Multiple myeloma is characterized by a procoagulant predisposition, and patients with multiple myeloma (MM) have an approximately nine-fold higher risk for venous thromboembolism (VTE) than the general population
[1][2]. The emergence of VTE as a significant complication in MM patients parallels the use of multiagent chemotherapy regimens (doxorubicin, vincristine, and more), and later on, the introduction of immunomodulatory agents (IMiDs). The association between IMiDs and VTE risk is well established in the literature
[1][2]. Both venous and arterial thrombosis are a leading cause of morbidity, but evidence of the association between VTE and mortality in MM is limited and conflicting
[3][4][5][6]. As the therapeutic options for the disease expand, and MM increasingly acquires a “chronic” disease trajectory, the question of optimal VTE prophylaxis becomes increasingly relevant. The International Myeloma Working Group (IMWG)
[7], the European Myeloma Network (EMN) and the National Comprehensive Cancer Network (NCCN)
[8] have published guidance on the prevention of MM-associated thrombosis. A consensus-based position paper was also released earlier this year
[9]. Appropriate risk identification for VTE is advocated for optimal care of patients with MM, and central to the framework of international guidelines is the ability to appropriately risk stratify MM patients for VTE. Patients with myeloma should be risk-stratified for VTE risk and then offered aspirin; low molecular weight heparin (LMWH); or according to more recent updates of guidelines, direct oral anticoagulants (DOACs), for thromboprophylaxis. Three MM-specific VTE risk assessment models have been developed and validated; one by International Myeloma Working Group (IMWG)
[7], and more recently, the SAVED and the IMPEDE VTE
[10] models. Recognizing and understanding the multiparametric nature of the procoagulant environment observed in MM is paramount for optimal risk stratification. Interestingly, VTE rates are also higher in the pre-symptomatic stages of the disease, such as monoclonal gammopathy of undetermined significance (MGUS)
[11][12][13][14]. Unfortunately, little progress has been made in the field over the last few years, and VTE rates remain high, often over 10%, both in the clinical trials and real-world settings
[1][2]. Data supporting the safety and efficacy of DOACs are increasingly emerging, but head-to-head comparisons between agents are still missing, and randomized control trial (RCT) data are scarce
[15][16][17][18][19]. The type and duration of thromboprophylaxis remain controversial.
2. Risk Factors for Thrombosis
Thrombogenicity in MM is multiparametric. Patient-specific “standard” risk factors, features related to disease biology and treatment-related factors interact to formulate the thrombotic risk observed in each patient (see Figure 1).
Figure 1. Risk factors for thrombosis in multiple myeloma. MM: multiple myeloma, TF: tissue factor, FVIII: factor VIII, vGF: von Willenbrand factor, TF-MPs: tissue factor-derived microparticles, PAI-1: plasminogen activator inhibitor, NETs: neutrophil extracellular traps, PPLs: procoagulant phospholipids, TM: thrombomodulin, EPCR: endothelial protein C receptor, APC: activated protein C, t-PA: tissue plasminogen activator, Abs: antibodies, EPO: recombinant erythropoein, BMI: body mass index, VTE: venous thromboembolism.
2.1. Patient-Related Risk Factors
Standard risk factors for thrombotic risk apply for the MM patient
[20][21]. The incidences of factor V Leiden and PTG20210A polymorphisms in patients with MM are similar to the general population, and no other genetic variants have been described
[22][23].
2.2. Disease-Specific Risk Factors
A new diagnosis of multiple myeloma is considered a risk factor for thrombosis compared to relapsed/refractory disease
[2]; however, VTE rates remain high also in the relapsed setting. As reported recently in a meta-analysis, the VTE incidence per 100 patient-cycles was comparable in newly diagnosed patients and in the relapsed setting, at 1.2 and 1.2, respectively
[24]. Numerous studies have confirmed that most events occur within six months of treatment initiation, and this finding is independent of the treatment type
[1][4]. Several groups have demonstrated that patients with MGUS and smoldering MM are also at a higher risk of developing VTE compared to the general population
[11][12][13][14], in support of the notion that MM as a disease is a hypercoagulable state. In a large hospital-based study by Kristinsson et al.
[11], during 17 years of follow-up, a 3.3-fold increase in DVT risk was observed in MGUS patients (n = 2374) compared to the healthy population. More recently, another large population-based Danish cohort study reported an incidence rate of 4.0 VTEs/1000 person-years in MGUS patients, and the IRR for VTE compared to a healthy control cohort matched with MGUS patients was 1.37
[25].
