 °C for 3 h. Reprinted with permission from ref. [27]. Copyright 2011 Journal of the American Chemical Society.
Figure 142.
a) Schematic representation showing different reaction conditions with the variance in time intervals of pouring titanium butoxide solution to the PS solution. (b-e) shows the typical SEM images of PS film obtained at a different time interval. Reprinted with permission from ref [104]. Copyright 2018 Polymer.
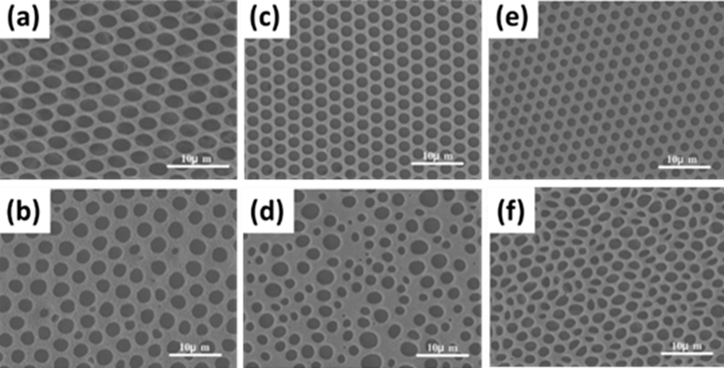
) Schematic representation showing different reaction conditions with the variance in time intervals of pouring (Ti(C4H9O)4) solution to the PS solution. (b–e) shows the typical SEM images of PS film obtained at a different time interval. Reprinted with permission from ref. [29]. Copyright 2018 Polymer.
Figure 153.
SEM images of porous PACA films fabricated from monomer solutions of (
a
c
e
) OCA, and polymer solutions of (
b
d) PBCA, (
) PBCA, (f) POCA. Reprinted with permission from ref. [31]. Copyright 2010 Journal of Colloid and Interface Science.
POCA. Reprinted with permission from ref [106]. Copyright 2010 Journal of Colloid and Interface Science.
2. Summary of reports using one reactant in polymer solution and water from the humid atmosphere as another reactant.
Reactant A in the Polymer Solution |
Humid Conditions |
Product/Functionalization in Pore |
Ref. |
Titanium tetrachloride |
Water |
Titanium dioxide |
[101] |
Titanium tetrachloride |
Water |
Titanium dioxide |
[102] |
Tin tetrachloride |
Water |
Tin dioxide |
[103] |
Titanium butoxide |
Water |
Titanium dioxide NPs |
[104] |
Titanium n-butoxide |
Water |
Titanium dioxide |
[107] |
Titanium tetraisopropoxide |
Water |
Titanium dioxide |
[108] |
Alkylcyanoacrylate |
Water |
PACA |
[106] |
2.3.2. BF process accompanying chemical reactions with a non-aqueous reactant
In the bottom-up process of the BF mechanism, the stage at which there is an interfacial interaction of water droplet and polymer solution can be applied for the site-selective functionalization. Applying this phenomenon, Huh et al. recently introduced a modified BF method, called the reactive BF (rBF) method, for the single-step fabrication of pore-selective functionalized films by accompanying an interfacial chemical reaction during the BF process. [98,109-113] For this method, a solvent-soluble reactant is added to the hydrophobic polymer solution, and a water-soluble counter reactant is added to the water used for humid conditions. When the polymer solution containing functionalizing reactant is cast under humid conditions containing counter reactant, a chemical reaction happens at the aqueous droplet/polymer solution interface and the product is formed in the pore of HCP film as a coating. [109-112] The benefit of this approach is that any material, either organic [109-112] or inorganic [113] can be functionalized by this method in one single step. Male et al. fabricated pore functionalized polyaniline (PANI) HCP porous films by in-situ polymerization via the BF method. [110] They added benzoyl peroxide to the PS solution and cast under reactive vapor of aniline hydrochloride, which resulted in PANI functionalized pores which otherwise might be impossible due to poor solubility of PANI in most of the solvents, as shown in
2.3.2. BF Process Accompanying Chemical Reactions with a Non-Aqueous Reactant
