The use of wood has gained social interest, leading to a global increase in its demand. Yet, this demand is often covered by the production of woods of low natural durability against biological deterioration. The main biological agents with the potential to attack the structural integrity of wood are wood-decay fungi, saproxylic beetles, termites, and marine molluscs and crustaceans. In most circumstances, fungi are the main wood-deteriorating agents. To attack the cell wall, wood-decay fungi combine a complex enzymatic mechanism with non-enzymatic mechanisms based on low-molecular-weight compounds. In some cases, the larvae of saproxylic beetles can also digest cell wood components, causing serious deterioration to wooden structures. The impact of subterranean termites in Europe is concentrated in the Southern countries, causing important economic losses. However, alien invasive species of voracious subterranean termites are expanding their presence in Europe. Wooden elements in permanent contact with marine water can be readily deteriorated by mollusc and crustacean borers, for which current preservatives lack efficacy. The natural durability of wood is defined as the inherent resistance of wood to catastrophic action by wood-destroying organisms. Besides exposure to the climate, product design and use conditions, the natural durability of wood is key to the prediction of the service life of wooden products, which can be shortened due to the impact of global change.
1. Biological Agents Responsible for Wood Deterioration and Potential Shifts Caused by Global Change
This section reviews the main biological agents causing the deterioration of wood in service in Europe. Because some agents, particularly termites, are found within a limited geographical distribution in Europe, the risk of attack by a particular biological agent will depend on the geographical location of the wooden element
[1] (European Standard EN 335:2013). The likelihood that a wood product will be attacked by a particular biological agent is also influenced by the local environmental conditions that surround the wooden element, with those factors affecting the moisture level that can be reached in wood being of particular importance (e.g., climate, soil texture, structure and organic matter content, constructive design, etc.). Furthermore, global change has significantly altered the spatial distribution and long-distance spread of forest insects and pathogens
[2]. The long-distance transfer of non-native biotic agents to new locations is most notable for the emergence of various novel forest pests, which can affect wooden products (e.g., the invasive
Reticulitermes flavipes or
Lyctus brunneus in Europe). Pest and pathogen introductions have primarily been facilitated by international trade and the movement of infected live plants, wood materials, or soil
[3], and the dispersal paths of biotic agents can be altered by changing climatic circumstances. Increasing temperatures affect many aspects of insect behavior, such as flight distances and the timing of seasonal emergence
[4]. A number of bark beetle pests, such as
Ips typographus and
Dendroctonus ponderosae, have been recorded to have earlier emergences and extended flight season lengths in warming conditions. Insect and microorganism survival rates are also directly impacted by climate change, which also affects the population growth rates of the agents. Increasing winter temperatures in temperate locations have been related to changes in the prevalence and effects of a range of biotic agents because rates of overwinter survival may restrict epidemics and breakouts (e.g.,
[5]). Insects may be able to produce more generations in a year as a result of warming temperatures and earlier emergence periods, which could have an impact on population growth, overwinter survival, and the risk of outbreaks. Furthermore, complex mutualistic or parasitic connections with symbionts or natural enemies control the populations of many insects, and these interactions may change as climatic circumstances change
[6]. It was predicted that warming and humidity would dramatically shorten the useful life of timber building materials and that the location and current climate would have a significant impact on the severity of climate-induced changes
[7].
Table 1 summarizes some of the most frequent biological agents deteriorating wood in service in Europe and the estimated risk of attack according to the class of use. In the following section, the researchers delve into the mechanisms of wood deterioration by these biological agents. The term ‘wood deterioration’ is used in this research in a broad sense and refers to any biological agent able to affect wood properties, such as mechanical or aesthetical traits. The term ‘wood decay’ is used specifically for the biochemical decomposition of wood by microorganisms.
Table 1. Frequent biological agents deteriorating wood in service in Europe.
