1. Introduction
The rapid appearance of organic optoelectronics as a promising alternative to conventional optoelectronics has been largely achieved through the design and development of new conjugated systems [1]. The foremost advantage of organic materials is that they are cheap, lightweight, and flexible and they can offer the opportunity to make the electronics sector greener through the use of abundant materials and manufacturing methods rely on fewer, safer, and more abundant raw materials and with cheaper processing based on solution techniques so the embodied energy for organic devices is expected to be lower than for their inorganic counterparts usually processed by vacuum and high-temperature techniques [2][3][4][5][6][7][2–7].
Since its discovery [8], the 4,4-difluoro-4-bora-3a,4a-diaza-s-indacene (BODIPY) molecule has been the subject of considerable interest thanks to its chemical stability, easy functionalization, and interesting optical and electronic properties combined with low toxicity and high biocompatibility and it has therefore emerged as a promising platform for multiple optoelectronic applications [9][10][11][12][9–12]. This type of structure is commonly described as an example of a “rigidified” monomethine cyanine dye or, as it has three p-delocalized rings, one is pyrrole and the others are azafulvene and diazaborinin-type ring systems [13], by analogy with the all-carbon tricyclic ring can be considered as a boradiazaindacene, and the numbering of any substituents follows rule setup for the carbon polycycles (see Figure 1). This dye has also been [14] called “porphyrin’s little sister” and this happy definition has been so successful that in analogy with porphyrinic systems, the 8-position is often referred to as the meso site.
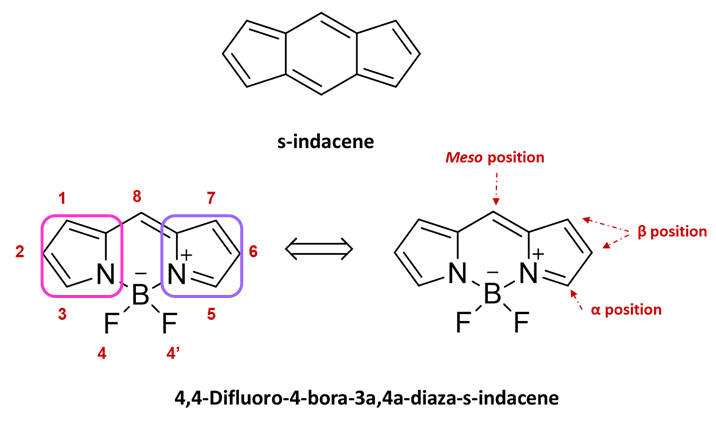
Figure 1. Naming and numbering of the 4,4-difluoro-4-bora-3a,4a-diaza-s-indacene (BODIPY)-based on s-indacene. Pyrrole ring pink boxes and azafulvene violet boxes Reproduced from Ref. [12] with permission of Taylor and Francis.
Over the years, several synthetic strategies have been implemented which have allowed the modulation of the optoelectronic properties of the BODIPY that is assumed to have two equivalent resonance forms resulting in the forming of two rings named azafulvene and pyrrole. Pyrrole is aromatic whereas azafulvene is a quinoid-type ring and it is not possible to distinguish the two forms [13]. Depending on the position and type of the substituents, it is possible to modify different parameters such as the delocalization of the π-electrons in the molecule and the supramolecular organization and the morphology of the solid thin film [15][16][17][15–17]. In this regard, the BODIPY core has eight reactive positions [18] that can be used to modulate its optical properties [19][20][19,20] (Figure 1): the two Boron positions, the two α-positions, the four β-positions, and the meso-position. The positions α and β are those which have the greatest influence on the electronic delocalization, while the other positions, meso and Boron, have a lower impact on electron delocalization but have a strong impact on the steric hindrance of the molecule.
BODIPY-based materials are playing an increasingly important role in the field of organic semiconductors as demonstrated by the high number of citations about 11,000 achieved from 2019 (Google scholar source) of which 5300 in the year 2020.
2. BODIPY-Based Active Materials in OPV
Renewable energy generated by solar cells is one of the potential solutions to the problem of maintaining our energy supply. Organic solar cells have the potential to be part of the next generation of low-cost solar cells. An encouraging state-of-the-art device has exhibited power conversion efficiencies (PCEs) over 17% [30], which represents a crucial step toward the commercialization of OPVs. Among the rapid development of optical-active donor/acceptor materials, interface materials, and device engineering, the properties of donors and acceptors, such as absorption, energy levels, and bandgaps, play a vital role in realizing high PCEs. Therefore, the evolution of new photovoltaic materials with a smaller optical bandgap (Eg) reasonable highest occupied molecular orbital (HOMO) and lowest unoccupied molecular orbital (LUMO) levels is crucial to further improve the PCEs of OPVs [31]. For these reasons, in the last 10 years BODIPY-based molecules have been of great interest as active materials for photovoltaic devices, thanks to their good absorbance at low energies (low optical gaps), high molar extinction coefficients, good chemical-stability, and opportune redox properties [23]. Table 1 summarizes the results in OPV, from the first work on the topic until 2020, where a BODIPY-based molecular material has been used as an active layer and the main parameters of a photovoltaic device are mentioned such as the current density J, the fill factor (FF), and the power conversion efficiency (PCE), where the FF is a measure of the quality of the solar cell and it is calculated by comparing the maximum power with the theoretical one [32].