In the bottom-up process of the BF mechanism, the stage at which there is an interfacial interaction of water droplet and polymer solution can be applied for the site-selective functionalization. Applying this phenomenon, Huh et al. recently introduced a modified BF method, called the reactive BF (rBF) method, for the single-step fabrication of pore-selective functionalized films by accompanying an interfacial chemical reaction during the BF process [23][32][33][34][35][36]. For this method, a solvent-soluble reactant is added to the hydrophobic polymer solution, and a water-soluble counter reactant is added to the water used for humid conditions. When the polymer solution containing functionalizing reactant is cast under humid conditions containing counter reactant, a chemical reaction happens at the aqueous droplet/polymer solution interface and the product is formed in the pore of HCP film as a coating, summarized in Table 3 [32][33][34][35]. The benefit of this approach is that any material, either organic [32][33][34][35] or inorganic [36], can be functionalized by this method in one single step. Male et al. fabricated pore functionalized polyaniline (PANI) HCP porous films by in-situ polymerization via the BF method [33]. They added benzoyl peroxide to the PS solution and cast under reactive vapor of aniline hydrochloride, which resulted in PANI functionalized pores which otherwise might be impossible due to poor solubility of PANI in most of the solvents, as shown in Figure 16. Cao et al. fabricated a pore-selective carboxyl group functionalized polyimide HCP porous films using potassium hydroxide as a counter reactant in humidity as shown in
4. Cao et al. fabricated a pore-selective carboxyl group functionalized PI HCP porous films using potassium hydroxide (KOH) as a counter reactant in humidity and decorated AgNPs at the pores, as shown in
Figure 17. [111] Followed by treating with Tollen’s reagent for the decoration of Ag NPs inside the pores. Similarly, tin sulfide was functionalized in the pores of PS HCP porous films using H
5 [34]. Similarly, tin sulfide (SnS) was functionalized in the pores of PS HCP porous films using H 2
S in the humidity as the reacting agent, shown in
Figure 18. [109] The pore-selective coating of SnS was used as a template for the fabrication of moth-eye patterned film for antireflecting due to photo-responsive property under solar stimulated light illumination. [114] Modigunta et al. fabricated a temperature-sensitive polymer, poly (N-isopropyl acrylamide) (PNIPAAm) inside the pores of the HCP porous films using this same strategy. They oxidized the PS-CHO assembled inside the pores of HCP porous films using oxone to fabricate carboxylic acid (-COOH) in the pores of the HCP porous films. [112] The oxidization of the aldehyde group occurred at the interface of the water droplet/polymer solution accompanying an interfacial reaction. This PS-pf-COOH film was functionalized with amine-terminated PNIPAAm (NH
6 [32]. The pore-selective coating of SnS was used as a template for the fabrication of a moth-eye patterned film for antireflection due to its photo-responsive property under solar stimulated light illumination [37]. Modigunta et al. fabricated a temperature-sensitive polymer, poly (N-isopropyl acrylamide) (PNIPAAm), inside the pores of the HCP porous films using this same strategy. They oxidized the PS-CHO assembled inside the pores of HCP porous films using oxone to fabricate –COOH in the pores of the HCP porous films [35]. The oxidization of the aldehyde group occurred at the interface of the water droplet/polymer solution accompanying an interfacial reaction. This PS-pf-COOH film was functionalized with amine-terminated PNIPAAm (NH 2
-PNIPAAm) via EDC coupling as shown in