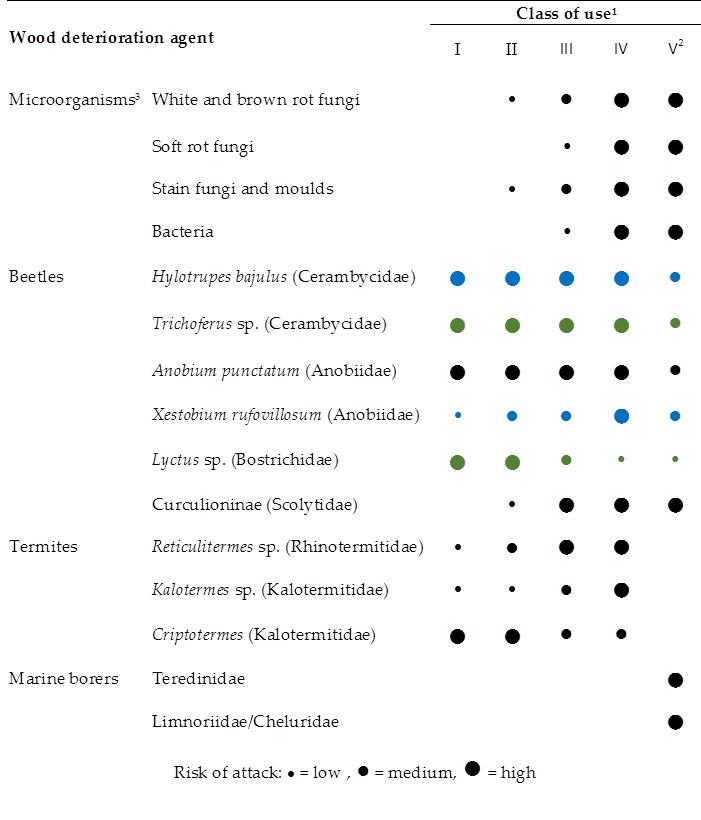
1 The risk of attack is tentatively presented for the five classes of use as an interpretation of the authors of the existing literature in the field, their personal experience and the European Standard EN 335:2013, which defines the five classes of use. Absence of dots indicates negligible risk of attack. Black dots: risk for hardwoods and softwoods; green dots: risk mainly for hardwoods; blue dots: risk mainly for softwoods. I = wood indoors without exposure to moisture; II = wood indoors with occasional exposure to moisture; III = wood outdoors without contact with soil or water; IV = wood in contact with soil or freshwater; V = wood submerged in saltwater. It should be acknowledged that the frequency and significance of the biological risk largely depend on the geographic location, particularly in the case of termites, which are locally present in Europe (see Figure 5). 2 The aerial part of certain elements may be subject to attack by xylophagous insects. 3 Submerged or water-saturated wood is not susceptible to attack by white- and brown-rot fungi, stain fungi and moulds but is susceptible to attack by bacteria and soft-rot fungi.
2. Factors Underlying the Natural Durability of Wood against Biological Deterioration
The natural durability of wood is defined as the inherent resistance of wood to attack by wood-destroying organisms (European standard EN 350:2016). Biological durability is an important factor in the selection of wood or wood products for a particular use. In addition to the climate, product design and use conditions, natural durability is key to the prediction of the service life of wooden products. The natural durability of wood against organisms such as wood-decay fungi and insects can be quantified through standardized tests, whether at the laboratory or field level. These tests are specified in the European standard EN 350:2016 and are specific for the most common deterioration agents. EN 350:2016 classifies the natural durability of wood against fungi into five classes, 1–5, where 1 is very durable and 5 is perishable; against insects into durable and susceptible; and against termites and marine organisms into three classes.
As a biological material, wood is heterogeneous, and its durability varies across species, among populations within species, between individual trees of the same species and even within a given tree. Most of this variability is controlled genetically, while some is due to the environment and cambial age
[8][9][10]. It is difficult to find consistent trends in wood durability among species. Many tropical species are durable, but not all, whereas the researchers can also find very durable species in temperate regions (European standard EN 350:2016). Long-lived species usually have a durable heartwood, the inner part of the wood with no living cells and no reserve material
[11], but there are a few exceptions, such as
Picea sitchnensis,
Fraxinus excelsior or
Fagus sylvatica, which may show very active defense mechanisms in the sapwood of living trees to hamper the wound invasion of a decay-susceptible heartwood (European standard EN 350:2016). Species with distinct heartwood are more durable than those with no visual difference between heartwood and sapwood, the outer part containing living cells and reserves in the living tree
[11]. The heartwood proportion within species appears to be under genetic control
[12], although the environment and management can also be important
[10]. For instance, Climent et al.
[13] found that the heartwood of
Pinus canariensis was wider in drier sites, and Margolis et al.
[14] reported that severe pruning increased heartwood diameter in
Abies balsamea. Between functional groups, a meta-analysis
[15] showed that gymnosperms generally tend to have lower decay rates than angiosperms, i.e., better durability. These results agreed with the lower decay rates found in temperate gymnosperms compared with temperate angiosperms
[16].
The major wood properties involved in wood durability are the amount and composition of heartwood extractives, i.e., secondary low-molecular-weight organic compounds which are produced during heartwood formation, although other factors such as wood anatomy, wood density, lignin content and moisture content are also involved
[17][18] (
Figure 1). In fact, the durability of different species is determined by different factors. For instance,
Prunus avium relies mainly on the fungicidal components of the heartwood, whereas the durability of
Entandrophragma cylindricum depends more on the moisture-regulating components
[17]. In wooden products obtained from the same tree, the heartwood is more durable than the sapwood due to the higher concentration of toxic extractive compounds, such as alkaloids, phenols, and terpenes. In general, extractive content increases from the pith towards the outer heartwood and reaches a maximum at the transition zone between heartwood and sapwood; thus, as the tree ages, the extractive content increases
[19], and the decay susceptibility decreases
[20]. Longitudinally, extractive concentration decreases with tree height. In many species, the outer heartwood at the base of the tree is the most resistant to decay
[18][21]. Durability gradients may reflect biological detoxification, natural oxidation of extractives, continued polymerization of extractives to produce less toxic compounds or increases in extractive deposition with age
[19][21].
Figure 1. Anatomical, chemical and environmental factors involved in natural durability of wood. According to the vertical axis, factors vary from macroscopic to microscopic and molecular scales. Created with BioRender.com.