Thanks to the planar core, easy lateral functionalization, and electron-deficient characteristics BODIPY derivative is an ideal acceptor unit [33] for the design of donor–acceptor D–A type semiconducting architectures [34][35][34,35] which is one of the most used approaches to modulate the optoelectronic properties of organic semiconductors [36][37][38][36–38]. The BODIPY derivatives have been used both as donors and acceptors in OPV devices, in direct and inverted architecture [23]. Some of the most interesting results have recently been obtained using conjugated polyelectrolytes at the interface with the metal cathode [29][39][29,39]. This class of materials allows the engineering of the interface [40][41][40,41] between the metal and the active layer improving the extraction of the charge without complicating the preparation of the device. In fact, for the preparation of films, it is possible to use solution techniques thanks to the use of solvents orthogonal to the active layer. It is important to underline that the parameters that influence the OPV efficiency as mentioned before, are many. It is not always possible to evaluate the individual contribution because they are often intimately connected and closely related to the chemical structure and the choice of position, type, and the number of lateral substituents.
2.1. BODIPY Molecules as Electron Acceptor Materials in Organic Solar Cells
The fullerene has been, so far, the most common material used as an acceptor in an organic solar cell, however in recent years the search for non-fullerene acceptors has gained significant attention. At the same time, there has also been an increasing effort in the development of small molecules to be used as active materials for OPVs, due to the potential control of frontier orbital energy level that they offer [43]. The combination of these two interests has triggered research for small molecules, non-fullerene acceptors, for bulk heterojunction (BHJ) OPV.
To the best of our knowledge, in early 2021, only few research groups have reported on the synthesis and application of non-fullerene acceptor based on BODIPY.
In 2014, Thayumanavana et al. [44] presented a series of acceptor–donor–acceptor molecules containing terminal BODIPY unit conjugated through meso-position using a 3-hexylthiophene linker to thiophebenzodithiophene, cyclopentadithiophene, or dithienopyrrole. The three molecules have deep LUMO energy levels of −3.79 eV, −3.82 eV, and −3.74 eV, and good visible absorption, with absorption maxima in thin-film at 532 nm, 530 nm, and 532 nm, respectively. The molecules have been tested in inverted solar cells with a maximum PCE of 1.51% obtained with the molecules containing cyclopentadithiophene.
In 2017, Zhan et al. [45] firstly reported the synthesis of a new acceptor small molecule based on a diketopyrrole core and two BODIPY lateral moieties. The molecule has a LUMO of −3.79 eV and is present in a solid-state, an intense plateau-like absorption with two maxima at 681 nm and 756 nm, which can be attributed to the delocalization of the LUMO along all the molecule. The small molecule has been tested as an acceptor in direct OSC with a PCE of 0.888%.
2.2. BODIPY Molecules as Donor Materials in Organic Solar Cells
In Table 1, the main works on BODIPY-based molecules as a donor material in the OPV devices are reported. For each research work, the year of the research publication, the peak of the absorption and the HOMO and the LUMO position of the new molecule are reported, together with the main parameters of an organic photovoltaic cell. The works are listed in chronological order and we observe that the first solar cell with a BODIPY-based active layer dates back to 2009 with a PCE of 1.7% and that just 6 years later, in 2015, a PCE of around 5% was achieved.
Table 1. Photophysical and photovoltaic characteristics of bulk heterojunction organic solar cells based on BODIPY small molecules.
Year
|
AbsFilmMax Film [nm]
|
HOMO [eV]
|
LUMO [eV]
|
Jsc
[mA/cm2]
|
Voc
[V]
|
FF [%]
|
PCE [%]
|
Reference
|
2009
|
572
|
−5.69
|
−3.66
|
4.7
|
0.866
|
42
|
1.7
|