Figure 19. Later, they showed capturing of Ag particles in the pores of the HCP film at the LCST of PNIPAAm. This opens a plethora of prospects to immobilize various types of functional or smart materials containing hydroxyl or amine groups. The fabrication of pore-selective –COOH functionalized HCP films by the rBF method may have various applications, like controlled drug release [115,116] or biosensors [117] because the –COOH group functionalized at the pores can be further immobilized with various types of functional or smart materials containing hydroxyl or amine groups. [112,118] Recently, Falak et al. fabricated HCP porous PS films with pore-selective Ag using the rBF method accompanied by an interfacial reaction shown in
7. Later, they showed capturing of Ag particles in the pores of the HCP film at the LCST of PNIPAAm. This opens a plethora of prospects to immobilize various types of functional or smart materials containing hydroxyl or amine groups. The fabrication of pore-selective –COOH functionalized HCP films by the rBF method may have various applications, such as in controlled drug release [38][39] or biosensors [40], because the –COOH group functionalized at the pores can be further immobilized with various types of functional or smart materials containing hydroxyl or amine groups [35][41]. Recently, Falak et al. fabricated HCP porous PS films with pore-selective Ag using the rBF method accompanied by an interfacial reaction, shown in Figure 18 20. [119] In this study, an inorganic reactant AgNO
[42]. An inorganic reactant AgNO 3
was added in the aq. humidity as a functionalizing agent. On the other hand, ferrocene was included in the PS polymer solution as a reducing agent, which reduced AgNO
3 to Ag inside the pores of the HCP films. This strategy might be useful for 3D micro-patterning of biological moieties of interest to the pores for applications in tissue engineering, antibacterial membranes, protein- and cell-based biosensors, microelectronic devices, or filtration membranes. [119]
Table 3. Summary of reports using two reactants, one in the polymer solution and the other in a humid atmosphere for the functionalization of pores in HCP films.
Reactant A in the Polymer solution
|
Reactant B in Humid conditions
|
Functionalization in pore
(Product C)
|
Ref.
|
Poly (4-vinylpyridine)
|
Formic acid
|
PVP-FA precipitate
|
[120]
|
Benzoyl peroxide
|
Aniline
|
Polyaniline
|
[110]
|
Tin dichloride
|
Hydrogen sulfide
|
Tin sulfide
|
[109]
|
Polyimide
|
Potassium hydroxide
|
Carboxylic group
|
[111]
|
PS aldehyde (PS-CHO)
|
Oxone
|
Carboxylic acid (-COOH)
|
[112]
|
Ferrocene
|
Silver Nitrate
|
Silver
|
[119]
|
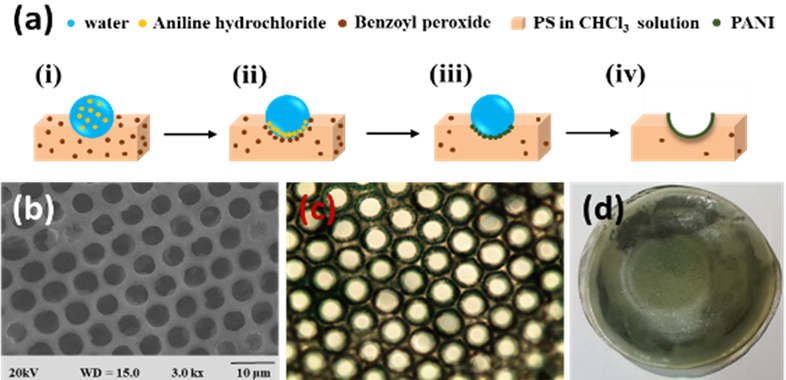
to Ag inside the pores of the HCP films. This strategy might be useful for 3D micro-patterning of biological moieties of interest to the pores for applications in tissue engineering, antibacterial membranes, protein- and cell-based biosensors, microelectronic devices, or filtration membranes [42].
Figure 164.
a) Mechanism showing the formation of PANI-f-PS film with PANI functionalized pores: (i) Condensed aq. aniline hydrochloride droplets on PS-BPO solution during BF process, (ii) assembly of aniline hydrochloride and BPO at aqueous droplet/polymer solution interface, (iii) interfacial polymerization, (iv) and formation of PANI functionalized pores after completion of reactive BF process. (b) SEM, (c) optical, and (d) digital photograph of PANI-f-PS film. Reprinted with permission from ref [110]. Copyright 2017 Polymer.
