For jet fuel, the aviation industry primarily uses two types of fuel: aviation gasoline and aviation kerosene. Aviation gasoline, also known as AVGAS, is derived from the distillation of crude oil in the range between 30 and 170 °C. AVGAS is normally used in small aircraft, such as private aviation, agricultural, and pilot training. Aviation kerosene is also a petroleum distillation product obtained in the range between 150 and 300 °C. This fuel is more suitable in aircraft engines for energy generation through combustion, used in many commercial and military aircraft.
1. Introduction
The rapid growth of the aviation industry, in recent decades, as well as airport expansions, has exacerbated aviation’s role in climate change and environmental protection
[1]. Annually, about 3.1 billion people and 51.7 million tonnes of cargo are operated by 1000 commercial airlines, using approximately 15,000 aircrafts
[2]. This has led to an annual 3.5–4.5% increase in hydrocarbon fuel consumption
[3]. To meet the 2050 carbon reduction targets, improving aircraft fuel carbon-neutrality and emissions are critical
[4].
Aircraft engine exhaust comprises up to 8% CO
2 (approximately 900 Mt CO
2) water vapour, NOx (0.03%), UHC, CO, and SO
2 [5]. In 2010, the share of total global GHG emissions from the air transport sector was 4% and 6%, respectively, for domestic and international flights
[6]. Although these numbers seem relatively low because the emissions are at high altitude, the NOx generated in the upper atmosphere leads to ozone production and is, therefore, likely to be more damaging than these figures initially suggest. Hence, to reduce the aviation carbon emission with sustainable energy efficiency, sustainable aviation fuel (SAF), such as algal-based biofuel, will be the key for this industry
[7]. Such an alternative could potentially reduce the life cycle of CO
2 emissions by up to 80% by 2050, compared to 2005
[6]. Fuel sustainability criteria must include: (1) suitability for existing aircraft engines, (2) no modifications in infrastructure, (3) must produce low life cycle carbon emissions, as compared to fossil-based jet fuel, (4) food and ecology should not be affected by energy crops, and (5) deforestation should not be the reason
[8]. The SAF should be produced from biological feedstocks, such as non-edible oil crops (e.g., Jatropha, Palm, and Camelina), algae, municipal sewage and agricultural wastes
[7][8][7,8]. The demand for jet fuel is 5–6 million barrels/day and is expected to increase by 15% by 2030 and account for 3.4% of the global CO
2 emissions. Of the required global fuel supply of 6–7 billion litres/year, 30% is expected to be from bio-sources by 2030 according to the International Air Transport Association (IATA)
[9][10][9,10]. In this context, offsetting CO
2 emissions using microalgae is attractive since 1 tonne of microalgae can absorb 1.8 tonnes of CO
2 from the 29 gigatons of CO
2 that is released into the atmosphere annually from burning fossil fuels
[9][10][9,10]. Improvements in other areas, such as lighter materials, improved engine design, and aerodynamics, have resulted in a 70% reduction in aircraft traveller/kilometre and tonne/kilometre consumption
[11].
For jet fuel, the aviation industry primarily uses two types of fuel: aviation gasoline and aviation kerosene
[12][22]. Aviation gasoline, also known as AVGAS, is derived from the distillation of crude oil in the range between 30 and 170 °C
[13][14][23,24]. This fuel mainly consists of isoparaffins with C
5 to C
9 carbon and a small amount of aromatic carbon. Alkylates are a mix of high-octane, low-vapour-pressure, branched paraffinic hydrocarbons used for blending into gasoline
[13][15][23,25]. AVGAS is normally used in small aircraft, such as private aviation, agricultural, and pilot training. Aviation kerosene is also a petroleum distillation product obtained in the range between 150 and 300 °C
[13][15][23,25]. This fuel is more suitable in aircraft engines for energy generation through combustion, used in many commercial and military aircraft
[16][26]. Many developed countries are following the jet fuel specifications, which are compatible with the Aviation Fuel Requirement for Operated System (AFQRJOS) (
Table 1).
Table 1. Regulatory agency and standards of aviation fuel around the world [15]. Regulatory agency and standards of aviation fuel around the world [25].
