1. EOC Removal Technologies for Municipal Wastewater
Depending on the processes that may be involved, EOC removal technologies have been classified into three categories: physical, biological, and/or chemical processes (
Figure 1). Over the last three decades, the main trends in PhACs removal were reported by Taoufik
[1] through a systematic mapping study. Taoufik
[1] concluded that adsorption was the most frequent process of PhACs’ removal from the water phase, followed by photodegradation and biodegradation. Meanwhile, these authors also found that systems based on chemical processes were the most studied
[1]. Some of the most applied physical processes use sorbents, such as activated carbon, or membrane technologies, such as nanofiltration, ultrafiltration, or reverse osmosis
[2][3][4]. Chemical processes include AOPs such as photolysis, ozonation, Fenton or photocatalysis, among others, and chemical precipitation. However, the use of these physical and chemical technologies involves significant electricity consumption, investment, and operational costs.
Figure 1. Main treatment methods for EOC removal from municipal wastewater.
Biological systems, on the other hand, were gaining popularity as several studies demonstrated their effectiveness in the degradation of many PhACs
[5]. In addition, the use of biological technologies implies lower operating costs. Biological treatment of MW is due to the coexistence of different microenvironments that allow physical, chemical, and biological processes to take place. In terms of EOC removal, several studies support the effectiveness of biodegradation through systems such as: conventional activated sludge (CAS) in WWTPs, membrane bioreactors (MBR), sequencing batch reactors, trickling filters, or CW
[6] (
Figure 1). However, the complete removal of EOCs presented in MW by the above-mentioned technologies operating individually remains a challenge. Therefore, the combination of different technologies (e.g., hybrid systems based on biological+chemical, biological+physical, or biological+chemical+physical processes,
Figure 1) emerged as the most effective approach to try to overcome the shortcomings of each specific treatment and attempt a global removal
[2].
The ranges of EOC removal efficiencies in WWTPs based on the reviewed literature. EOC removal efficiencies in conventional WWTPs typically range from 20–50% during primary treatment, 30–70% in systems that include primary and secondary treatment, and over 90% in systems that reach tertiary treatment
[7]. EOCs that exhibit hydrophilicity (i.e., log D
ow < 1) were generally not well removed during primary treatment. Nevertheless, many hydrophobic EOCs (i.e., log D
ow > 3) showed a tendency to adsorb strongly onto primary sludge and were therefore partially removed from the dissolved phase after primary treatment
[8][9][10]. In fact, Martín
[8] observed that only 3 of the 16 studied EOCs were not detected in the sludge, while 11 of them were identified even in the final compost from sewage sludge.
Through biological processes (i.e., anaerobic and/or aerobic systems), certain EOCs can be degraded to a greater or lesser extent, leading to complete or partial mineralization and potentially generating by-products. In addition to sorption and biodegradation, volatilization may also contribute to the removal of volatile EOCs from the water phase.
2. EOC Removal by Sorption
The main mechanisms involved in the biological degradation of EOCs are sorption on sludge particles and aerobic or anaerobic biodegradation
[11].
Sorption on sludge particles can occur through two mechanisms: absorption and adsorption. When the hydrophobic EOCs pass from the aqueous phase into the lipophilic cell membrane of the biomass, this is referred to as absorption. If the positively charged EOCs are retained by electrostatic interactions on the negatively charged surface of the sludge particle, adsorption takes place
[12][13]. In general, sorption on EOCs is related to their physicochemical properties (log K
ow, log D
ow, pK
a, and k
d). Tiwari
[11] previously estimated the sorption of pharmaceutical compounds in sludge from their log K
ow values and concluded that log K
ow and pK
a determine the affinity of EOCs to undergo sorption, so that EOCs with log K
ow > 3.5 (i.e., lipophilic compounds) are prone to sorption onto sediments. For example, BPA with log K
ow = 3.64 (
Table 1) would have a high affinity for sorption.
The most relevant factors in terms of EOC sorption in sewage sludge are biomass composition and concentration, hydrodynamic parameters, and the use of sorbents
[12]. Compared to granular biomass versus flocculent biomass, Alvarino
[14] found that EOCs were absorbed more efficiently in flocculent biomass than in granular biomass due to a higher specific surface area available and lower mass transfer resistance. However, sorption was more important in UASB digesters than in CAS because of a higher concentration of accumulated biomass (7–30 g VSS/L in UASB vs. 1–2 g VSS/L in CAS).