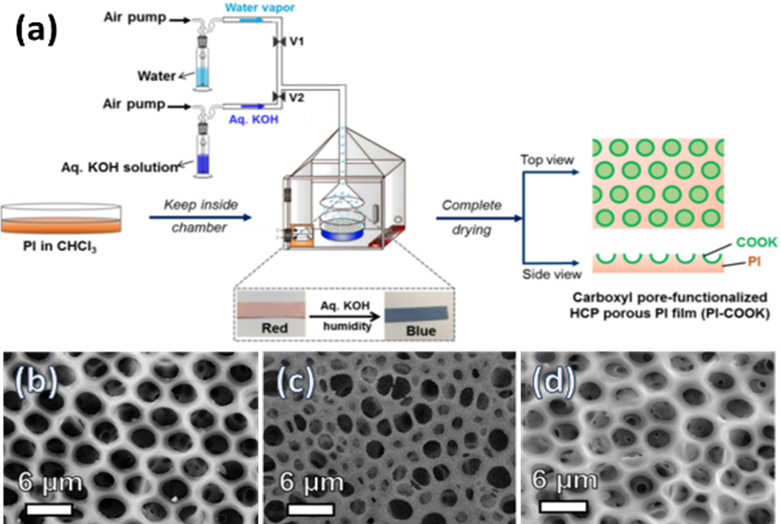
) Mechanism showing the formation of PANI-f-PS film with PANI functionalized pores: (i) Condensed aq. aniline hydrochloride droplets on PS-BPO solution during BF process, (ii) assembly of aniline hydrochloride and BPO at aqueous droplet/polymer solution interface, (iii) interfacial polymerization, (iv) and formation of PANI functionalized pores after completion of reactive BF process. (b) SEM, (c) optical, and (d) digital photograph of PANI-f-PS film. Reprinted with permission from ref. [33]. Copyright 2017 Polymer.
Figure 17. (a) Schematic representation showing the fabrication of the pore-selective carboxyl functionalized PI HCP films (PI-COOK) via BF method under aq. KOH humidity with a time interval after using initial water humidity. SEM images of PI films fabricated under (b) water humidity, (c) aq. KOH humidity, and (d) under aq. KOH humidity for 20 min after initial water humidity. Reprinted with permission from ref. [111] Copyright 2018 Polymer.
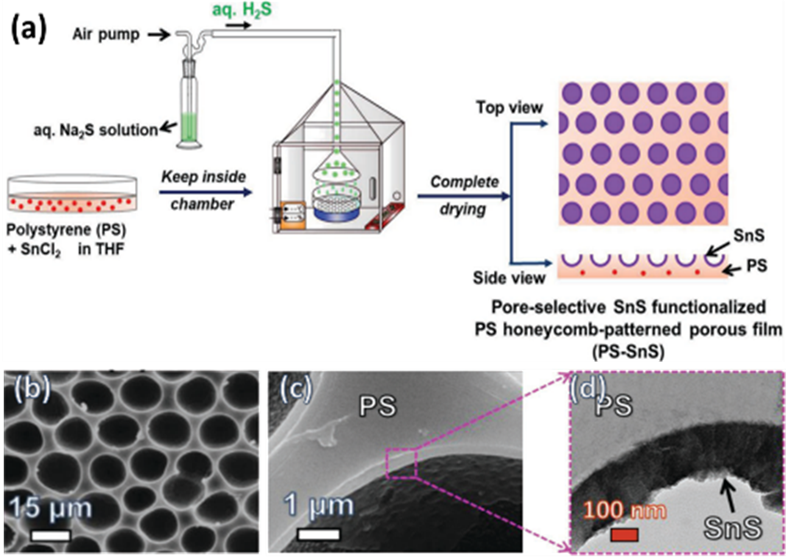
5. (a) Schematic representation showing the fabrication of the pore-selective carboxyl functionalized PI HCP films (PI-COOK) via BF method under aq. KOH humidity with a time interval after using initial water humidity. SEM images of PI films fabricated under (b) water humidity, (c) aq. KOH humidity, and (d) under aq. KOH humidity for 20 min after initial water humidity. Reprinted with permission from ref. [34]. Copyright 2018 Polymer.
