Systemic sclerosis (SSc) is a severe auto-immune, rheumatic disease, characterized by excessive fibrosis of the skin and visceral organs. SSc is accompanied by high morbidity and mortality rates, and unfortunately, few disease-modifying therapies are currently available. Inflammation, vasculopathy, and fibrosis are the key hallmarks of SSc pathology. There are current and novel treatment options in diminishing SSc-related fibrosis based on selected clinical trials.
1. Introduction
Systemic sclerosis (SSc) is a severe rheumatic, auto-immune disease which affects up to 20 people per 100,000 and women up to nine times more often than men
[1][2][3]. Inflammation, vasculopathy, and fibrosis are the key hallmarks of SSc. Patients suffer from fibrotic skin lesions, and as the disease progresses, the function of internal organs including the heart, lungs, gastrointestinal tract, and kidneys deteriorates due to fibrosis. The disease has a high morbidity and greatly negatively affects the quality of life and life expectancy of patients.
Although the pathophysiology of SSc has not been elucidated yet, the immune system has been hypothesized as an important driver of the disease (
Figure 1). SSc patients exhibit disease-specific autoantibodies, and a typical cytokine profile in their blood indicating immune cell activation. This profile is characterized by increased T helper 2 (Th2) and decreased T helper 1 (Th1) cytokines
[4][5]. Furthermore, in skin biopsies of early disease patients, perivascular accumulation of immune cells, such as CD4+ and CD8+ cytotoxic T cells (CTLs), can be observed
[6]. This immune activation can cause (or is maybe a response to) capillary damage, which leads to capillary breakdown, adherence of platelets, and activation of pro-fibrotic pathways. Additionally, vascular injury causes damage and apoptosis of endothelial cells. The release of internal damage-associated molecular patterns (DAMPs) increases microvascular permeability which causes additional recruitment of immune cells to the endothelium and therefore increased immune cell activation and inflammation
[7].
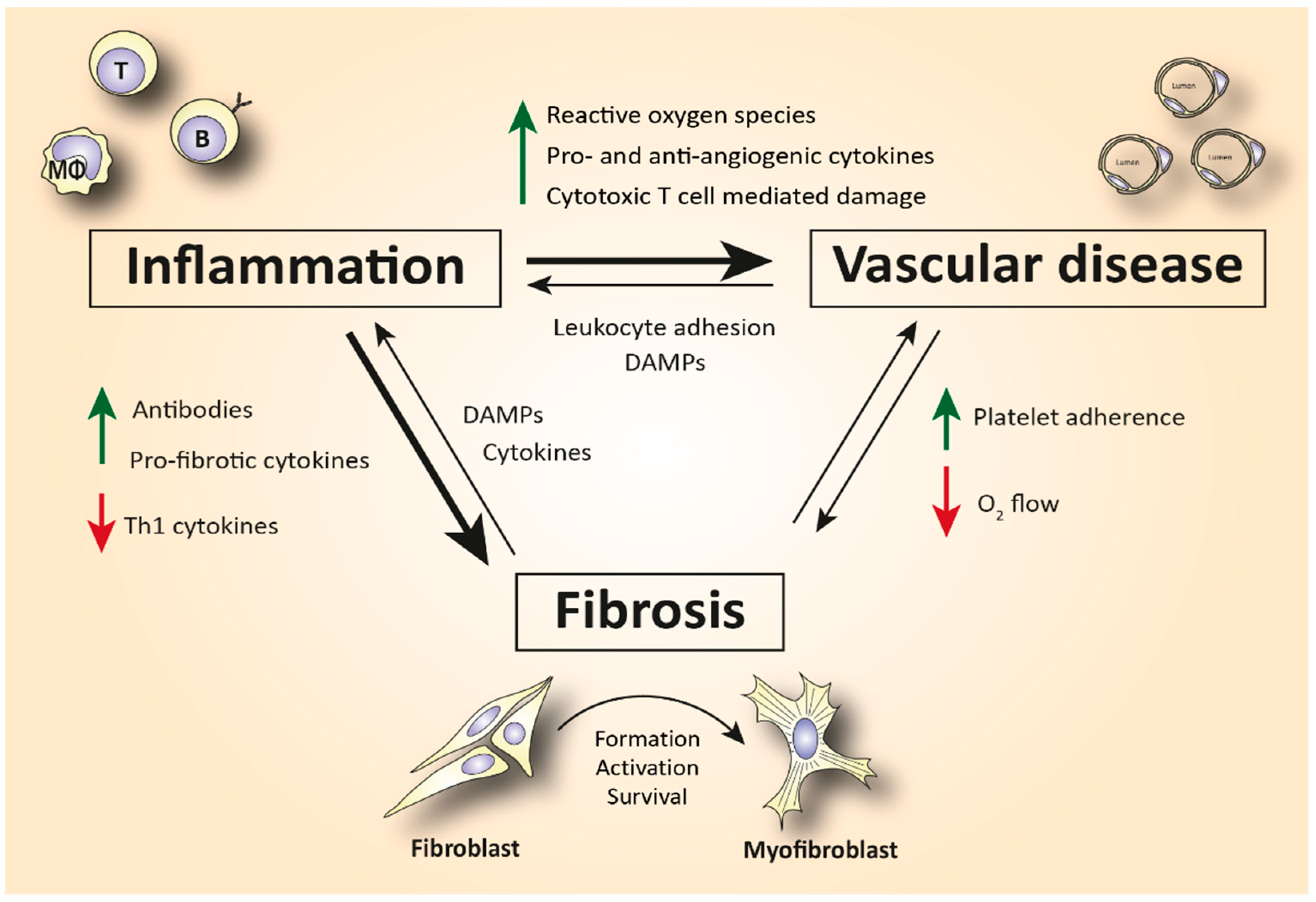
Figure 1. Simplified schematic representation of the complex interplay between inflammation, vasculopathy, and fibrosis in SSc. Abbreviations: Th1 = T helper type 1 cells; DAMPs = damage-associated molecular patterns; T = T lymphocyte; B = B lymphocyte; MΦ = macrophage.