The exact mechanisms that underly disease-related hypercoagulability in MM remain to be delineated. There are data to support that hyperviscosity, inhibition of natural anticoagulants
[26] and an increase in procoagulant inflammatory cytokines such as interleukin-6 (IL-6) and tumor necrosis factor-a (TNFa) all contribute to the prothrombotic environment observed. Monoclonal paraprotein also interferes with fibrin polymerization
[27], clot retraction and clot lysis time
[28][29], and hypofibrinolysis is observed
[30]. More recently, immunoglobulin k light chain was found to be associated with dysfibrinogenemia through a mechanism of fibrin interference
[31]. Chalayer et al. recently identified serum gamma globulin levels >27 g/L (2.8 [1.2–6.8],
p = 0.02) as an independent prognostic factor for VTE occurrence in patients eligible for autologous transplantation who receive bortezomib, dexamethasone and lenalidomide induction.
Other proposed mechanisms include acquired activated protein C resistance; lupus antibody anticoagulant activity by the monoclonal component; increased microparticle-associated tissue factor (TF); elevated von Willebrand factor (vWF), fibrinogen or factor VIII; decreased protein C; and increased thrombin generation
[26][27][32][33][34]. A recent review summarized the data regarding the association between increased vWF/FVIII levels in patients with MM, increased risk of thrombosis and decreased overall survival
[35]. Altered platelet function has also been reported, but data are not conclusive yet; Egan et al.
[36] reported decreased platelet aggregation in MM patients but no link between platelet hyporeactivity and paraprotein levels, and Sullivvan et al. reported platelet hyperactivation with increased CD63, PAC-1 expression and annexin V in resting platelets of MM and MGUS patients compared to healthy controls
[37].
2.3. Treatment-Related Risk Factors
Treatment-related factors are key determinants of the final thrombotic risk of MM patients. The introduction of IMiDs was associated with a significant rise in VTE occurrence among the MM population. Thalidomide or lenalidomide (Len) monotherapy baseline risk is around 3–4%, but this increases substantially when combined with dexamethasone. The dose of dexamethasone and the partner drugs affect the risk significantly; the risk increases up to 26% with the addition of high-dose dexamethasone or multiagent chemotherapy
[38][39][40][41]. Lenalidomide maintenance also confers a significant thrombotic risk; both venous and arterial events were significantly higher compared to placebo in the myeloma XI trial
[42]. Notably, the VTE risk persists following drug cessation
[43]. Fewer data exist regarding pomalidomide; the VTE risk reported is around 2–3% when pomalidomide is administered in combination with dexamethasone. The VTE risk is therefore lower compared to lenalidomide-based combinations. It should be, however, taken into account that data come from the relapsed setting only and following the implementation of regular thromboprophylaxis in this patient population
[44][45][46].
The exact mechanisms underlying the IMiD-induced thrombogenic effect are unknown, but higher levels of P-selectin, fibrinogen and homocysteine following lenalidomide treatment have been reported. Platelet activation is enhanced, and resistance to protein C, mediated by cytokines following IMiD-based therapy, has been reported
[47]. High-dose dexamethasone increases the levels of P-selectin, vWF and FVIII
[48], and there are reports of higher plasma thrombin levels following therapy with doxorubicin
[49].
The proteasome inhibitor (PI) bortezomib is associated with a low risk of VTE
[50][51]. A protective effect has even been reported in some studies when bortezomib is combined with IMiDs
[52][53], but more recent data are conflicting; two RCTs did not show a significant difference in the all-grade risk of thrombosis when IMiD plus dexamethasone is combined with bortezomib
[54][55]. In contrast to bortezomib, the second-generation PI carfilzomib has a different adverse effect profile and is associated with significant cardiovascular side effects, but less is known about its VTE risk. In the newly diagnosed setting, VTE rates reported for carfilzomib-IMiD-based combinations range from 5 to 14%, but a uniform approach to thromboprophylaxis is missing
[56][57][58]. In a recent study, the VTE rate was 16.1% for patients on KRD (carfilzomib, lenalidomide, dexamethasone), and it was 4.8% for patients on VRD (bortezomib–Rd), confirming the different profiles of the two PIs in terms of thrombotic risk
[58]. The cause of carfilzomib-induced cardiotoxicity is suspected to be a result of endothelial dysfunction
[59]. Whether the mechanisms of endothelial dysfunction are involved in venous thromboembolic events is still unknown, but many groups support that patients on the KRd combination should be considered high risk in terms of VTE risk
[58].
