2. The Functionalization of Calix[4]arene
The different nature of the two rims available on the calixarene skeleton allows for selective functionalization on the upper rim (functionalization of the para carbon of the aromatic nucleus) or on the lower rim (functionalization of the phenolic hydroxyl) (
Figure 2). Concerning the upper rim, it can be modified by aromatic nucleophilic substitution, via halogenation
[19][20][73,74], nitration
[21][22][75,76], sulfonation
[23][24][25][59,61,77], acylation
[26][27][78,79], or other means. In addition, on the native,
para-tert-butylated calixarene, it is possible to completely or selectively detertiobutylate by first passing through the protection of the OH of the phenolic rings on which it is desired to retain a
para-substitution
[28][80]. On the other hand, the lower rim allows, via the reactive hydroxyl groups, the introduction of various arms by formation of ether or ester bonds
[29][81].
In the case of calix[4]arene, the lower rim has four hydroxyl groups engaged in a network of intramolecular hydrogen bonds favoring the conical conformation (
Figure 2). These strong hydrogen bonds lead to different pKa values for the hydroxyl groups involved in this ring
[30][82]. This allows the establishment of various regio- and stereo-selective functionalization procedures on the lower crown. It has been shown that by implementing a carrier ion effect (“template effect”) relating to the nature and strength of the base used, it is possible to stereospecifically incorporate different substituents on the lower part of the calixarene. It is thus possible to substitute in the lower rim a calix[4]arene with 1 to 4 groups and, moreover, for bis-functionalization, a possibility of regioselectivity in the
1,2-adjacent or
1,3-alternated position
[31][32][33][34][35][36][37][38][39][40][83,84,85,86,87,88,89,90,91,92].
3. Cytotoxicity of Calix[n]arenes
To develop biomedical applications, research into strong, possibly deleterious, interactions between the design molecular object and the biological target (cell, protein…) must examine the toxicity of various biological entities that these objects could potentially encounter before reaching their target. This is the case for the calixarene derivatives, more particularly the water-soluble ones, with the increase in interest which devoted to them for various biological applications and potential clinical studies. Relatively rare some twenty years ago, these studies are beginning to appear, and almost all converge towards the fact that this structure seems to have no toxicity or immunogenicity
[41][42][93,94].
For example, the
para-sulfonatocalix[
n]arenes (
Figure 3), widely used for numerous biomedical applications (anti-viral, anti-thrombotic, protein complexation, anti-coagulant…) exhibit an innocuous character with regard to studies conducted until this date
[43][35]. It thus has very low toxicity on red blood cells
[44][95], no toxicity against neutrophils involved in immune response
[45][97], against the human ovarian carcinoma cell line A2780 and its equivalent cisplatin-resistant cell line A2780cis
[46][99], as well as against L-132 epithelial cell line
[47][100] or HL50 cells (i.e., human leukemia cells)
[48].[101]
Figure 3.
Structure of para-sulfonated calix[4, 6, or 8]arenes [49]. -sulfonated calix[4, 6, or 8]arenes [95].
4. Antibacterial Activities of Calix[n]arenes
The calixarenic structure is endowed with great versatility, and this is why it is used in a wide variety of fields. The most interesting features are the multiple functionalizable positions and the access to multivalence. Multivalency is, for example, a key element in generating the strongest and most specific interactions possible
[50][51][52][104,105,106]. This last is widely used for new drug research, for example: simple use as a carrier of multiple active pharmaceutical ingredients (API) (identical or different) in the case of development of prodrugs; the genesis of an environment conducive to the complexation of metals in the search for compounds capable of disrupting the activity of metalloenzymes or the formation of toxic metal complexes; and research on therapeutic activities related to strong interactions with proteins, enzymes, or nucleic acids
[53][107].
As the versatility of calixarenes is very wide, it is possible to optimize the design of a multivalent ligand to improve the desired activity. For example, the length of the bond between the calixarene and the group of interest will often be a very important and critical point: a calix[4]arene could lead to spatial constraints and greater interactions than a calix[8]arene; however, this last, more fluxional, could allow more adaptability face to a given target. Thus, using this central skeleton, researchers can very precisely tune the nature, number, distance, or orientation in space of any type of functionality in such a way as to best match a biological target as finely as possible.
4.1. Intrinsically Active Calixarenes
Since the 1950s, researchers have sought to functionalize calixarene crowns with various types of functional groups, sometimes known to already possess a biological activity. The objective is often to take advantage of the multivalence provided by the macrocycle and to envisage a synergy of action. It appears very clearly in certain studies, by comparison with the constitutive monomers of calixarene, that the latter allows a marked improvement in the activities sought, fully justifying its use.
The first direct antibacterial activity of calixarene was reported in 1955 by Cornforth et al.