Regulating Agency |
Country |
Standards/Resolution |
Commercial Name |
Methods |
Description |
Agência Nacional do Petróleo, Gás Natural Biocombustíveis |
Brazil |
Resolution No. 37 |
Jet A-1 |
FT-SPK |
Fischer-Tropsch synthetic paraffinic kerosene (FT-SPK). Biomass is converted into syngas and then biofuels via the FT process. ASTM approved the approach in 2009, and the UK MOD Def-Stan (91-91) approved it in 2010. FT-SPK aviation biofuel can be blended up to 50% with fossil jet fuel. |
Federal Aviation Administration |
USA |
ASTM D1655/ASTM 6615 |
Jet A, Jet A1/Jet B |
Transport Canada Civil Aviation |
HEFA |
Hydro-processed fatty acid esters and free fatty acid (HEFA). Hydrogen is used to transform liquid feedstock, including vegetable oils, cooking oil, and tallow, into green diesel, which can then be isomerised and separated to produce a jet fraction. In 2011, the route was certified for a 50% blend with fossil jet fuel. |
Canada |
CAN/CGSB-3.23 CAN/CGSB-3.22 |
Jet A/A1/Jet B |
Civil Aviation Authority |
UK |
DefStan 91–91 |
Jet A1 |
European Aviation Safety Agency |
EU |
AFQRJOS |
Jet A1 |
Federal Air Transport Agency |
Russia |
GOST 10,227/GOST R 52,050 |
TS-1/Jet A1 |
Civil Aviation Administration of China |
China |
GB 6537 |
No. 3 |
2. Aviation Fuel Specification
Aviation fuel properties and compositions should meet the fuel specifications standards
[17][27]. They should have the lowest energy density by mass, the highest permitted freeze point, the highest allowable viscosity, the highest allowable sulfuret and aromatic content, the highest allowable acidity, and the lowest allowable flash point
[18][19][20][28,29,30]. Parameters for the certified international standards for aviation jet fuel are presented in
Table 2 and agree closely with one another in many respects.
Table 2. International standards for aviation jet fuel [18][19][20]. International standards for aviation jet fuel [28,29,30].
Properties |
Unit |
ASTM 1655-4a |
Def Stan 91-91 |
ANPn°37 |
Density |
g/mL |
0.775–0.840 |
0.775–0.840 |
0.771–0.836 (20 °C) |
Viscosity at 20 °C |
mm2/s |
8.0 max |
8.0 max |
8.0 max |
Acid value |
mgKOH/g |
0.100 |
0.0012 |
0.015 |
HFS-SIP |
Hydro-processing of fermented sugars–synthetic isoparaffinic kerosene (HFS-SIP). Sugars can be transformed to hydrocarbons using modified yeasts. The current permitted technique creates a C15 hydrocarbon terpenoid, farnesene. ASTM authorised this technology in 2014, and it can be combined with fossil jet fuel up to 10%. |
Flash point |
°C |
38 min |
38 min |
38–40 min |
FT-SPK/AHeat of combustion |
MJ/kg |
42.8 min |
42.8 min |
42.8 min |
Freezing point |
°C |
−47 |
−47 |
−47 |
Sulphur |
% |
0.3 |
0.3 |
0.3 |
Aromatics |
% |
25 |
25 |
25 |
This is a modified FT-SPK process. Light aromatics are alkylated to yield a hydrocarbon mix with an aromatic component. This method was authorised in 2015 and can blend up to 50%. |
ATJ-SPK |
Alcohol-to-jet-synthetic paraffinic kerosene (ATJ-SPK). Hydro-processing, dehydration, and oligomerisation are used to convert alcohols (iso-butanol) into hydrocarbon. A certified process allows a maximum 50% blending. |
Co-processing |
Biological liquid feedstock, such as fats, oil, and other residues, can be blended with fossil crude oil by 5% (v/v) to carry out the refining process. This process was approved in April 2018 by ASTM and certified with ASTM D1655. |
CCS-APR |
Catalytic conversion of sugars by aqueous phase reforming. |
CH |
Catalytic hydrotreating of liquid to jet fuels. |
Smoke point |
Mm |
25 min |
25 min |
25 min |
JFTOT Delta P (260 °C) |
mmHg |
25 |
25 |
25 |
Conductivity |
pS/m |
50–450 |
50–600 |
50–600 |
Maximum boiling point |
°C |
300 max |
300 max |
300 max |
The chemical composition of Jet A and A1 fuels is presented in Table 3. Straight-chain hydrocarbons, C8 (octanes), and cyclic derivatives, both aromatic and non-aromatic, are major components.