Activation of the immune system is also linked to fibrosis. For example, Th2 cytokines, such as interleukin (IL)-4 and -13, can activate myofibroblasts
[8]. Myofibroblasts are a strongly pro-fibrotic cell type which produces large amounts of extracellular matrix (ECM) molecules and matrix-strengthening enzymes. Furthermore, these cells also excrete growth factors and cytokines that worsen inflammation
[9]. IL-4, IL-6, and transforming growth factor (TGF)-β are the predominant fibrogenic cytokines that cause subendothelial accumulation of fibrous tissue, leading to aberrant vascular remodeling
[10]. In turn, this aberrant vascular remodeling makes the capillaries more prone to damage, fueling an immune response.
The important role of the immune system in the pathogenesis of SSc is further supported by the fact that autologous hematopoietic stem cell transplantation (ASCT) can induce long-term disease remission in patients with SSc. However, ASCT is accompanied by a high mortality rate (~10%) and is only administered in the most severe cases of SSc
[11]. Currently, there is no specific and effective disease-modifying treatment available, which, regarding the severity of the disease, results in an unmet medical need. Presently, mainly broad-spectrum immunosuppressive and anti-inflammatory drugs, developed for other auto-immune diseases, are used to treat SSc.
2. Evaluation of Cell-Specific Anti-Inflammatory Treatment
On the road towards personalized therapy in SSc, specific elimination of pathogenic innate or adaptive immune cell populations could be promising in alleviating fibrosis and reducing the morbidity of the side effects of broad immunosuppression. Of note, there are no clinical trials evaluating drugs depleting innate immune cell populations. On the other hand, several trials (
Table 1) have evaluated the efficacy of B and T cell-depletive therapies in SSc, and thus herein begins with addressing treatments targeting these cells first
[12].
2.1. B Cell-Specific Treatment
To begin with B cell-specific treatment, rituximab (RTX), belimumab, and inebilizumab are the three representative monoclonal antibodies that have been used.