Other agents with anti-myeloma activity, such as the monoclonal antibodies, daratumumab, belantamab and elotuzumab, and the oral proteasome inhibitor ixazomib, have not been associated with an increased risk of thrombotic complications
[57][58][60][61], at least in the clinical trial setting. In the GRIFFIN study, daratumumab with VRD (DVRd) was compared to VRd, and the all-grade cumulative rates of VTE were 10% and 15%, respectively
[62]. A post-hoc pooled analysis of the phase III CASTOR, POLLUX and MAIA trials demonstrated that daratumumab treatment did not affect VTE risk
[63].
3. Risk Assessment Models for VTE in Multiple Myeloma
The importance of risk assessment models (RAMs) for thrombosis prediction in cancer patients has become well-established since the Khorana risk score was developed in 2008
[64]. However, its discriminative power for VTE risk in patients with MM is poor, as reported by Sanfilippo et al. (assessment in 2870 MM patients)
[65]. In the MM population, the only parameter that retains its predictive significance for VTE is the white blood cell count
[66]. Research efforts focused on the development of MM-specific RAM. Three MM-specific RAMs have been published and validated to date for predicting VTE in MM patients: the earlier International Myeloma Working Group (IMWG)
[7], and more recently, the SAVED
[67] and the IMPEDE VTE
[10] models. The NCCN guidelines historically adopted the IMWG score, but it has performed poorly when applied in the clinical trial setting (
Table 1). Bradbury et al. reported the thrombosis rate in Myeloma XI and XI clinical trials and demonstrated the suboptimal predictive power of the IMWG model
[42]. Half of the patients who developed VTE in the Myeloma XI trial were not classified as high risk using the IMWG scoring system
[52]. A minimum of 3 months of treatment with LMWH was recommended for high-risk patients, and aspirin for low-risk patients. Out of the 3838 patients treated in the trial, the VTE rate was 11.8%. At the time of the event, 87.7% were on thromboprophylaxis. The use of the IMWG score outside clinical trials has been moderate
[68]. In a retrospective review, 80 MM patients with VTE were identified and matched to controls (MM patients without VTE); among patients with VTE, 82% were considered high risk and 18% low risk at baseline. Only 19% of patients received appropriate thromboprophylaxis (LMWH, aspirin, none) based on IMWG risk-score baseline assessment
[68].
The IMPEDE-VTE score was developed using retrospective data from the Veterans Administration Central Cancer Registry (VACCR) in 4446 patients, and it was subsequently validated using the Surveillance, Epidemiology, End Results (SEER) Medicare database. The derivation cohort included patients who were diagnosed with MM between 1 September 1999 and 30 June 2014 and had received chemotherapy for MM; patients were followed up retrospectively for 180 days to determine the occurrence of VTE events. The validation cohort from the SEER database included patients who were Medicare eligible (≥65 years old) and were diagnosed with MM from January 2007 to December 2013. Three risk groups were identified; the respective 6-month cumulative incidence of VTE following treatment initiation was 3.3% for the low-risk group, 8.3% for the intermediate-risk group and 15.2% for the high-risk group
[10]. A recent validation of the IMPEDE score in 575 patients with NDMM reports a 6-month cumulative incidence for VTE of 5% (95% CI: 2.1–7.9) in the low-risk group, compared to 12.6% (95% CI: 8.9–16.4%) and 24.1% (95% CI: 12.2–36.1) in the intermediate- and high-risk groups, respectively (
p < 0.001 for both)
[69]. The SAVED risk score (surgery, Asian race, VTE history, eighty years old, dexamethasone) was developed around the same time using the SEER database and was validated using the Veterans cohort
[67]. It was developed only in patients on IMiD-based therapy; patients were stratified as low or high risk for VTE with hazard ratios of 1.85 (
p < 0.01) and 1.98 (
p < 0.01) in the derivation and validation cohorts, respectively. In the recent retrospective study by Piedra et al., who reported VTE rates for KRd and VRd and compared rivaroxaban to ASA, the SAVED model did not predict VTE, but due to the retrospective design of the study high-risk patients on anticoagulation were excluded
[58]. The SAVED scores were calculated retrospectively in the GRIFFIN study for patients in both the Dara-VRd and VRd arms. Median SAVED scores in both groups were in the low-risk category (0 and 0.5 scores, respectively); the VTE risk was low, but the incidence of VTE in both groups was relatively high
[62]. In addition, only 60% of patients in the Dara-RVd and 67% in the RVd arm were on antithrombotic treatment at the time of VTE
[62]. The performances of the SAVED and IMPEDE-VTE risk scores were retrospectively assessed in the CASTOR, POLLUX and MAIA studies; both scores had good discrimination for patients on non-daratumumab-based regimens, and the SAVED score performed better in patients receiving daratumumab
[63].
