Peripheral neuropathy is the primary predisposing factor in DFU development, due to long-term hyperglycemia, which results in oxidative stress and damages the sensitive, motor, and autonomous nerves
[10,28][1][2]. Sensory defects manifest as a loss of sensitivity to injury and stimulation in the lower limbs due to small-fiber nerve dysfunction, thereby promoting constant unconscious trauma and subsequent ulceration
[10][1]. These sensory defects may include sensory dullness, numbness, and abnormal pain, among others
[10,26][1][3]. By generating intrinsic muscle weakness and atrophy, consequently leading to biomechanical anatomical changes in the feet such as hammer toe, Charcot’s ankle, pes-planus, and pes-cavus, motor neuropathy triggers high-pressure zones in the feet
[3,8,10,26,28][1][2][3][4][5]. This increased shear stress and friction force further promote foot ulceration
[10][1]. Peripheral sympathetic nerves may also be damaged, causing thermoregulatory dysfunction that involves altered sweating, dry skin, cracking, and calluses, subsequently facilitating ulceration
[10,26][1][3].
2. Impaired Healing in Diabetic Foot Ulcers
DFUs may arise from several risk factors that collectively impair the wound healing process of these individuals. Wound healing is a process with four overlapping phases, involving a complex and dynamic sequence of cellular and biochemical events to restore skin integrity and functionality after trauma
[5,40,41,42][7][14][15][16]. The first phase—hemostasis—begins immediately after skin injury with the constriction of the damaged blood vessels and activation of platelets
[5,40,43][7][14][17]. This promotes platelet aggregation and the subsequent formation of a fibrin clot, covering the injured endothelium and consequently stopping the bleeding
[5,40,43][7][14][17]. The second phase—inflammation—starts with the recruitment of neutrophils to the wound site, as a first line of defense against pathogens
[5,40,41,43][7][14][15][17]. After a peak population between 24 and 48 h post skin injury, the number of neutrophils greatly reduces and pro-inflammatory M1 phenotype macrophages arrive successively at the wound site to continue clearing microbial pathogens and debris
[5,40,41][7][14][15]. In detail, M1 macrophages attract different types of adaptive immune system cells to the wound site by the secretion of cytokines and chemokines, either to continue clearing cellular debris or to fight infection
[5,40,41,42][7][14][15][16]. These macrophages then switch from the pro-inflammatory M1 to anti-inflammatory M2 phenotype as inflammation resolves to further foster tissue regeneration, producing anti-inflammatory cytokines and growth factors. The third phase—proliferation—occurs with the formation of granulation tissue to fill the wound, the contraction of the wound borders, wound coverage with epithelial cells (i.e., re-epithelialization), and neovascularization
[5,40,41,42,43][7][14][15][16][17]. The fourth phase—remodeling—involves collagen fiber reorganization, tissue remodeling and maturation, and an overall increase in tensile strength
[5,40,41,43][7][14][15][17].
Nonetheless, the impairment of local and systemic factors in individuals with DFUs leads to a poorly orchestrated cascade of the four phases, thus delaying or even interrupting the healing process, as per
Figure 1. The concurrent presence of DM and DFU stimulates an unbalanced accumulation of immune cells, as well as an increase in the M1/M2 macrophage ratio, reactive oxygen species (ROS), and pro-inflammatory cytokines, together ending in chronic non-healing wounds that remain in a state of low-grade inflammation
[5,16,26,42][3][7][16][18]. In detail, Erem et al. showed that patients with DM exhibited hypercoagulability and decreased fibrinolysis during the hemostasis phase, compared to healthy individuals
[44][19]. Patients with DM have also been associated with an imbalance in cytokine release by neutrophils during the inflammatory phase, thus favoring wound infection
[26,45][3][20]. Furthermore, fibroblast and keratinocyte migration, as well as their proliferative capacity, is compromised in patients with DM due to hyperglycemia, leading to poor re-epithelialization of the wound
[46,47][21][22]. On top of this compromised cell migration, angiogenesis is also reduced in patients with DM, resulting in decreased blood supply to the wound site
[48][23]. During the remodeling phase, patients with DM have also shown altered fibroblast function, contributing to flawed closure of the wound
[49][24]. This may probably be explained by an inefficient response to transforming growth factor beta (TGF-β) from fibroblasts, as well as an aberrant production of the extracellular matrix
[26,49][3][24].
Figure 1. Healthy versus impaired phases of the healing process: the case of diabetic foot ulcers (DFUs) (produced using BioRender). Under DFU conditions, an unbalanced accumulation of immune cells and an increase in the M1/M2 macrophage ratio, reactive oxygen species (ROS), and pro-inflammatory cytokines occur. In addition, re-epithelialization and angiogenesis are scarce, altogether ending in chronic non-healing wounds that remain in a state of low-grade inflammation.