2.1.1. Rituximab
Rituximab is a chimeric anti-CD20 monoclonal antibody that binds to both immature and mature B lymphocytes expressing CD20 on their surface (including the pathogenic Beffs) and eliminates them (
Figure 2). Of note, RTX does not consistently succeed in depleting B cells in tissues
[13][14], and antibody-producing plasma cells are not targeted by RTX, since they lack the CD20 surface antigen
[15]. Indeed, in a small, randomized, double-bind, placebo-controlled trial, treatment with RTX was not associated with reduced fibrosis but caused significant depletion of naïve and memory B cells in the peripheral blood and scleroderma-associated dermal lesions
[16]. As expected from RTX’s mode of action, various plasma cells and autoantibody titers did not show a statistically significant change after treatment. The potential anti-fibrotic effect of RTX is supported by histopathological observations suggesting a reduced dermal hyalinized collagen content and myofibroblast count
[17]. As discussed, IL-6 is a cytokine largely produced by activated B cells that shows pro-fibrotic capacity. In a small, open-label study where RTX significantly improved patients’ skin scores, it was observed that patients had elevated basal levels of IL-6 in biopsies from their skin lesions. IL-6 levels decreased from 3.7
±
5.3 pg/mL at baseline to 0.6
± 0.9 pg/mL (
p = 0.02) six months post-treatment. Furthermore, after treatment 7/9 patients exhibited a complete depletion of B cells in skin lesions. Thus, interestingly, the RTX-associated skin thickness was correlated with B cell depletion and IL-6 reduction in patients’ skin
[18]. This observation further empowers the mechanism and role of RTX in skin fibrosis.
Figure 2. Schematic representation of the mechanism of action of synthetic corticosteroids (CS), mycophenolate mofetil, cyclophosphamide, inebilizumab, rituximab, belimumab, abatacept, nintedanib, tocilizumab, tofacitinib, fresolimumab, metelimumab, and pirfenidone in reducing fibrosis in SSc. Abbreviations: ECM = extracellular matrix; TGF-β = transforming growth factor-β.
2.1.2. Inebilizumab and Belimumab
Since depletion of CD20+ B cells does not consistently deplete B cells in tissues and does not eliminate plasma cells, developing monoclonal antibodies that target the bone marrow-resident pro- and pre-B cell co-receptor CD19 might be a more effective B cell-depletive approach (
Figure 2). The efficacy of a humanized anti-CD19 monoclonal antibody, inebilizumab (MEDI-551), in reducing SSc fibrosis was assessed in a phase I randomized, double-blind, placebo-controlled study
[19]. This therapy effectively depleted both B cells and plasma cells in the skin and blood of SSc patients in a dose-dependent manner. Furthermore, an improved skin score was observed in all patients, suggesting a role of inebilizumab in halting skin fibrosis. Strikingly, patients with an elevated plasma cell signature at inclusion showed greater improvement in modified Rodman skin thickness score (mRSS) compared to the patients that had normal or lower plasma cell counts
[20].
Belimumab is another human monoclonal antibody targeting the soluble B cell activating factor (BAFF) cytokine, a member of the tumor necrosis factor ligand superfamily that was a breakthrough in the treatment of systemic lupus erythematosus (
Figure 2). This monoclonal antibody prevents the binding of BAFF to receptors on B cells and inhibits the survival of B cells, including the autoreactive ones. It further decreases the differentiation of B cells into immunoglobulin-producing plasma cells
[21]. In a randomized, double-blind, placebo-controlled, pilot trial, 20 patients with diffuse cutaneous systemic sclerosis (dcSSc) recently treated with MMF (1 gr twice a day) were administered 10 mg/kg of belimumab i.v., or placebo. Belimumab administration improved the mRSS score from 27 to 18 (
p = 0.039), while the improvement in the placebo group was from 28 to 21 (
p = 0.023). Patients with improved mRSS exhibited a significant reduction in both B cell and pro-fibrotic gene expression. However, the results of this small pilot study were not statistically significant, and larger trials should be conducted in order to make treatment recommendations
[22]. A study evaluating the combination therapy of belimumab and RTX in SSc is currently pending (NCT03844061).