[54][55][108,109]. An important study was carried out on macrocyclon (
Figure 4), alternatively named HOC 12.5 EO, constituted from macrocyclic phenol (calix[8]arene), which for brevity is termed HOC, and which was prepared from tert-octylphenol and formaldehyde by a modified Zinke–Ziegler procedure, and by reacting under basic conditions with ethylene oxide (EO). The four resulting polyoxyethylene ether chains have an average chain length of 11–12.5 units (HOC 12.5 EO)
[55][56].[109,110]
Figure 4. Examples of intrinsically active calixarenes: macrocyclon, peptidocalix[4]arenes, guanidinium and ammonium calix[4]arene respectively from left to right
Subsequently, very good results appeared, for example, for peptidocalix[4]arenes (Figure 4) with good activity against MRSA (methicillin-resistant S. aureus) (4 to 8 µg/mL) [57][58][59][115, 116 ,117]. From 2006, calix[4]arene positively charged by the introduction of guanidinium functions showed excellent activities on reference bacterial strains (Figure 4). These last introduced on the upper rim displays a significant antibacterial activity (MIC <8 µg/mL) against the bacterial reference strains E. coli, S. aureus, E. faecalis and P. aeruginosa [18][60][72,103], but also on the clinical isolates penicillinase producing E. coli, methicillin-resistant S. aureus, vancomycin-resistant E. faecium, vancomycin- and teicoplanin-resistant E. faecalis, and P. aeruginosa overexpressing efflux pumps [61][120]. This guanidinium calixarene and its derivatives also have very good activity against a particular strain: Mycobacterium tuberculosis [60][62][103,132].
Another examples concern a water-soluble macromolecule based on calix[4]arene and morpholine units [63][133], appears to have an excellent activity profile with the inhibitions of growth were measured at 4 µg/mL against gram-positive and gram-negative species, but also a serie of azo compounds [64][141] showing very good activities through CMI measurements on five Gram-positive bacterial strains (B. subtilis, S. aureus, methicillin-resistant S. aureus (MRSA), S. epidermidis, and E. faecalis) (<10 µg/mL).
Finally, a recent study confirms the interest of calixarenes carrying cationic functions but also of the impact of conformation [65][147] (Figure 4) as described in a previous study [60][103]. Indeed, the ammonium derivatives concerned exhibit activities close to 1 µg/ml against Gram-positive and Gram-negative strains.
4.2. Molecular Carrier/Drug Delivery
Widely used in the search for new active compounds, the principle of carriers is part of the strategies for the development of new active ingredients. In the case of calixarenes, the authors generally seek to use the platform in order to modify the bioavailability (increase the hydro- or the lipo-solubility for examples), increasing the activity of the active ingredient by generating a greater density of antibiotic per unit surface (cluster effect), and this via strong covalent bonds or physiologically hydrolyzable bonds.
These studies propose, for example, the introduction of penicillin via amide or ester functions, which should release the biologically active free acid and amine after hydrolysis by esterases [66][67][149,150] (Figure 5). The same strategy allows the synthesis of bis-quinolone derivatives [68][69][151,152] and a study of the anti-bacterial activity against Gram-positive reference ATCC strains, S. aureus and E. faecalis, as well as against two Gram-negative reference strains E. coli and P. aeruginosa. Designed as hydrophobic compounds, these structures are not really easy for in vitro standard evaluation as antimicrobial agents. Thus, an additional study proposes the development of water-soluble compounds via the introduction of ammonium functions [70][156]. Other researchers have also obtained promising results through the synthesis and biological evaluation of penicillins V and X clustered by a calixarene scaffold [71][157] and the corresponding cephalosporine derivatives [72][158] (Figure 5). The impact of cyclotetramerization was demonstrated by a notable increase in activity on all penicillin cyclotetramer strains versus monomers.

Figure 5.
Examples of molecular carriers/drug delivery: penicillin (left) or cephalosporine (right)
4.3. Complexes
The ion complexation by calixarene derivatives has been the subject of numerous studies and is widely used in the separation and extraction of metal ions, sensors material or catalysts. They are good carriers for cations due to their cyclic structure with different sizes of cavities and the many possibilities of functionalization. These functional arms, endowed of electron donating groups, coupled to the organizational capacity of calixarene, allow generating a conducive environment to the ion’s reception.
In this sense, researchers have developed complex structures of mononuclear copper [73][74][163,164] (Figure 6) for their antibacterial activities against Gram-positive (B. subtilis, B. cereus, E. faecalis (clinic sample), S. aureus) and Gram-negative bacteria (E. coli, E. coli, P. aeruginosa, Proteus vulgaris) or binuclear [75][166] (Figure 6) active against Gram-positive S. albus and Gram-negative E. coli. On the other hand, the thiosemicarbazide moieties and their thiosemicarbazone derivatives known for their ability to coordinate with transition metals by bonding with sulfur and nitrogen atoms [167,168] allowed the development of cobalt (II), nickel (II), copper (II), or zinc (II) complexes.