Validation analysis of the three scores was performed using the two large MM cohorts (SEER-Medicare and Veterans Administration Healthcare System); Harrell’s c-statistics were 0.52 and 0.55, respectively, for the IMWG model, and slightly better for the newer models. Harrell’s c-statistic was 0.60 for the IMPEDE-VTE model and 0.64 for the SAVED model, respectively
[70]. Based on these findings, the IMPEDE-VTE and the SAVED models are preferentially recommended for utilization in the latest guidelines
[71]. However, as Stefano et al. argue in their latest consensus paper
[9], there are insufficient data to recommend one specific RAM over the other in clinical practice. We should also note that both IMPEDE-VTE and SAVED scores were developed using patients who received treatment mostly before the era of novel agent availability used currently in clinical practice.
Table 1a: IWMG score and algorithm for MM patient risk stratification
IWMG score and algorithm for MM patient risk stratification
|
Patient-related risk factors
1 point for each
|
Disease-related risk
factors: 1 point for each
|
Treatment-related risk factors:
points as seen below:
|
Body mass index >25, age>75, Personal or family history of VTE, Central venous catheter, Acute infection or Hospitalization, Blood clotting disorders or Thrombophilia, Immobility with a performance status of >1, Comorbidities (liver, renal impairment, Chronic obstructive pulmonary disorder, diabetes mellitus, chronic inflammatory bowel disease), Race (Caucasian)
|
· Diagnosis of Multiple Myeloma
· Evidence of hyperviscosity
|
•IMiD in combination with low-dose dexamethasone (<480mg/month) (1 point)
•IMiD plus High-dose dexamethasone (>480mg/month) or doxorubicin or multiagent chemotherapy (2 points)
•IMiD alone (1 point)
•Erythropoietin use (1 point)
|
Risk stratification and recommended thromboprophylaxis:
0 points: Low risk - None
1 point: Intermediate risk - Aspirin at 100mg
>1 point: High risk - Low molecular weight heparin at prophylactic dose or therapeutic dose of warfarin
|
Table 1b: IMPEDE and SAVED risk assessment models
CLINICAL RAMs for VTE in MM
|
IMPEDE VTE score
|
SAVED* score
|
Immunomodulatory drug (+4)
BMI ≥ 25 kg/m2 (+1)
Pathologic fracture pelvis/femur (+4)
Erythropoiesis-stimulating agent (+1)
Dexamethasone (High-dose, ≥1600mg/cycle) (+4)
Dexamethasone Low-Dose (<160mg/cycle) (+2)
Doxorubicin (+3)
Ethnicity/Race= Asian (-3)
VTE history (+5)
Tunnelled line/CVC (+2)
Existing use of therapeutic warfarin or low molecular weight heparin (LWMH) (-5)
Existing use of prophylactic LMWH or aspirin (-3)
|
Surgery (within the last 90 days) (+2)
Asian Race (-3)
VTE history (+3)
Eight (age >=80 y) (+1)
Dexamethasone dose
Standard (120-160mg/cycle) (+1)
High (>160mg/cycle) (+2)
*for patients on IMiD-based regimens only
|
Stratified risk groups based on a weighted scoring system
|
Low risk (score of ≤ 3)
Intermediate-risk (score of 4-7)
High risk (score of ≥ 8)
|
High risk (score of ≥ 2)
Low risk (≤1)
|
* for patients on IMiD-based regimens only.