2.2. T Cell-Specific Treatment
The elevated infiltration of cytotoxic T cells in SSc-affected skin and lungs, along with the increased Th2 pro-fibrotic cytokine levels in the serum of SSc patients, suggests that targeting T cells could be a promising treatment option in SSc. T cell-specific treatment has shown encouraging results in other inflammatory diseases such as rheumatoid arthritis (RA)
[23]. The biologicals that have been used either directly affect T cell activity by reducing their number, or they indirectly interact with cytokines expressed by T cells. Abatacept is the main representative of the former category, and it causes T cell deactivation by blocking CD28 (
Figure 2), a molecule that is essential for T cell activation mediated by antigen-presenting cells (APCs)
[24]. CD28 knockout mice exhibited decreased fibrosis in comparison with the wild type in bleomycin-induced fibrosis
[25]. In 2015, the efficacy of abatacept in attenuating fibrosis was examined in a placebo-controlled RCT that included 10 patients with dcSSc
[26]. Five out of seven patients that received abatacept showed a ≥30% decrease in mRSS, while this improvement was shown in one out of the three controls. Interestingly, patients with decreased mRSS also showed reduced CD28 gene expression along with decreased expression of genes associated with T cell proliferation. This empowers the proposed mechanism of abatacept’s action. Recently, a phase II, multicenter, double-blind, randomized, placebo-controlled trial assessed the safety and efficacy of subcutaneous administration of abatacept in 88 dcSSc patients
[27]. The results failed to show a statistically significant improvement in mRSS (primary outcome) in the abatacept arm, but secondary outcomes reflecting patients’ disability and quality of life were significantly improved in favor of the abatacept group. Of note, patients were stratified into inflammatory, normal-like, and fibroproliferative subgroups based on molecular gene expression data of their skin biopsies. Patients with an inflammatory intrinsic gene expression showed a larger decrease in mRSS compared to the other groups. Interestingly, the improvement in the skin score was associated with decreased gene expression of immune pathways including cytotoxic T lymphocyte-associated protein 4 (CTLA-4) and CD28 (targets of abatacept). These results are novel regarding the potentially targeted mechanism of action of abatacept as an inflammation immunomodulator. Furthermore, abatacept exhibits a good safety profile and seems to decrease joint involvement and related disability
[28][29]. Based on these studies, abatacept seems to be effective in treating scleroderma-associated joint inflammation, but studies with a larger number of participants (phase III) are required to evaluate its anti-fibrotic effect.
Table 1. Representation of the studies evaluating the role of B and T cell-specific treatments in SSc.
Drug |
Target |
Type of Trial(s) |
Duration (Months) |
Patients |
Results |
Rituximab (RTX) |
Anti-CD20 B cell depletion |
1. Double-blind, RCT [16] |
1. 24 |
1. 16 early SSc |
1. B cell depletion in blood did not improve mRSS and forced vital capacity (FVC) scores |
2. Open-label trial [18] |
2. 54 |
2. 9 dcSSc |
2. Median decrease in mRSS of 43.3% (p = 0.001) accompanied by reduction in IL-6 levels |
3. Open-label RCT [30] |
3. 24 |
3. 14 SSc |
3. FVC improved by 10.25% in RTX and reduced by 5.04% in the placebo group (p = 0.0018). RTX arm: mRSS improvement of 13.5 vs. 8.37 in placebo, 12 months post-treatment (p < 0.001) |
4. Multicenter, open-label trial [31] |
4. 48 |
4. 51 SSc-interstitial lung disease (ILD) |
4. No significant change in mRSS, but lung function improved significantly |
5. Open-label RCT [32] |
5. 6 |
5. 60 early dSSc |
5. Significant improvement in RTX vs. cyclophosphamide (CYC) in median percentage of FVC (67.52 vs. 58.06, p = 0.003), but not in mRSS scores |
6. Multicenter, double-blind RCT [33] |
6. 6 |
6. 57 SSc-associated pulmonary arterial hypertension (SSc-PAH) |
6. RTX arm: the improvement in median 6MWD was 25.5 m compared to 0.4 m in placebo (p = 0.03) after 48 weeks |
Inebilizumab (MEDI-551) |
Anti-CD19 B cell depletion |
Multicenter, double-blind RCT [19] |
3 |
28 SSc |
Depletion of B and plasma cells was correlated with improved mRSS and reduced expression of fibrotic genes in skin biopsies |
Belimumab |
Inhibition of B cell survival by blocking BAFF |
Double-blind RCT [22] |
13 |
20 dcSSc |
mRSS score improved from 27 to 18 (p = 0.039), while in placebo group from 28 to 21 (p = 0.023) |
Abatacept |
CD28 blocking T cell depletion |
1. RCT [26] |
1. 10 |
1. 6 dcSSc |
1. 5/7 patients and 1/3 controls showed >30% improved mRSS |
2.Multicenter, double-blind RCT [27] |
2. 12 |
2. 88 dcSSc |
2. No significant improvement in mRSS, but secondary outcomes related to quality of life improved significantly |
3. Emerging Therapies with Tyrosine Kinase Inhibitors
Tyrosine kinases are enzymes that use adenosine triphosphate (ATP) to transfer a phosphate group to intracellular proteins. Phosphorylation of proteins by kinases is vital for signal transduction and regulation of cell proliferation, differentiation, migration, and development. The pathological activation of tyrosine kinases (TKs) is crucial in multiple disease processes such as carcinogenesis, vascular remodeling, and fibrogenesis
[34][35]. Furthermore, in inflammatory lesions, tyrosine kinases are also activated through over-production of growth factors and/or cytokines from the tissues
[36]. Several current studies suggest that inhibiting tyrosine kinases may lead to an effective anti-inflammatory therapy. Of note, targeting TK activity is feasible with monoclonal antibodies designed to target the extracellular domains of the receptors, or with small molecules that enter the cytoplasm and bind to intracellular catalytic domains of both receptor and non-receptor TKs
[37] (
Table 2).