[27]
|
2009
|
646
|
−5.56
|
−3.75
|
4.14
|
0.753
|
44
|
1.34
|
[26]
|
2010
|
649
|
-
|
-
|
7.00
|
0.75
|
38
|
2.17
|
[46]
|
2011
|
733
|
−4.71
|
−2.57
|
6.3
|
-
|
67
|
3.7
|
[47]
|
2012
|
580
|
−5.47
|
−3.48
|
8.25
|
0.988
|
39.5
|
3.22
|
[48]
|
2012
|
673
|
−4.26
|
−3.75
|
2.9
|
0.51
|
35
|
0.52
|
[49]
|
2012
|
714
|
−5.32
|
−3.86
|
14.3
|
0.7
|
47
|
4.70
|
[50]
|
2014
|
748
|
−5.00
|
−3.59
|
7
|
0.68
|
31
|
1.50
|
[51]
|
2014
|
733
|
−5.02
|
−3.64
|
6.8
|
0.67
|
34.3
|
1.56
|
[52]
|
2014
|
760
|
−5.02
|
3.37
|
8.9
|
0.51
|
34
|
1.5
|
[53]
|
2014
|
643
|
−5.31
|
−3.50
|
3.39
|
0.71
|
27
|
0.65
|
[54]
|
2015
|
680
|
−5.3
|
−2.75
|
8.42
|
0.82
|
55
|
3.76
|
[55]
|
2015
|
652 *
|
−5.62
|
−3.52
|
10.48
|
0.9
|
56
|
5.29
|
[56]
|
2015
|
774
|
−5.23
|
−3.72
|
10.32
|
0.97
|
46.5
|
4.75
|
[57]
|
2015
|
614
|
−5.48
|
−3.44
|
10.20
|
0.90
|
55
|
5.05
|
[58]
|
2015
|
514 *
|
−5.33
|
−3.86
|
3.03
|
0.81
|
24
|
0.58
|
[59]
|
2015
|
655–792
|
−5.01
|
−3.73
|
13.39
|
0.73
|
37.3
|
3.6
|
[60]
|
2015
|
761
|
−5.34
|
−3.66
|
8.17
|
00.85
|
39
|
2.70
|
[61]
|
2015
|
696
|
−5.40
|
−3.81
|
7.64
|
0.73
|
38
|
2.12
|
[62]
|
2016
|
748
|
−5.19
|
−3.60
|
6.77
|
0.78
|
41
|
2.15
|
[63]
|
2017
|
627
|
−4.93
|
−3.28
|
13.79
|
0.768
|
66.5
|
7.2
|
[64]
|
2017
|
550
|
−5.11
|
−3.65
|
11.84
|
0.73
|
53.8
|
4.61
|
[65]
|
2017
|
765
|
−5.26
|
−3.91
|
13.9
|
0.64
|
65
|
5.8
|
[66]
|
2017
|
800
|
−5.23
|
−3.87
|
13.3
|
0.73
|
63
|
6.1
|
[42]
|
2018
|
668
|
−5.06
|
−3.60
|
12.98
|
0.7
|
62
|
5.61
|
[67]
|
2018
|
720 *
|
−5.39
|
−3.74
|
12.43 **
|
0.88
|
61
|
6.67
|
[68]
|
2018
|
752 *
|
−5.36
|
−3.79
|
14.32 **
|
0.95
|
67
|
8.98
|
[68]
|
2018
|
550–640
|
−5.37
|
−3.46
|
11.46 **
|
0.915
|
63
|
6.60
|
[39]
|
2019
|
717
|
−5.00
|
−3.42
|
5.17
|
0.672
|
40.8
|
1.62
|
[69]
|
2019
|
580
|
−5.28
|
−3.61
|
10.9
|
0.83
|
60
|
5.5
|
[70]
|
2019
|
725
|
−5.16
|
−3.43
|
7.72
|
1
|
31
|
2.79
|
[71]
|
2019
|
716
|
−5.47
|
−3.76
|
10.58
|
0.769
|
56.4
|
4.58
|
[72]
|
2020
|
538
|
−5.35
|
−3.08
|
2.27
|
0.67
|
27
|
0.37
|
[73]
|
2020
|
586
672 *
|
−5.16
−4.99
|
−3.17
−3.27
|
13.56
16.24
|
0.78
0.71
|
61
66
|
6.45
7.61
|
[29]
|
2020
|
586*
|
−5.91
|
−4.09
|
0.87
|
0.45
|
21
|
1.36
|
[74]
|
* In solution: ** After Solvent Vapor Annealing (SVA).
In Table 2, only devices with PCE above 5% are analyzed in detail. The table ranges from the least to the most efficient device. The parameters reported are the absorption window, the optical energy gap and the holes mobility of the molecule, and the geometry of the device with any thermal or solvent annealing treatments.
Table 2. Photophysical and photovoltaic characteristics of bulk heterojunction organic solar cells based on BODIPY small molecules with PCE above 5%.
Molecule
[Ref.]
|
Optical Energy GAP [eV]
|
Treatment
|
PCE [%]
|
FF [%]
|
Hole
Mobility
[cm2/Vs]
|
Electron
Mobility
[cm2/Vs]
|
Abs. Band
[nm]
|
3
[58]
|
1.72
|
-
|
2.71
|
38
|
7.84 × 10−6
|
-
|
450–650
|
TA
|
3.99
|
48
|
5.34 × 10−5
|
-
|
TA + SVA
|
5.05
|
55
|
8.45 × 10−5
|
-
|
4
[56]
|
1.84
|
NO
|
3.48
|
46
|
8.5 × 10−6
|
2.34 × 10−4
|
350–700
|
Processed with pyridine 4% v/v
|
5.29
|
56
|
8.15 × 10−5
|
2.29 × 10−4
|
5b
[70]
|
1.59
|
Vacuum processed
|
5.5
|
60
|
9.2 × 10−6
|
-
|
350–1000
|
6
[67]
|
1.74
|
-
|
2.74
|
34
|
-
|
-
|
300–850
|
TA
|
4.72
|
57
|
2.09 × 10−4
|
TA +SVA
|
5.61
|
62
|
4.37 × 10−4
|
7
[66]
|
1.52
|
TA
|
5.8
|
65
|
0.8 × 10−3
|
-
|
400–800
|
8
[42]
|
1.32
|
Vacuum processed
|
6.1
|
63
|
-
|
-
|
500–900
|
9
[39]
|
1.79
|
-
|
2.54
|
39
|
5.34 × 10−5
|
2.35 × 10−4
|
400–700
|
SVA
|
6.6
|
63
|
1.13 × 10−4
|
2.45 × 10−4
|
10
[63]
|
1.65
|
-
|
5.3
|
52
|
0.9 × 10−4
|
3.9 × 10−4
|
300–800
|
TA
|
7.2
|
66.5
|
2.1 × 10−4
|
2.8 × 10−4
|
11a
[29]
|
1.78
|
-
|
3.21
|
46
|
-
|
-
|
300–600
|
SVA
|
6.45
|
61
|
8.89 × 10−5
|
2.41 × 10−4
|
11b
[29]
|
1.58
|
-
|
3.76
|
48
|
/
|
/
|
300–700
|
SVA
|
7.61
|
66
|
1.07 × 10−4
|
2.47 × 10−4
|
12a
[68]
|
1.52
|
-
|
3.32
|
41
|
3.95 × 10−5
|
2.31 × 10−4
|
400–800
|
SVA
|
6.67
|
61
|
1.10 × 10−4
|
2.36 × 10−4
|
12b
[68]
|
1.44
|
-
|
4.73
|
45
|
4.85 × 10−5
|
2.38 × 10−4
|
400–800
|
SVA
|
8.98
|
67
|
1.59 × 10−4
|
2.43 × 10−4
|
Molecule
[Ref.]