Figure 186. (a) Schematic representation showing the experimental process for the pore-selective SnS-functionalized PS HCP film using a modified breath figure method. SEM images of PS HCP films fabricated by casting PS solution containing SnCl
(a) Schematic representation showing the experimental process for the pore-selective SnS-functionalized PS HCP film using a modified BF method. SEM images of PS HCP films fabricated by casting PS solution containing SnCl
2 under (b, c) aq. H
2S humidity, and (d) magnified TEM image showing a thin layer of SnS. Reprinted with permission from ref [109]. Copyright 2018 Advanced Materials Interfaces. (e) SEM image of PEDOT: PSS-SnS MEP film with SnS thin layer coated on the microdomes for the application of an antireflecting film. Reprinted with permission from ref. [112] Copyright 2019 Macromolecular Materials and Engineering.
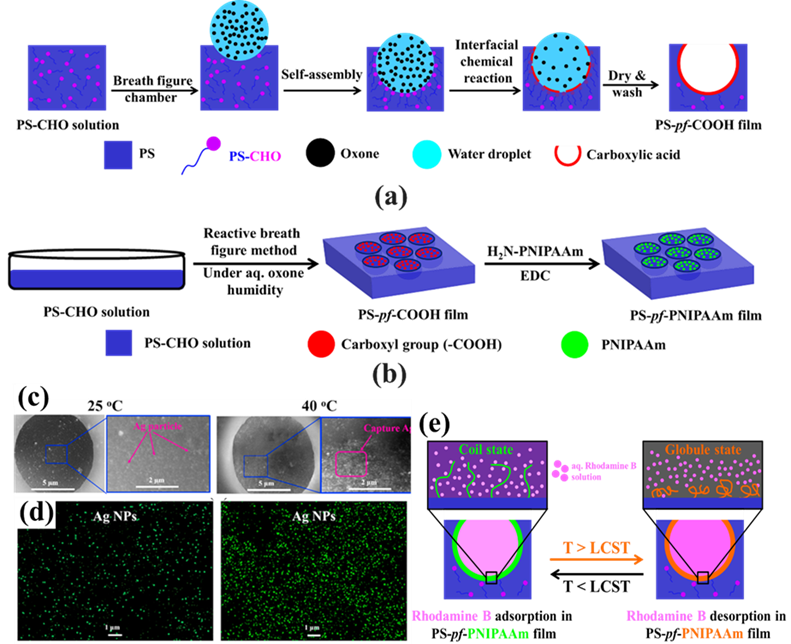
S humidity, and (d) magnified TEM image showing a thin layer of SnS. Reprinted with permission from ref. [32]. Copyright 2018 Advanced Materials Interfaces.
Figure 19. (a) The possible mechanism involved in the rBF process via self-assembly process accompanying an interfacial chemical reaction between formylated PS and oxone at the interface of the water droplet/polymer solution. (b) Schematic process for the preparation of pore-selective PNIPAAm modified (PS-pf-PNIPAAm) film by the reaction between PS-pf-COOH film and an aq. H
7. (a) The possible mechanism involved in the rBF process via self-assembly process accompanying an interfacial chemical reaction between formylated PS and oxone at the interface of the water droplet/polymer solution. (b) Schematic process for the preparation of pore-selective PNIPAAm modified (PS-pf-PNIPAAm) film by the reaction between PS-pf-COOH film and an aq. H
2N-PNIPAAm solution in the presence of EDC. (c-d) SEM images and elemental mapping show the capture of Ag particles at 25 °C and 40 °C. Reprinted with permission from ref. [112] Copyright 2020 Polymer. (e) Scheme showing the release of rhodamine B at the LCST of PNIPAAm. Reprinted with permission from ref. [115] Copyright 2021 Polymer Bulletin.