3.1. Indolinone-Derived Small Molecule Tyrosine Kinase Inhibitors
Imatinib mesylate is the principal compound among the small molecule TK inhibitors and was approved by the FDA in 2001 for the treatment of chronic myelogenous leukemia, in order to block the Ab1 kinase activity
[38]. Its use in SSc has been examined due to its ability to inhibit the PDGF receptor and TGF-β signaling pathways
[39]. Several case studies
[39][40][41][42][43] have examined the efficacy and tolerability of imatinib in scleroderma patients. Moderate improvement in skin scores and predicted FVC has been reported, along with a large number of adverse effects. From a mechanistic point of view, imatinib successfully depleted patients’ pDCs, immune cells that play an important role in the disease’s pathogenesis
[43][44]. Of note, treatment with imatinib exhibited a reduced amount of pathogenic IL-4-producing T cells in bronchoalveolar lavage of SSc patients, which was accompanied by elevated numbers of total T helper cells. Based on this observation, it could be suggested that imatinib’s anti-fibrotic capacity could be mediated via shifting the Th2 response to a non-type 2 T helper phenotype
[41]. In an open-label, multicenter study, 16 out of 27 patients with early dcSSc completed a six-month imatinib administration. The mean decrease in mRSS was 21% compared to baseline; however, five patients exhibited severe adverse effects including generalized edema, erosive gastritis, anemia, upper respiratory tract infection, neutropenia, and neutropenic infection
[44]. Therefore, based on the moderate improvement and the severe adverse effects that were observed, the use of the imatinib’s analog nilotinib was proposed.
Nilotinib has the same mechanism of action as imatinib, but it is 20–30-fold more potent and has a more favorable toxicity profile
[45]. The use of nilotinib in SSc has been tested in an open-label, single-arm, phase II trial that included 10 scleroderma patients. In this trial, nilotinib was well tolerated by the majority of the participants (7/10), with only a few cases of increased edema and mild QTc prolongation being reported. Furthermore, it was observed to be promising in reducing mRSS scores in patients with early and active disease (reduction of 16%,
p = 0.02, and 23%,
p = 0.01, at 6 and 12 months post-treatment, respectively)
[46]. Furthermore, data from skin gene expression profiling showed that patients responding to treatment were characterized by elevated TGF-β signaling. Interestingly, the expression of TGF-β signaling genes was significantly reduced after these patients were treated with nilotinib. This observation empowers the mechanism of action of nilotinib and supports its potential anti-fibrotic effect. However, the progression into phase III trials will help to validate its efficacy in reducing fibrosis and also determine its limitations concerning potential side effects.