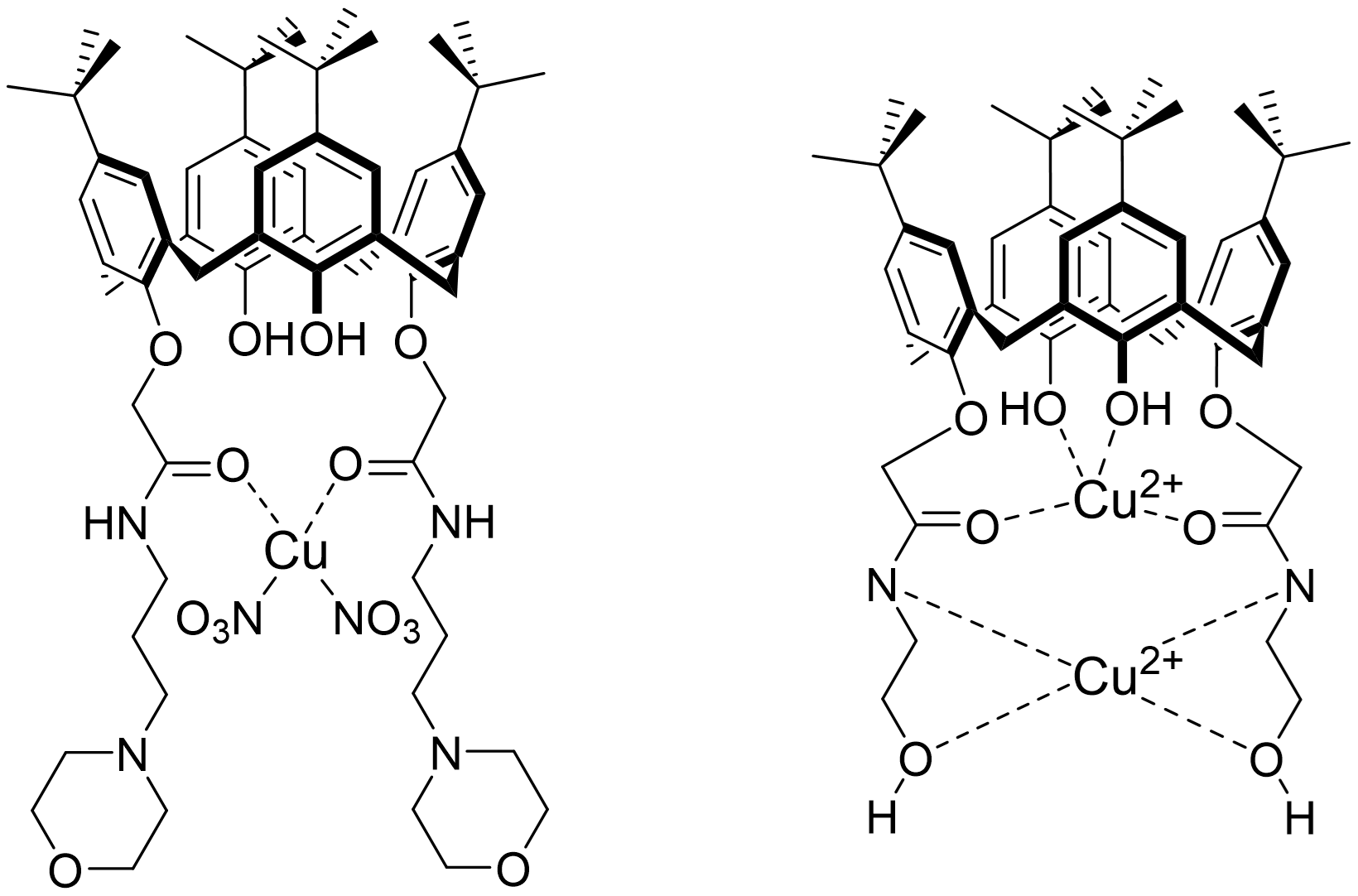
Figure 6. Examples of mononuclear copper (left) and binuclear copper (right) complexes
Examples of mononuclear copper (left) and binuclear copper (right) complexes
5. Conclusions
Calixarenes have already come a long way since A. von Baeyer in 1872 and have found their place in many fields of study and applications. Thanks to their great flexibility (functionalization and spatial organization), the Man of Art knew how to modulate them in order to confer remarkable activities. The crucial lack of new antibiotics associated with the alarming increase in bacterial resistance requires a major effort in the search for new therapeutic strategies, and it appears that the calixarene structure finds its place there. The researchers have thus designed many structures carrying intrinsic activity, activity linked to the possibility of releasing active principles or to the formation of metal ion complexes. From average to very good, these activities make it possible to determine structure–activity relationships and to direct researchers towards structures with higher potential. For example, many works report the development of cationic calixarenes with an original mechanism of action based on electrostatic interactions with the bacterial cell wall. The very interesting results highlighted could lead to improvement in future work. The exceptional modularity (regioselective polyfunctionalization and multiple conformations) of the calixarene structure can still allow researchers to shape their structures in order to further increase the affinities with the targets of action sought, to broaden the spectrum of action, or, on the contrary, to target more specific strains.