|
Optical Energy GAP [eV]
|
Treatment
|
PCE [%]
|
FF [%]
|
Hole
Mobility
[cm2/Vs]
|
Electron
Mobility
[cm2/Vs]
|
Abs. Band
[nm]
|
3
[58]
|
1.72
|
-
|
2.71
|
38
|
7.84 × 10−6
|
-
|
450–650
|
TA
|
3.99
|
48
|
5.34 × 10−5
|
-
|
TA + SVA
|
5.05
|
55
|
8.45 × 10−5
|
-
|
4
[56]
|
1.84
|
NO
|
3.48
|
46
|
8.5 × 10−6
|
2.34 × 10−4
|
350–700
|
Processed with pyridine 4% v/v
|
5.29
|
56
|
8.15 × 10−5
|
2.29 × 10−4
|
5b
[70]
|
1.59
|
Vacuum processed
|
5.5
|
60
|
9.2 × 10−6
|
-
|
350–1000
|
6
[67]
|
1.74
|
-
|
2.74
|
34
|
-
|
-
|
300–850
|
TA
|
4.72
|
57
|
2.09 × 10−4
|
TA +SVA
|
5.61
|
62
|
4.37 × 10−4
|
7
[66]
|
1.52
|
TA
|
5.8
|
65
|
0.8 × 10−3
|
-
|
400–800
|
8
[42]
|
1.32
|
Vacuum processed
|
6.1
|
63
|
-
|
-
|
500–900
|
9
[39]
|
1.79
|
-
|
2.54
|
39
|
5.34 × 10−5
|
2.35 × 10−4
|
400–700
|
SVA
|
6.6
|
63
|
1.13 × 10−4
|
2.45 × 10−4
|
10
[63]
|
1.65
|
-
|
5.3
|
52
|
0.9 × 10−4
|
3.9 × 10−4
|
300–800
|
TA
|
7.2
|
66.5
|
2.1 × 10−4
|
2.8 × 10−4
|
11a
[29]
|
1.78
|
-
|
3.21
|
46
|
-
|
-
|
300–600
|
SVA
|
6.45
|
61
|
8.89 × 10−5
|
2.41 × 10−4
|
11b
[29]
|
1.58
|
-
|
3.76
|
48
|
/
|
/
|
300–700
|
SVA
|
7.61
|
66
|
1.07 × 10−4
|
2.47 × 10−4
|
12a
[68]
|
1.52
|
-
|
3.32
|
41
|
3.95 × 10−5
|
2.31 × 10−4
|
400–800
|
SVA
|
6.67
|
61
|
1.10 × 10−4
|
2.36 × 10−4
|
12b
[68]
|
1.44
|
-
|
4.73
|
45
|
4.85 × 10−5
|
2.38 × 10−4
|
400–800
|
SVA
|
8.98
|
67
|
1.59 × 10−4
|
2.43 × 10−4
|
The highest efficiency for BODIPY small molecule-based OSC (data updated to 2020) was obtained by Bucher and co-worker [68] in 2018. The group designed and synthesized two small molecules comprised of a central BODIPY core, surrounded with two diketopyrrolopyrrole (DPP) and two porphyrin side units, differing only from each other in the aromatic bridge between BODIPY and porphyrins: in one case phenylene was used, in the other case thienyl.
From the results reported in Table 2, it is evident that in a solar cell the parameters to be taken into consideration are manifold and all closely connected to each other. Among them, two proved to be particularly important for the development of this class of materials, the absorption spectral window that must clearly be as wide as possible and the active layer morphology. The latter strongly affects the efficiency of exciton dissociation, the charges mobility, and their recombination. For this reason, it is worth to stress the thermal treatments importance and the solvent annealing. We have shown that for the same material, once TA and SVA have been made, the solar cell efficiency can often be doubled.
References
- Semiconducting Polymers: Chemistry, Physics and Engineering, 2nd Edition, Two-Volume Set | Wiley Available online: https://www.wiley.com/en-us/Semiconducting+Polymers%3A+Chemistry%2C+Physics+and+Engineering%2C+2nd+Edition%2C+Two+Volume+Set-p-9783527312719 (accessed on 24 November 2020).
- Ostroverkhova, O. Organic Optoelectronic Materials: Mechanisms and Applications. Rev. 2016, 116, 13279–13412, doi:10.1021/acs.chemrev.6b00127.
- Forrest, S.R.; Thompson, M.E. Introduction: Organic Electronics and Optoelectronics. Rev. 2007, 107, 923–925, doi:10.1021/cr0501590.
- Zvezdin, A.; Mauro, E.D.; Rho, D.; Santato, C.; Khalil, M. En Route toward Sustainable Organic Electronics. MRS Energy Sustain. 2020, 7, doi:10.1557/mre.2020.16.
- Giovanella, U.; Betti, P.; Bolognesi, A.; Destri, S.; Melucci, M.; Pasini, M.; Porzio, W.; Botta, C. Core-Type Polyfluorene-Based Copolymers for Low-Cost Light-Emitting Technologies. Electron. 2010, 11, 2012–2018, doi:10.1016/j.orgel.2010.09.009.
- Vohra, V.; Galeotti, F.; Giovanella, U.; Mróz, W.; Pasini, M.; Botta, C. Nanostructured Light-Emitting Polymer Thin Films and Devices Fabricated by the Environment-Friendly Push-Coating Technique. ACS Appl. Mater. Interfaces 2018, 10, 11794–11800, doi:10.1021/acsami.8b00137.
- Irimia-Vladu, M. “Green” Electronics: Biodegradable and Biocompatible Materials and Devices for Sustainable Future. Soc. Rev. 2014, 43, 588–610, doi:10.1039/C3CS60235D.
- Treibs, A.; Kreuzer, F.-H. Difluorboryl-Komplexe von Di- Und Tripyrrylmethenen. Justus Liebigs Annalen Chem. 1968, 718, 208–223, doi:10.1002/jlac.19687180119.
- Banuelos, J. BODIPY dye, the most versatile fluorophore ever? Rec., 2016 16, 335-348, doi:10.1002/tcr.201500238.