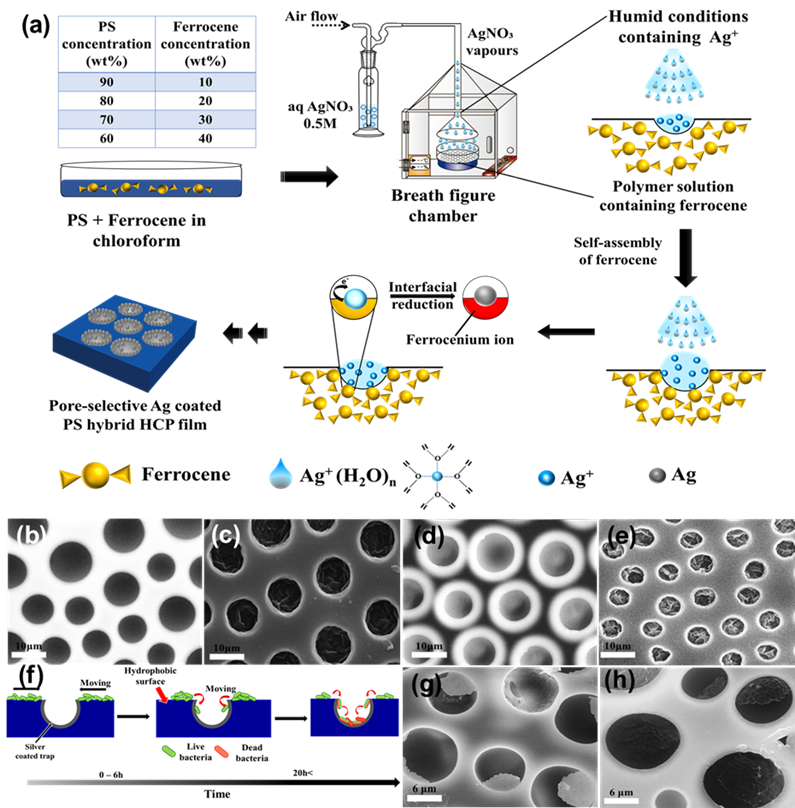
N-PNIPAAm solution in the presence of EDC. (c,d) SEM images and elemental mapping show the capture of Ag particles at 25 °C and 40 °C. Reprinted with permission from ref. [35] Copyright 2020 Polymer. (e) Scheme showing the release of rhodamine B at the LCST of PNIPAAm. Reprinted with permission from ref. [38] Copyright 2021 Polymer Bulletin.
Figure 2018.
(a) Schematic representation showing the fabrication of the pore-selective Ag functionalized PS HCP films via rBF method under aq. AgNO
3
humidity. Typical SEM images of the 10 and 20 wt% PS HCP films fabricated under (b,d) aq. humidity, and (c,e) aq. AgNO
3 humidity, respectively. (f) The mechanism for trapping of bacteria inside the pits of the Ag-functionalized porous HCP film. SEM images of (g) E. coli and (h) S. aureus showing the trapped bacteria at the pores of the HCP film. Reprinted with permission from ref. [119] Copyright 2022 Polymer.
Since the discovery of the BF method in 1994, the past decade has seen rapid developments in the BF approach. HCP films fabricated via the BF method have shown great potential in chemistry and materials science. As the porous films are composed of the polymer framework and pores, their use can be applicable to frameworks and pores. [121] Compared with the vigorous progress of the framework, the applications of pores is less reported, acting as secondary templates, [105,114,122,123] selective antibacterial surfaces, [5,113,124] catalysis, [70,71] selective cell culturing, [5,124] drug capture and release [95,115] or in protein recognition [87,89] as shown in
humidity, respectively. (f) The mechanism for trapping of bacteria inside the pits of the Ag-functionalized porous HCP film. SEM images of (g) E. coli and (h) S. aureus showing the trapped bacteria at the pores of the HCP film. Reprinted with permission from ref. [42] Copyright 2022 Polymer.