3. Management of Diabetic Foot Ulcer
Current therapeutic approaches for managing DFUs involve multidisciplinary strategies that address key aspects of diabetic wound care, including glycemic control, adequate arterial supply, the debridement of necrotic tissue, pressure offloading, and the treatment of any infection with appropriate broad-spectrum antibiotics
[3,4,9,11,12,13,14,15,16][4][18][25][26][27][28][29][30][31]. For example, it was recently reported that the MADADORE acronym corresponds to the recommended DFU management principles: Metabolic control, Assessment of foot, Debridement, Antibiotics, Dressing, Offloading pressure, Referral to multidisciplinary teams, and Education
[50][32]. Metabolic control involves the management of associated medical conditions such as hyperglycemia and hyperlipidemia, through adequate medication and dietary counseling
[51][33]. Assessment of foot concerns the correct evaluation of the associated risk factors and classification of the ulcer according to the perfusion, extent, depth, infection, and sensation (PEDIS) scale
[52][34]. Debridement involves the surgical removal of any necrotic or unhealthy tissue, while treating any infection with appropriate broad-spectrum antibiotics. Furthermore, dressings are needed to foster wound exudate absorption and create a protected environment propitious for tissue regeneration. In turn, offloading through minimally invasive surgery such as the minimally invasive metatarsal osteotomies is essential to reduce plantar pressure, while supporting minimal tissue damage, immediate post-operative weight bearing, and a lower risk of potential infections, consequently preventing recurrent ulceration
[53,54][35][36]. Last but not least, referral to multidisciplinary teams means the indication of appropriate adjuvant therapies for the optimal management of DFUs, such as stress-reducing approaches
[55[37][38],
56], while education is fundamental to improve DFU health literacy for the prevention of future ulcers.
However, despite these efforts, the efficient management of DFUs remain a clinical challenge, with limited success rates in treating severe infections
[17,18][39][40]. Indeed, current DFU treatments still exhibit a huge recurrence rate of 40% within one year, 60% within three years, and 65% within five years
[8[2][5][29][41],
13,28,57], due to persistent risk factors even after the former ulcer has healed
[58][42]. Consequently, there is a crucial need for novel strategies that can successfully address the multifactorial etiology of DFUs.
4. Biomaterials as a Promising Therapeutic Platform for Wound Dressings
A therapeutic strategy with rising potential to handle the challenging macro and micro wound environment of individuals with DM involves the use of biomaterials as wound dressings. Biomaterials have long been related to unique versatility, biocompatibility, biodegradability, and hydrophilicity, characteristics that make them ideal candidates for therapeutic applications [22,23,24][43][44][45]. Furthermore, biomaterials have also been explored for their innate properties for wound healing [21,22,23,24][43][44][45][46]. An ideal wound dressing for the management of DFUs should present several key features. Firstly, it must demonstrate excellent biocompatibility and biodegradability to ensure tissue healing. Secondly, it should create a moist and warm environment conducive to tissue regeneration. Thirdly, the dressing should prevent polymicrobial infections to ensure proper wound healing. Finally, it should exhibit adequate porosity that enables gas exchange, and stimulate cell migration, proliferation, and neovascularization, as depicted in Figure 2 [21,22][43][46].
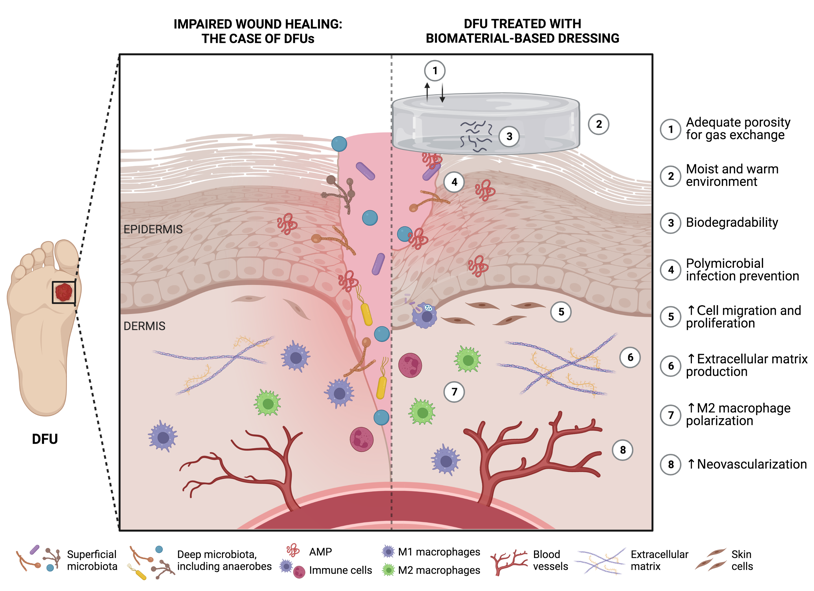
Figure 2. Potential features of functional biomaterials as ideal wound dressings for diabetic foot ulcer (DFU) healing (produced using BioRender). ↑ represents an increase.