- Loudet, A.; Burgess, K. BODIPY Dyes and Their Derivatives: Syntheses and Spectroscopic Properties. Rev. 2007, 107, 4891–4932, doi:10.1021/cr078381n.
- Squeo, B.M.; Gregoriou, V.G.; Avgeropoulos, A.; Baysec, S.; Allard, S.; Scherf, U.; Chochos, C.L. BODIPY-Based Polymeric Dyes as Emerging Horizon Materials for Biological Sensing and Organic Electronic Applications. Polym. Sci. 2017, 71, 26–52, doi:10.1016/j.progpolymsci.2017.02.003.
- Squeo, B.M.; Pasini, M. BODIPY Platform: A Tunable Tool for Green to NIR OLEDs. Chem. 2020, 32, 56–70, doi:10.1080/10610278.2019.1691727.
- Menges, N. Computational Study on Aromaticity and Resonance Structures of Substituted BODIPY Derivatives. Theor. Chem. 2015, 1068, 117–122, doi:10.1016/j.comptc.2015.06.031.
- Ulrich, G.; Ziessel, R.; Harriman, A. The Chemistry of Fluorescent Bodipy Dyes: Versatility Unsurpassed. Chem. Int. Ed. 2008, 47, 1184–1201, doi:10.1002/anie.200702070.
- Poddar, M.; Misra, R. Recent Advances of BODIPY Based Derivatives for Optoelectronic Applications. Chem. Rev. 2020, 421, 213462, doi:10.1016/j.ccr.2020.213462.
- Singh, A.; Yip, W.-T.; Halterman, R.L. Fluorescence-On Response via CB7 Binding to Viologen–Dye Pseudorotaxanes. Lett. 2012, 14, 4046–4049, doi:10.1021/ol300963c.
- Frath, D.; Yarnell, J.E.; Ulrich, G.; Castellano, F.N.; Ziessel, R. Ultrafast Photoinduced Electron Transfer in Viologen-Linked BODIPY Dyes. ChemPhysChem 2013, 14, 3348–3354, doi:10.1002/cphc.201300547.
- Tao, J.; Sun, D.; Sun, L.; Li, Z.; Fu, B.; Liu, J.; Zhang, L.; Wang, S.; Fang, Y.; Xu, H. Tuning the Photo-Physical Properties of BODIPY Dyes: Effects of 1, 3, 5, 7- Substitution on Their Optical and Electrochemical Behaviours. Dyes Pigment. 2019, 168, 166–174, doi:10.1016/j.dyepig.2019.04.054.
- Llano, R.S.; Zaballa, E.A.; Bañuelos, J.; Durán, C.F.A.G.; Vázquez, J.L.B.; Cabrera, E.P.; Arbeloa, I.L. Tailoring the Photophysical Signatures of BODIPY Dyes: Toward Fluorescence Standards across the Visible Spectral Region. Photophys. Fundam. Appl. 2018, doi:10.5772/intechopen.74848.
- Littler, B.J.; Miller, M.A.; Hung, C.-H.; Wagner, R.W.; O’Shea, D.F.; Boyle, P.D.; Lindsey, J.S. Refined Synthesis of 5-Substituted Dipyrromethanes. Org. Chem. 1999, 64, 1391–1396, doi:10.1021/jo982015+.
- Shimizu, S.; Iino, T.; Araki, Y.; Kobayashi, N. Pyrrolopyrrole Aza-BODIPY Analogues: A Facile Synthesis and Intense Fluorescence. Commun. 2013, 49, 1621–1623, doi:10.1039/C3CC38452G.
- Adarsh, N.; Shanmugasundaram, M.; Avirah, R.R.; Ramaiah, D. Aza-BODIPY Derivatives: Enhanced Quantum Yields of Triplet Excited States and the Generation of Singlet Oxygen and Their Role as Facile Sustainable Photooxygenation Catalysts. Chem. A Eur. J. 2012, 18, 12655–12662, doi:10.1002/chem.201202438.
- Ho, D.; Ozdemir, R.; Kim, H.; Earmme, T.; Usta, H.; Kim, C. BODIPY-Based Semiconducting Materials for Organic Bulk Heterojunction Photovoltaics and Thin-Film Transistors. ChemPlusChem 2019, 84, 18–37, doi:10.1002/cplu.201800543.
- Wanwong, S., Sangkhun, W., Kumnorkaew, P., & Wootthikanokkhan, J. Improved Performance of Ternary Solar Cells by Using BODIPY Triads. Materials, 2020, 13, 2723, doi: 10.3390/ma13122723.
- Boens, N.; Verbelen, B.; Dehaen, W. Postfunctionalization of the BODIPY Core: Synthesis and Spectroscopy. J. Org. Chem. 2015, 2015, 6577–6595, doi:10.1002/ejoc.201500682.
- Rousseau, T.; Cravino, A.; Bura, T.; Ulrich, G.; Ziessel, R.; Roncali, J. BODIPY Derivatives as Donor Materials for Bulk Heterojunction Solar Cells. Commun. 2009, 1673, doi:10.1039/b822770e.
- Rousseau, T.; Cravino, A.; Bura, T.; Ulrich, G.; Ziessel, R.; Roncali, J. Multi-Donor Molecular Bulk Heterojunction Solar Cells: Improving Conversion Efficiency by Synergistic Dye Combinations. Mater. Chem. 2009, 19, 2298–2300, doi:10.1039/B903189H.
- Liu, B.; Ma, Z.; Xu, Y.; Guo, Y.; Yang, F.; Xia, D.; Li, C.; Tang, Z.; Li, W. Non-Fullerene Organic Solar Cells Based on a BODIPY-Polymer as Electron Donor with High Photocurrent. Mater. Chem. C 2020, 8, 2232–2237, doi:10.1039/C9TC05480D.