Table 3. Summary of reports using two reactants, one in the polymer solution and the other in a humid atmosphere for the functionalization of pores in HCP films.
Reactant A in the Polymer Solution |
Reactant B in Humid Conditions |
Functionalization in Pore (Product C) |
Ref. |
Poly (4-vinylpyridine) |
Formic acid |
PVP-FA precipitate |
[120] |
Benzoyl peroxide |
Aniline |
Polyaniline |
[110] |
Tin dichloride |
Hydrogen sulfide |
Tin sulfide |
[109] |
Polyimide |
Potassium hydroxide |
Carboxylic group |
[111] |
PS aldehyde (PS-CHO) |
Oxone |
Carboxylic acid (–COOH) |
[112] |
Ferrocene |
Silver Nitrate |
Silver |
[119] |
Since the discovery of the BF method in 1994, the past decade has seen rapid developments in the BF approach. HCP films fabricated via the BF method have shown great potential in chemistry and materials science. As the porous films are composed of the polymer framework and pores, their use can be applicable to frameworks and pores [43]. Compared with the vigorous progress of the framework, the applications of pores is less reported, acting as secondary templates [30][37][44], selective antibacterial surfaces [18][36][45], catalysis [21][46], selective cell culturing [18][45], drug capture and release [17][38], or in protein recognition [16][3], as shown in Figure 21. Thus, how to develop the new functions of the cavities becomes very meaningful, which will bring new vitality to the field of porous films.
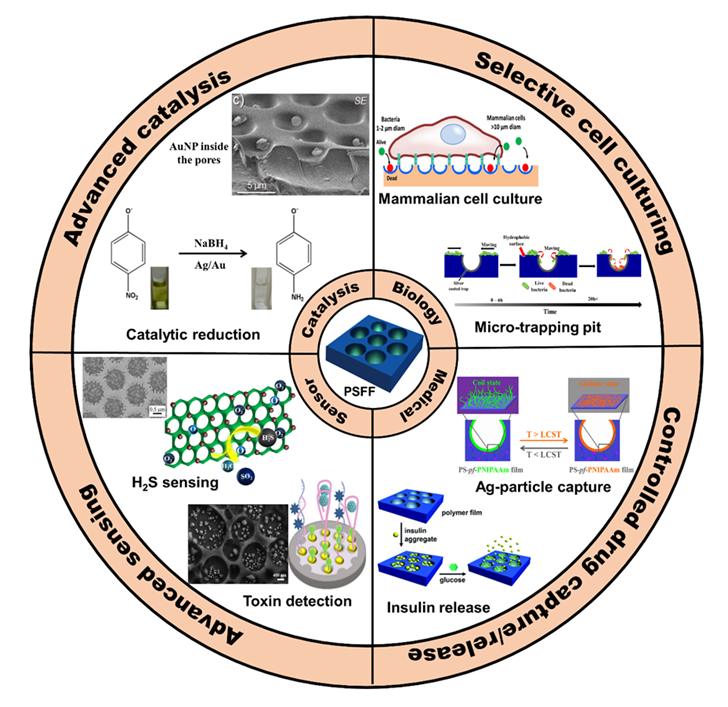
9. Thus, how to develop the new functions of the cavities becomes very meaningful, which will bring new vitality to the field of porous films.
Figure 219. Pore-selectively functionalized HCP films via the modified BF method and their applications in biology, medical, sensor application, and catalysis. PSFF- Pore-Selectively Functionalized Film.