4. Primary VTE Prevention in Multiple Myeloma
Clinical trial data regarding pharmacological thromboprophylaxis in patients with MM are limited. The researchers identified only four randomized clinical trials in the literature that compare antithrombotic agents for VTE prevention for patients with MM on IMiDs
[72], and further evidence comes from non-randomized observational trials. ASA was compared to fixed low-dose warfarin (1.25 mg/day) and to LMWH (enoxaparin 40 mg/day) in 667 NDMM patients who received thalidomide in a randomized clinical trial (RCT). No significant differences were demonstrated between the three agents; the VTE rate at six months was 6.3% in the ASA group, 8.2% in the warfarin group and 5% in the LMWH group
[18]. The rates of cardiovascular events, all-cause mortality and bleeding events also did not differ between groups. Aspirin (100 mg/day) was also compared to enoxaparin 40 mg/day in another RCT in MM patients who received lenalidomide, and no significant differences were reported; the VTE rate was 2.2% in the aspirin group and 1.2% in the enoxaparin group
[19]. Pooled data analysis in a Cochrane systematic analysis demonstrated no superiority or inferiority regarding ASA use compared to LMWH in this setting, and appraisal resulted in very low-certainty evidence
[72]. It should be noted, however, that high-risk patients for thrombotic complications were excluded from both trials.
The VTE rate was 10.7% for patients on ASA and 1.4% for patients on prophylactic LMWH in a retrospective review of 1126 patients with MM, questioning its protective role even in low-risk patients
[73]. A meta-analysis published in 2018 in 1964 patients included data from retrospective and longitudinal studies also; ASA was found to be superior to no intervention (OR = 0.20; 95%CI: 0.07–0.61,
p = 0.005; I
2 = 41%) and inferior to LMWH (OR = 2.60; 95%CI: 1.08–6.25;
p = 0.03; I
2 = 0%)
[74]. In another large retrospective review (4892 patients with MM), 586 patients had a VTE event. After adjusting for IMiD use and prior VTE history, aspirin did not reduce the risk of VTE enough to justify its role
[75]. ASA thromboprophylaxis is, therefore, not recommended during the first six months of first-line treatment in MM patients who receive IMiDs
[76]. In the Myeloma XI study, patients were risk-stratified at baseline; among patients who experienced a VTE, 9.2% were on a therapeutic dose of warfarin, 44.1% on prophylactic LMWH dose and 31% on aspirin. A direct comparison is not possible, given the baseline risk stratification
[52].
The type, intensity and duration of thromboprophylaxis should be patient-specific and tailored to each patient’s baseline thrombotic and hemorrhagic risk
[9]. Important issues when considering the mode of thromboprophylaxis include, of course, effectiveness, but also the route of administration, patient convenience—one should opt for the least intrusive method and safety in the context of frailty—age, cyclical thrombocytopenias, minimizing bleeding risk and impaired renal function. Patient preference, probability of compliance with treatment and cost also need to be considered. Drug–drug interactions and the use of strong cytochrome P inducers/inhibitors should be taken into account. Among anti-myeloma agents, dexamethasone is a strong inducer of CYP3A4 and P-glycoprotein and an inhibitor of P-glycoprotein; bortezomib is a CYP3A4 inhibitor; and venetoclax is an inhibitor of P-glycoprotein
[77][78]. The initial choice of thromboprophylaxis should be adapted to renal function. A patient with thrombocytopenia is another challenge, as clear-cut instructions and thresholds for using different agents are absent
[77][78][79]. Other factors to consider are extremes of body weight, history of heparin-induced thrombocytopenia (HIT), availability of reversal agents and single/dual antiplatelet agent use.
5. Treatment of VTE and Secondary Prevention of VTE
The treatment of acute VTE in cancer patients is well-established
[80]. The same principles apply to the treatment and secondary prevention of thromboembolic events in patients with MM. Management should also be disease-specific and patient-tailored. In cancer patients, there is a gradual transition from the use of LMWH to the use of DOACs, as data regarding the safety and efficacy of these agents are accumulating
[81][82][83][84]. It should be noted that hematological malignancies represent a small proportion of patients enrolled in these studies. A meta-analysis in 2894 cancer patients demonstrated superiority for DOACs in efficacy, as VTE recurrence was lower, at 5.2% compared to 8.2% for LMWH. There was, however, a non-significant increase in major bleeding events and a significant increase in non-major bleeding events
[85]. Based on current guidelines, when a VTE event occurs in patients with MM, the bleeding risk should be assessed, and patients should receive anticoagulation for at least six months
[71]. De Stefano et al.’s recently published consensus paper summarizes recommendations for treating acute thrombotic events and secondary antithrombotic prophylaxis
[9]. In the case of ongoing treatment with IMiDs, indefinite thromboprophylaxis should be considered. The long-term thromboprophylaxis should also be considered in the relapsed or high-disease burden setting until a response is achieved. The authors suggest that with regard to thrombocytopenia, the same principles apply as for primary thromboprophylaxis. Dose-adjusted LMWH or VKA is most appropriate for patients with severe renal insufficiency. DOACs can be considered for patients with mild or moderate renal insufficiency based on each drug’s summary of product characteristics.