- Yang, J.; Devillers, C.H.; Fleurat-Lessard, P.; Jiang, H.; Wang, S.; Gros, C.P.; Gupta, G.; Sharma, G.D.; Xu, H. Carbazole-Based Green and Blue-BODIPY Dyads and Triads as Donors for Bulk Heterojunction Organic Solar Cells. Dalton Trans. 2020, 49, 5606–5617, doi:10.1039/D0DT00637H.
- Liu, Q.; Jiang, Y.; Jin, K.; Qin, J.; Xu, J.; Li, W.; Xiong, J.; Liu, J.; Xiao, Z.; Sun, K.; et al. 18% Efficiency Organic Solar Cells. Bull. 2020, 65, 272–275, doi:10.1016/j.scib.2020.01.001.
- Clarke, T.M.; Durrant, J.R. Charge Photogeneration in Organic Solar Cells. Rev. 2010, 110, 6736–6767, doi:10.1021/cr900271s.
- Achieving a High Fill Factor for Organic Solar Cells—Journal of Materials Chemistry A (RSC Publishing). Available online: https://pubs.rsc.org/en/content/articlelanding/2016/ta/c6ta00126b#!divAbstract (accessed on 24 November 2020).
- Squeo, B.M.; Gregoriou, V.G.; Han, Y.; Palma-Cando, A.; Allard, S.; Serpetzoglou, E.; Konidakis, I.; Stratakis, E.; Avgeropoulos, A.; Heeney, M.; et al. α,β-Unsubstituted Meso -Positioning Thienyl BODIPY: A Promising Electron Deficient Building Block for the Development of near Infrared (NIR) p-Type Donor–Acceptor (D–A) Conjugated Polymers. Mater. Chem. C 2018, 6, 4030–4040, doi:10.1039/C7TC05900K.
- Zampetti, A.; Minotto, A.; Squeo, B.M.; Gregoriou, V.G.; Allard, S.; Scherf, U.; Chochos, C.L.; Cacialli, F. Highly Efficient Solid-State Near-Infrared Organic Light-Emitting Diodes Incorporating A-D-A Dyes Based on α,β -Unsubstituted “BODIPY” Moieties. Rep. 2017, 7, 1611, doi:10.1038/s41598-017-01785-2.
- Chochos, C.L.; Drakopoulou, S.; Katsouras, A.; Squeo, B.M.; Sprau, C.; Colsmann, A.; Gregoriou, V.G.; Cando, A.-P.; Allard, S.; Scherf, U.; et al. Beyond Donor–Acceptor (D–A) Approach: Structure–Optoelectronic Properties—Organic Photovoltaic Performance Correlation in New D–A1–D–A2 Low-Bandgap Conjugated Polymers. Rapid Commun. 2017, 38, 1600720, doi:10.1002/marc.201600720.
- Mahesh, K.; Karpagam, S.; Pandian, K. How to Design Donor–Acceptor Based Heterocyclic Conjugated Polymers for Applications from Organic Electronics to Sensors. Top. Chem. 2019, 377, 12, doi:10.1007/s41061-019-0237-4.
- Salzner, U. Effect of Donor–Acceptor Substitution on Optoelectronic Properties of Conducting Organic Polymers. Chem. Theory Comput. 2014, 10, 4921–4937, doi:10.1021/ct500816c.
- Porzio, W.; Destri, S.; Pasini, M.; Giovanella, U.; Ragazzi, M.; Scavia, G.; Kotowski, D.; Zotti, G.; Vercelli, B. Synthesis and Characterisation of Fluorenone–Thiophene-Based Donor–Acceptor Oligomers: Role of Moiety Sequence upon Packing and Electronic Properties. New J. Chem. 2010, 34, 1961–1973, doi:10.1039/C0NJ00045K.
- Mishra, R.; Basumatary, B.; Singhal, R.; Sharma, G.D.; Sankar, J. Corrole-BODIPY Dyad as Small-Molecule Donor for Bulk Heterojunction Solar Cells. ACS Appl. Mater. Interfaces 2018, 10, 31462–31471, doi:10.1021/acsami.8b08519.
- Squeo, B.M.; Carulli, F.; Lassi, E.; Galeotti, F.; Giovanella, U.; Luzzati, S.; Pasini, M. Benzothiadiazole-Based Conjugated Polyelectrolytes for Interfacial Engineering in Optoelectronic Devices. Pure Appl. Chem. 2019, 91, 477–488, doi:10.1515/pac-2018-0925.
- Carulli, F.; Scavia, G.; Lassi, E.; Pasini, M.; Galeotti, F.; Brovelli, S.; Giovanella, U.; Luzzati, S. A Bifunctional Conjugated Polyelectrolyte for the Interfacial Engineering of Polymer Solar Cells. Colloid Interface Sci. 2019, 538, 611–619, doi:10.1016/j.jcis.2018.12.027.
- Li, T.; Meyer, T.; Ma, Z.; Benduhn, J.; Körner, C.; Zeika, O.; Vandewal, K.; Leo, K. Small Molecule Near-Infrared Boron Dipyrromethene Donors for Organic Tandem Solar Cells. Am. Chem. Soc. 2017, 139, 13636–13639, doi:10.1021/jacs.7b07887.
- Lin, Y.; Li, Y.; Zhan, X. Small Molecule Semiconductors for High-Efficiency Organic Photovoltaics. Soc. Rev. 2012, 41, 4245–4272, doi:10.1039/C2CS15313K.
- Poe, A.M.; Pelle, A.M.D.; Subrahmanyam, A.V.; White, W.; Wantz, G.; Thayumanavan, S. Small Molecule BODIPY Dyes as Non-Fullerene Acceptors in Bulk Heterojunction Organic Photovoltaics. Commun. 2014, 50, 2913–2915, doi:10.1039/C3CC49648A.