The last indolinone-derived small molecule inhibitor that will be discussed is nintedanib. Nintedanib inhibits a plethora of molecules implicated in fibroblast activation such as PDGF receptor, fibroblast growth factor receptor (FGFR), and vascular endothelial growth factor receptor (VEGFR) (
Figure 2). In vitro and animal data support the anti-fibrotic effect of nintedanib in inhibiting crucial pathways in the initiation and progression of lung fibrosis. For instance, nintedanib inhibits the secretion of the pro-fibrotic cytokines IL-4, IL-5, and IL-13 in the peripheral blood of SSc patients. In addition, its anti-fibrotic effect is partly mediated by preventing the polarization towards the pro-fibrotic M2 macrophages
[47]. Nintedanib may also exert its anti-fibrotic efficacy by restraining the migration and differentiation of fibroblasts and fibrocytes. Interestingly, in vitro data have shown that nintedanib downregulates the transition of fibrocytes towards myofibroblasts, and thus the pro-fibrotic effect of the latter is reduced
[48][49]. Nintedanib has received FDA approval for the treatment of IPF. Since IPF shares pathogenic commonalities with SSc-ILD, the use of nintedanib to reduce lung fibrosis in patients with SSc-ILD has been recently evaluated in the SENSCIS trial
[50]. This trial included 576 patients with SSc-ILD that were randomly assigned (1:1 ratio) to receive 150 mg of nintedanib two times daily or placebo for 52 weeks. Deterioration of lung function in the nintedanib arm was significantly lower compared to the control group. More specifically, the adjusted annual rate of decline in FVC was −52.4 mL in patients treated with nintedanib compared to −93.3 mL in the placebo group (
p = 0.04). Based on the results
of this study, in 2019, nintedanib was the first FDA-approved treatment for patients with SSc-ILD
[51]. It is worth mentioning that no significant clinical benefit was observed in skin fibrosis measured by mRSS over a 1-year period. Until now, no clinical data verifying the in vitro and in vivo anti-fibrotic mechanisms have been published to date. An open-label, extension trial, assessing the long-term safety and efficacy of nintedanib in 444 SSc-ILD patients, is ongoing and expected to provide data on prolonged nintedanib therapy and its exact mechanism of action in SSc patients (NCT03313180).
3.2. Tofacitinib
Except for the Ab1 and PDGF tyrosine kinases, inhibition of Janus kinases (JAK) is another potential treatment with anti-inflammatory properties. Recent data provide evidence that the JAK/STAT signaling pathway is highly activated in SSc skin biopsies
[52]. Tofacitinib is a small molecule JAK inhibitor, with a structure similar to ATP, and has been used in the treatment of RA. Regarding its mechanism of action, it enters the cell with passive diffusion and binds to the ATP site of the JAK1 and JAK3 receptors. As a result, it inhibits the phosphorylation and activation of signal transducer and activator of transcription proteins (STATS), which leads to the downregulation of IL-6 expression
[53] (
Figure 2). In view of this mechanism, the use of tofacitinib in SSc might be of interest. The results from an observational study suggest tofacitinib as a potential treatment in reducing skin thickening of patients with refractory dcSSc
[54]. More specifically, tofacitinib-treated patients showed a significantly shorter response time compared to the conventional immunosuppressive group, with a mean change of −3.7 (
p = 0.001) in mRSS already 1 month post-treatment. After 6 months of treatment, the mean change in mRSS was even greater between the two groups (−10.0 tofacitinib vs. −4.1 conventional immunosuppressants,
p = 0.026). Similarly, in another pilot study, patients treated with tofacitinib showed a significantly higher improvement in skin fibrosis compared to patients treated with methotrexate (MTX)
[55]. These results indicate that tofacitinib might be even more effective in reducing fibrosis than conventional immunosuppressants, further showing a quicker and higher response rate. The tolerability and efficacy of tofacitinib have been recently evaluated in a small clinical phase I/II trial in 15 SSc patients. According to the preliminary results, tofacitinib is well tolerated and shows a trend towards improving fibrosis (NCT03274076). Further evaluation of tofacitinib in dcSSc seems warranted.
Table 2. Clinical trials evaluating the efficacy of tyrosine kinase inhibitors in SSc treatment.
Drug |
Target |
Type of Trial(s) |
Duration (Months) |
Patients |
Results |
Imatinib mesylate |
Inhibits PDGFR and TGF-β signaling |
Multicenter, open-label RCT [44] |
6 |
27 dcSSc |
Mean decrease in mRSS was 21% compared to baseline (p < 0.001) |
Nilotinib |
Same as imatinib, but 20–30-fold more potent |
Open-label, single-arm trial [46] |
8 |
10 early dcSSc |
Promising reduction of 23% in mRSS 12 months post-treatment (p = 0.01) |
Nintedanib |
Inhibits PDGFR, FGFR, and VEGFR signaling |
Double-blind RCT (SENSCIS) [50] |
13 |
576 SSc-ILD |
Adjusted annual rate of decline in FVC −52.4 mL compared to −93.3 mL in the placebo group (p = 0.04) |
Tofacitinib |
Inhibits JAK/STAT signaling |
Double-blind RCT (NCT03274076) |
6 |
15 dcSSc |
Preliminary data show a trend towards improved fibrosis |
4. Conclusions and Future Perspectives
SSc is a disabling, chronic, auto-immune disease accompanied by high mortality and morbidity rates. Herein examined the potential effects of anti-inflammatory and immunosuppressive treatments in attenuating fibrosis. To do this, herein evaluated the first-line, generalized as well as cell- and cytokine-specific anti-inflammatory and anti-fibrotic treatments, including the main therapies that have been used or are currently being tested in clinical trials.