Pore-selectively functionalized HCP films via the modified BF method and their applications in biology, medical, sensor application, and catalysis. PSFF—Pore-Selectively Functionalized Film.
3. Conclusions
Here has been one of the first attempts to thoroughly review the pore-selective functionalization of the HCP films via the modified BF methods. The researchers have focused more on the use of rBF accompanying chemical reactions for the pore-selective functionalization of the HCP films. The functionalization of pore surfaces in one step is possible by using dynamic BF processes, in which there is an active flow of vapors over the surface of the polymeric solution facilitating the evaporation of water droplets containing the chemical moieties dissolved in it, thereby facilitating the interfacial reaction between the two reactants at the interface of the polymer/water droplet and functionalization of the pore surfaces. However, in the static BF process, the effects of vapor flow could be neglected due to the passive exposure of polymeric solutions without inducing extra vapor flow. Therefore, pore-selective functionalization using the self-assembly of amphiphilic polymers is feasible with the static BF method, while pore-selective functionalization using the self-assembly accompanying the interfacial chemical reaction between two reactants is not achievable. Earlier, optimizing versatility in functionalizing these pore surface structures required an ex situ process, and the in situ functionalization was limited to surfactants that are compatible with the BF formation. Therefore, the possibility of combining the BF method with interfacial chemical reactions might push forward the development of pore-selective functionalization in both fundamental and application aspects. Although the principle of the BF method looks very simple, optimizing the concentration of reactants for the formation of products at the pores without compromising the pattern remains a challenge. In the modified BF method, the interfacial interactions between water droplets containing a reactant and the polymer solution containing the counter reactant play a key role. Understanding the kinetics and conditions of the chemical reaction is one of the most important aspects of the process. Additionally, the selection of reactants concerning their nature, solubility, or other aspects will help fabricate HCP films with the desired functionalization in a more controllable way. The traces of reactant or the irrelevant byproducts left at the pores may also be unfavorable in this system. Moreover, the chemical reactions possible for the pore-selective functionalization are limited to the formation and application of the new products formed. Nevertheless, here provides an insight for future research as the modified BF method is still in its preliminary stage.
3. Conclusion
The functionalization of pore surfaces in one-step is possible by using the dynamic BF processes, in which there is an active flow of vapors over the surface of the polymeric solution facilitating the evaporation of water droplets containing the chemical moieties dissolved in it. Thereby, facilitating the interfacial reaction between the two reactants at the interface of polymer/ water droplet and functionalization of pore surfaces. However, in the static BF process, the effects of vapor flow could be neglected due to the passive exposure of polymeric solutions without inducing extra vapor flow. Therefore, pore-selective functionalization using the self-assembly of amphiphilic polymers is feasible with the static BF method while pore-selective functionalization using the self-assembly accompanying the interfacial chemical reaction between two reactants is not achievable.
Earlier, optimizing versatility in functionalizing these pore surface structures required an ex situ process, and the in situ functionalization is limited to surfactants that are compatible with the BF formation. Therefore, the possibility of combining the BF method with interfacial chemical reactions might push forward the development of pore-selective functionalization in both fundamental and application aspects. Although the principle of the BF method looks very simple, optimizing the concentration of reactants for the formation of products at the pores without compromising the pattern remains a challenge. In the modified BF method, the interfacial interactions between water droplets containing a reactant and the polymer solution containing the counter reactant play a key role. Understanding the kinetics and conditions of the chemical reaction is one of the most important aspects. Additionally, the selection of reactants concerning their nature, solubility, or other aspects will help fabricate HCP films with the desired functionalization in a more controllable way. The traces of reactant or the irrelevant byproducts left at the pores may also be unfavorable in this system. Moreover, the chemical reactions possible for the pore-selective functionalization are limited to the formation and application of the new products formed. The fabrication of pore selective COOH opens up a plethora of opportunities for further immobilization of the smart or functional polymers, proteins, ligands, and biomolecules which extends the applications of the HCP films fabricated via the modified BF method.