- Liu, W.; Yao, J.; Zhan, C. A Novel BODIPY‐Based Low‐Band‐Gap Small‐Molecule Acceptor for Efficient Non‐fullerene Polymer Solar Cells. J. Chem. 2017, 35, 1813–1823, doi:10.1002/cjoc.201700542.
- Rousseau, T.; Cravino, A.; Ripaud, E.; Leriche, P.; Rihn, S.; Nicola, A.D.; Ziessel, R.; Roncali, J. A Tailored Hybrid BODIPY–Oligothiophene Donor for Molecular Bulk Heterojunction Solar Cells with Improved Performances. Commun. 2010, 46, 5082–5084, doi:10.1039/C0CC01144D.
- Kubo, Y.; Watanabe, K.; Nishiyabu, R.; Hata, R.; Murakami, A.; Shoda, T.; Ota, H. Near-Infrared Absorbing Boron-Dibenzopyrromethenes That Serve As Light-Harvesting Sensitizers for Polymeric Solar Cells. Lett. 2011, 13, 4574–4577, doi:10.1021/ol201770g.
- Lin, H.-Y.; Huang, W.-C.; Chen, Y.-C.; Chou, H.-H.; Hsu, C.-Y.; Lin, J.T.; Lin, H.-W. BODIPY Dyes with β-Conjugation and Their Applications for High-Efficiency Inverted Small Molecule Solar Cells. Commun. 2012, 48, 8913, doi:10.1039/c2cc34286c.
- Hayashi, Y.; Obata, N.; Tamaru, M.; Yamaguchi, S.; Matsuo, Y.; Saeki, A.; Seki, S.; Kureishi, Y.; Saito, S.; Yamaguchi, S.; et al. Facile Synthesis of Biphenyl-Fused BODIPY and Its Property. Lett. 2012, 14, 866–869, doi:10.1021/ol2033916.
- Bura, T.; Leclerc, N.; Fall, S.; Lévêque, P.; Heiser, T.; Retailleau, P.; Rihn, S.; Mirloup, A.; Ziessel, R. High-Performance Solution-Processed Solar Cells and Ambipolar Behavior in Organic Field-Effect Transistors with Thienyl-BODIPY Scaffoldings. Am. Chem. Soc. 2012, 134, 17404–17407, doi:10.1021/ja3072513.
- Kolemen, S.; Cakmak, Y.; Ozdemir, T.; Erten-Ela, S.; Buyuktemiz, M.; Dede, Y.; Akkaya, E.U. Design and Characterization of Bodipy Derivatives for Bulk Heterojunction Solar Cells. Tetrahedron 2014, 70, 6229–6234, doi:10.1016/j.tet.2014.03.049.
- Liu, W.; Tang, A.; Chen, J.; Wu, Y.; Zhan, C.; Yao, J. Photocurrent Enhancement of BODIPY-Based Solution-Processed Small-Molecule Solar Cells by Dimerization via the Meso Position. ACS Appl. Mater. Interfaces 2014, 6, 22496–22505, doi:10.1021/am506585u.
- Sutter, A.; Retailleau, P.; Huang, W.-C.; Lin, H.-W.; Ziessel, R. Photovoltaic Performance of Novel Push–Pull–Push Thienyl–Bodipy Dyes in Solution-Processed BHJ-Solar Cells. New J. Chem. 2014, 38, 1701–1710, doi:10.1039/C3NJ01436C.
- Cortizo-Lacalle, D.; Howells, C.T.; Pandey, U.K.; Cameron, J.; Findlay, N.J.; Inigo, A.R.; Tuttle, T.; Skabara, P.J.; Samuel, I.D.W. Solution Processable Diketopyrrolopyrrole (DPP) Cored Small Molecules with BODIPY End Groups as Novel Donors for Organic Solar Cells. Beilstein J. Chem. 2014, 10, 2683–2695, doi:10.3762/bjoc.10.283.
- Chen, J.J.; Conron, S.M.; Erwin, P.; Dimitriou, M.; McAlahney, K.; Thompson, M.E. High-Efficiency BODIPY-Based Organic Photovoltaics. ACS Appl. Mater. Interfaces 2015, 7, 662–669, doi:10.1021/am506874k.
- Sharma, G.D.; Siddiqui, S.A.; Nikiforou, A.; Zervaki, G.E.; Georgakaki, I.; Ladomenou, K.; Coutsolelos, A.G. A Mono(Carboxy)Porphyrin-Triazine-(Bodipy) 2 Triad as a Donor for Bulk Heterojunction Organic Solar Cells. Mater. Chem. C 2015, 3, 6209–6217, doi:10.1039/C5TC01076D.
- Xiao, L.; Wang, H.; Gao, K.; Li, L.; Liu, C.; Peng, X.; Wong, W.-Y.; Wong, W.-K.; Zhu, X. A-D-A Type Small Molecules Based on Boron Dipyrromethene for Solution-Processed Organic Solar Cells. Asian J. 2015, 10, 1513–1518, doi:10.1002/asia.201500382.
- Jadhav, T.; Misra, R.; Biswas, S.; Sharma, G.D. Bulk Heterojunction Organic Solar Cells Based on Carbazole–BODIPY Conjugate Small Molecules as Donors with High Open Circuit Voltage. Chem. Chem. Phys. 2015, 17, 26580–26588, doi:10.1039/C5CP04807A.
- Zou, L.; Guan, S.; Li, L.; Zhao, L. Dipyrrin-Based Complexes for Solution-Processed Organic Solar Cells. Res. Chin. Univ. 2015, 31, 801–808, doi:10.1007/s40242-015-5140-0.