To begin, among the broad-spectrum treatments, MTX has been well tolerated, but its efficacy in reducing fibrosis is still controversial. Although CYC’s use seems efficient in reducing fibrosis, its high toxicity limits its use, and it has now been replaced with MMF. MMF is well tolerated, but its use has not been fully examined. On the other hand, cellular targeting of inflammation with molecules that reduce the number of B or T cells, such as rituximab, belimumab, and abatacept, has exhibited a potential effect in diminishing fibrosis compared to broad-spectrum immunosuppressants. Furthermore, the cytokine signaling-specific antibodies rilonacept, basiliximab, fresolimumab, and metelimumab show a trend towards reducing fibrosis, but the lack of large phase III trials limits their potential addition to SSc treatment guidelines. Among the TK inhibitors, nintedanib and tofacitinib are two promising therapies in halting SSc-related fibrosis. Based on encouraging outcomes from large phase III RCTs, tocilizumab and nintedanib have been FDA approved for the treatment of SSc-ILD. On the positive side, ASCT shows increased event-free and improved overall survival rates, but its use is limited to younger patients with early dcSSc that fulfill a list of very strict inclusion criteria. Careful patient selection is vital in reducing the relatively high mortality rates that accompany ASCT.
In conclusion, scientists are in a new era of a multitude of clinical trials with drugs targeting specific pathogenic cells and biological pathways related to SSc. Several anti-inflammatory treatments have been used or tested in SSc patients, but only a mild to moderate improvement in reducing fibrosis has been demonstrated. However, the level of published evidence on the effectiveness of each tested drug varies greatly among case series and observational studies, and only a few RCTs have been conducted
[56]. Thus, conclusions and comparisons between the efficacy of different drugs should be handled with caution. The anti-inflammatory approaches described show a trend towards reducing fibrosis, but the effect is moderate and, in many cases, controversial. The lack of complete understanding of the pathophysiology and the rare frequency of the disease are two obvious arguments that support this conclusion. Furthermore, SSc is a very complex and heterogeneous disease, and the traditional classification of the patients into the limited or diffuse form based on the severity of skin involvement is an oversimplification. Lately, multiple studies have utilized intrinsic gene expression analyses to classify SSc patients into four categories: the inflammatory, fibroproliferative, limited, and normal-like subsets
[57]. It is currently not certain if these categories are reflective of stable disease states that differ between patients. Other studies suggest that they concern different stages of the SSc disease process
[58]. Various disease manifestations of SSc, such as ILD, pulmonary hypertension, and gastrointestinal disease, develop with a time course that differs from that of the skin manifestations. The underlying maladaptive innate and adaptive immune responses likely differ between these pathologies. Taken together, it is believed that in-depth insight and measurement tools of the pathological processes may yield markers to determine the biological processes driving specific disease manifestations. Such markers will help to determine the best treatment approach in individual cases.
Furthermore, targeted drug selection is expected to show more remarkable results in the anti-inflammatory therapies that have been mentioned. Additionally, to understand if new drugs are effective, there is also a need for a better understanding of the pathological processes driving disease features. The use of novel and advanced molecular tools such as single-cell RNA sequencing is constantly advancing
ourthe knowledge about novel pathogenic cytokines, antibodies, and genes implicated in the pathogenesis of SSc. This knowledge will facilitate more personalized treatments. For example, scleroderma patients that exhibit high amounts of the Th2 cytokines IL-4 and IL-13 early after diagnosis, or those with prominent ILD, could be treated with immunotoxins or monoclonal antibodies that have been designed to block the IL-4 or IL-13 pathway and have shown promising results in anti-tumor, IPF, and asthma treatment. Another suggestion for future studies would be to evaluate the outcome of combined biological therapies.