Table 3. Chemical composition of Jet A and Jet A1 fuels [18][19][20]. Chemical composition of Jet A and Jet A1 fuels [28,29,30].
CATJ-SKA |
Catalytic upgrading of alcohol intermediate-catalytic ATJ-synthetic kerosene with aromatics. |
ATJ-SPK |
|
expansion |
Catalytic upgrading of ethanol. |
HEFA expansion |
Direct use of a wider cut of HEFA with renewable diesel. |
HDCJ UOP-Eco-refining™ |
Pyrolysis-hydrotreated de-polymerised cellulose. Blending vegetable biodiesels with petroleum-based fuels. |
Compound |
Formula |
Type |
Chemical Structure |
n-octane |
C8H18 |
n-paraffin |
 |
2-Methylheptane |
C8H18 |
Isoparaffin |
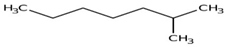 |
1-Methyl-1-ethylcyclopentane |
C8H14 |
Cycloparaffin |
 |
Ethyl-cyclohexane |
C8H16 |
Cycloparaffin |
 |
o-Xylene |
C8H10 |
Aromatic |
 |
p-Xylene |
C8H10 |
Aromatic |
 |
Cis-Decalin |
C10H18 |
Cycloparaffin |
 |
Tetralin |
C10H12 |
Aromatic |
 |
Naphthalene |
C10H8 |
Aromatic |
 |
n-Dodecane |
C12H26 |
n-paraffin |
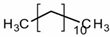 |
2-Methylundecane |
C12H26 |
Isoparaffin |
 |
1-Ethylnaftalene |
C12H12 |
Aromatic |
 |
n-Hexylbenzene |
C12H18 |
Aromatic |
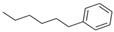 |
n-Hexadecane |
C16H34 |
n-paraffin |
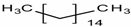 |
2-Methylpentadecane |
C16H34 |
Isoparaffin |
 |
n-Decylbenzene |
C16H26 |
Aromatic |
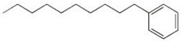 |
3. Kerosene
Kerosene is a clear hydrocarbon-rich liquid obtained from petroleum distillation over a temperature range of 150 to 300 °C
[21][14]. It is less viscous than biodiesel with a density of 0.78–0.81 g/mL and is composed of hydrocarbons predominantly from C
10 to C
16, although the range can extend to C
6–C
20. The main constituents are branched and straight-chain alkanes, as well as cycloalkanes, which account for at least 70% of the total volume
[19][29]. Studies on synthetic alternative jet fuels with predominantly linear and lightly branched alkane content provide stronger low-temperature ignition characteristics, while other types of synthetic alternative jet fuels with a high content of highly branched alkanes exhibit weaker, low-temperature ignition characteristics, when compared to conventional jet fuels
[22][31]. Aromatic hydrocarbons such as alkyl benzenes and alkyl naphthalene are rarely seen in concentrations greater than 25% by volume. Alkene content is low and typically makes up less than 5% of the total volume. Kerosene has a flash point of 37 to 65 °C and an autoignition temperature of 220 °C. Kerosene has a freezing point of −47 °C; however, this varies depending on the quality of the aviation fuel requirements
[23][32]. Kerosene has a lower heating value of around 43 MJ/kg and a higher heating value of around 46 MJ/kg, similar to fossil diesel. The ASTM D-3699-78 recognises two grades of kerosene: 1-K (less than 0.04% sulphur by weight) and 2-K (0.3% sulphur by weight). The 1-K grade is better than the 2-K grade, providing cleaner combustion and fewer toxic emissions
[23][32].
4. Routes to SAF Production
Several conversion routes have been developed to convert biomass into aviation fuel, and some key features are highlighted in
Table 4, which include hydro-processing of esters and fatty acids (HEFA), Fischer-Tropsch (FT), and alcohol-to-jet (ATJ)
[24][33]. In this regard, the only commercially demonstrated process for 100% SAF is the UOP-Eco-refining™ technology developed by Honeywell (USA).
Table 4. Sustainable aviation fuel (SAF) pathways [24]. Sustainable aviation fuel (SAF) pathways [33].