- Liu, W.; Yao, J.; Zhan, C. Performance Enhancement of BODIPY Dimer-Based Small-Molecule Solar Cells Using a Visible-Photon-Capturing Diketopyrrolopyrrole π-Bridge. RSC Adv. 2015, 5, 74238–74241, doi:10.1039/C5RA16725F.
- Zhang, X.; Zhang, Y.; Chen, L.; Xiao, Y. Star-Shaped Carbazole-Based BODIPY Derivatives with Improved Hole Transportation and near-Infrared Absorption for Small-Molecule Organic Solar Cells with High Open-Circuit Voltages. RSC Adv. 2015, 5, 32283–32289, doi:10.1039/C5RA02414E.
- Liao, J.; Xu, Y.; Zhao, H.; Wang, Y.; Zhang, W.; Peng, F.; Xie, S.; Yang, X. Synthesis, Optical, Electrochemical Properties and Photovoltaic Performance of Novel Panchromatic Meso-Thiophene BODIPY Dyes with a Variety of Electron-Donating Groups at the 3,5-Positions. RSC Adv. 2015, 5, 86453–86462, doi:10.1039/C5RA15389A.
- Liao, J.; Zhao, H.; Xu, Y.; Cai, Z.; Peng, Z.; Zhang, W.; Zhou, W.; Li, B.; Zong, Q.; Yang, X. Novel D–A–D Type Dyes Based on BODIPY Platform for Solution Processed Organic Solar Cells. Dyes Pigment. 2016, 128, 131–140, doi:10.1016/j.dyepig.2016.01.020.
- Rao, R.S.; Bagui, A.; Rao, G.H.; Gupta, V.; Singh, S.P. Achieving the Highest Efficiency Using a BODIPY Core Decorated with Dithiafulvalene Wings for Small Molecule Based Solution-Processed Organic Solar Cells. Commun. 2017, 53, 6953–6956, doi:10.1039/C7CC03363J.
- Liao, J.; Xu, Y.; Zhao, H.; Zong, Q.; Fang, Y. Novel A-D-A Type Small Molecules with β-Alkynylated BODIPY Flanks for Bulk Heterojunction Solar Cells. Electron. 2017, 49, 321–333, doi:10.1016/j.orgel.2017.06.054.
- Bulut, I.; Huaulmé, Q.; Mirloup, A.; Chávez, P.; Fall, S.; Hébraud, A.; Méry, S.; Heinrich, B.; Heiser, T.; Lévêque, P.; et al. Rational Engineering of BODIPY‐Bridged Trisindole Derivatives for Solar Cell Applications. ChemSusChem 2017, 10, 1878–1882, doi:10.1002/cssc.201700465.
- Liao, J.; Zhao, H.; Cai, Z.; Xu, Y.; Qin, F.G.F.; Zong, Q.; Peng, F.; Fang, Y. BODIPY-Based Panchromatic Chromophore for Efficient Organic Solar Cell. Electron. 2018, 61, 215–222, doi:10.1016/j.orgel.2018.05.049.
- Bucher, L.; Desbois, N.; Koukaras, E.N.; Devillers, C.H.; Biswas, S.; Sharma, G.D.; Gros, C.P. BODIPY–Diketopyrrolopyrrole–Porphyrin Conjugate Small Molecules for Use in Bulk Heterojunction Solar Cells. Mater. Chem. A 2018, 6, 8449–8461, doi:10.1039/C8TA01291A.
- Marques dos Santos, J.; Jagadamma, L.K.; Latif, N.M.; Ruseckas, A.; Samuel, I.D.W.; Cooke, G. BODIPY Derivatives with near Infra-Red Absorption as Small Molecule Donors for Bulk Heterojunction Solar Cells. RSC Adv. 2019, 9, 15410–15423, doi:10.1039/C9RA01750J.
- Li, T.; Benduhn, J.; Qiao, Z.; Liu, Y.; Li, Y.; Shivhare, R.; Jaiser, F.; Wang, P.; Ma, J.; Zeika, O.; et al. Effect of H- and J-Aggregation on the Photophysical and Voltage Loss of Boron Dipyrromethene Small Molecules in Vacuum-Deposited Organic Solar Cells. Phys. Chem. Lett. 2019, 10, 2684–2691, doi:10.1021/acs.jpclett.9b01222.
- Aguiar, A.; Farinhas, J.; da Silva, W.; Ghica, M.E.; Brett, C.M.A.; Morgado, J.; Sobral, A.J.F.N. Synthesis, Characterization and Application of Meso-Substituted Fluorinated Boron Dipyrromethenes (BODIPYs) with Different Styryl Groups in Organic Photovoltaic Cells. Dyes Pigment. 2019, 168, 103–110, doi:10.1016/j.dyepig.2019.04.031.
- Thumuganti, G.; Gupta, V.; Singh, S.P. New Dithienosilole- and Dithienogermole-Based BODIPY for Solar Cell Applications. New J. Chem. 2019, 43, 8735–8740, doi:10.1039/C9NJ00550A.
- Aguiar, A.; Farinhas, J.; da Silva, W.; Susano, M.; Silva, M.R.; Alcácer, L.; Kumar, S.; Brett, C.M.A.; Morgado, J.; Sobral, A.J.F.N. Simple BODIPY Dyes as Suitable Electron-Donors for Organic Bulk Heterojunction Photovoltaic Cells. Dyes Pigment. 2020, 172, 107842, doi:10.1016/j.dyepig.2019.107842.
- Ivaniuk, K.; Pidluzhna, А.; Stakhira, P.; Baryshnikov, G.V.; Kovtun, Y.P.; Hotra, Z.; Minaev, B.F.; Ågren, H. BODIPY-Core 1,7-Diphenyl-Substituted Derivatives for Photovoltaics and OLED Applications. Dyes Pigment. 2020, 175, 108123, doi:10.1016/j.dyepig.2019.108123.