6. Conclusions
The main challenges in the current management of thromboembolic complications in patients with MM are (1) the suboptimal performance of the risk stratification tools that are currently available and (2) the fact that the use of thromboprophylaxis is not routinely incorporated for all patients with MM in every day clinical practice. In the clinical trial setting, despite adequate thromboprophylaxis, patients on lenalidomide-based regimens still have a substantial risk of VTE of around 6%, indicating either that risk assessment models have poor discriminatory power or that the use of thromboprophylaxis is not optimal. There is also data to support that in the real-world setting, patients often receive inappropriate thromboprophylaxis based on baseline risk stratification and that the approach is usually not guideline based.
To summarize current recommendations, all NDMM should have a baseline risk assessment concerning thrombotic complications using available tools. The baseline VTE risk should be weighed against the hemorrhagic risk in a patient-specific manner. The final choice of the agent used for thromboprophylaxis should be tailored to each patient. Finally, the baseline risk should be reevaluated throughout the disease course as it is an evolving process and pharmacological thromboprophylaxis should be adapted accordingly. Disease status (relapse or remission), performance status and patient mobilization, platelet count and renal function are a few of the factors that need to be reassessed at regular intervals. Aspirin should be reserved for very-low-risk patients. For other patients, given that there is no contraindication, a choice should be made between LMWH and DOACs. Figure 2 presents an algorithm for VTE prevention in MM patients.
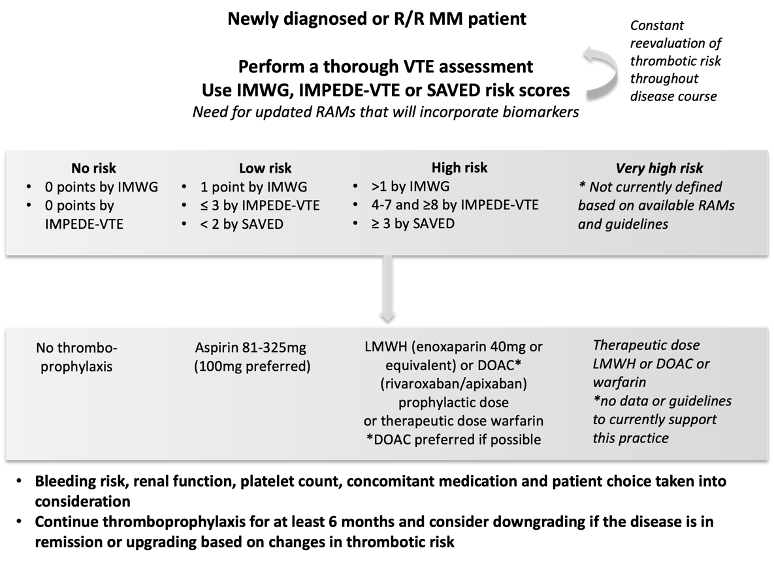
Figure 2. Algorithm for the assessment of VTE risk and choice of thromboprophylaxis. R/R: relapsed/refractory, MM: multiple myeloma, IMWG: international myeloma working group risk score, RAMs: risk assessment models, LWMH: low molecular weight heparin, DOAC: direct oral anticoagulant
Unfortunately, advances in the management of thromboembolic complications in patients with MM in recent years have not followed the evolution of the other anti-myeloma therapies, and thromboembolism remains a significant complication. To overcome the unmet need for thromboprophylaxis optimization, we need to address the gaps in existing knowledge. First, we need to understand better the procoagulant state observed in MM patients, the contribution of individual risk factors and the interplay between the disease, patient and treatment-specific prothrombotic mechanisms. Second, we need to identify biomarkers and develop tools that accurately reflect the thrombotic risk in MM patients. Incorporating coagulation biomarkers in the current risk assessment tools seems to be a potentially useful tool. Third, we need clinical trials that will provide robust data on the safety and efficacy of different modes of thromboprophylaxis, using risk-assessment tools to stratify patients. Finally, we need to increase the implementation of international guidelines in everyday clinical practice.