In addition, EOCs with a low affinity to be retained in sludge may have a high affinity to interact with some adsorbents, such as activated carbon. For example, Alvarino
[3] obtained 0% and 30% removal for DCL and CBZ, respectively, under biological treatment. It should be noted that DCL and CBZ (with 1 > log Dow < 3,
Table 1) usually behave as recalcitrant to the biodegradation process. However, these authors improved the removals of DCL and CBZ to more than 80% and 90%, respectively, by adding activated carbon in a MBR. In contrast, these authors highlighted the progressive saturation of the active carbon, requiring new doses.
Table 1. Physicochemical properties of EOCs under review according to the references consulted on biological wastewater treatment processes a.
Name Acronym Chemical Composition |
CAS MW b Formula |
pKa a |
log Kow b |
log Dow c |
kbio (L/gSS·d) d |
kd (L/kgSS) d |
Acetaminophen ACE
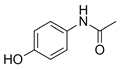 |
103-90-2 151.17 C8H9NO2 |
9.5 |
0.46 |
0.9 |
58–240 |
1.5–1160 |
Ofloxacin OFL
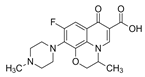 |
82419-36-1 361.37 C18H20FN3O4 |
5.97 9.28 |
−0.39 |
- |
0.01–0.0933 |
12,000–22,100 |
Caffeine CAF
 |
58-08-2 194.19 C8H10N4O2 |
10.4 |
−0.07 |
−0.55 |
0.48–156.24 |
<30–140 |
Carbamazepine CBZ
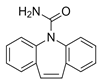 |
298-46-4 236.27 C15H12N2O |
13.9 15.96 |
2.45 |
2.77 |
0.005–0.389 |
<8–314 |
Ketoprofen KET
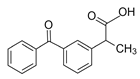 |
22071-15-4 254.28 C16H14O3 |
4.45 |
3.12 |
0.39 |
0.24–3.36 |
0.24–226 |
Ibuprofen IBU
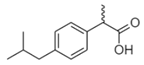 |
15687-27-1 206.28 C13H18O2 |
4.85 |
3.5 3.97 |
- |
3.24–38.7 |
6–103 |
Diclofenac DCL
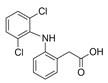 |
15307-86-5 296.15 C14H11Cl2NO2 |
4.2 |
4.98 |
2.26 0.86 |
0.02–8 |
1.9–321 |
Clofibric Acid ACB
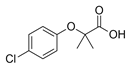 |
882-09-7 214.64 C10H11ClO3 |
−4.9 3.2 |
2.57 |
-0.42 |
0.03–1 |
7–87.5 |
Bisphenol A BPA
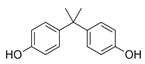 |
80-05-7 228.29 C15H16O2 |
9.6 |
3.32 |
4.05 |
0.24–16.56 |
314–505 |
Sotalol SOT
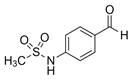 |
3930-20-9 272.37 C12H20N2O3S |
8.3 |
0.85 |
- |
- |
- |
3. Biodegradation
Biodegradation is influenced by the concentration and chemical structure of EOCs. In addition, the type of metabolism would be determined by the concentration of the compound, while the degree and rate of biodegradation would be determined by the activity of the biomass
[6][12]. Co-metabolism is the predominant mechanism in the degradation of EOCs contained in wastewater, due to the relatively low concentration of EOCs and the high concentrations of other substrates, such as easily biodegradable organic matter, acetate, ammonium, or nitrate. During co-metabolism, persistent EOCs are transformed into biodegradable intermediates within the overall metabolic pathways. A well-known example of co-metabolism is the nitrification process
[19]. Ammonium oxidizing bacteria contain the enzyme ammonium monooxygenase, which is responsible for the degradation of certain EOCs
[20]. For instance, an improvement in the degradation of recalcitrant ACB, CBZ, and DCL compounds was obtained by Tran
[21] by increasing the ammonium concentration (from 20 mg to 200 mg NH
4+-N/L) in a nitrifying activated sludge reactor compared to a CAS. Meanwhile, these authors found a high removal of IBU in the presence of allylthiourea (an ammonium monooxygenase inhibitor) and concluded that heterotrophic bacteria were also able to degrade IBU.
Aerobic metabolism and co-metabolism, on the other hand, appeared to be preferable to anaerobic or anoxic routes
[22]. The redox potential and chemical structure of EOCs could also determine the metabolic pathway of degradation. According to Alvarino
[12], most of the EOCs were transformed under aerobic conditions (such as IBU), and globally these authors, observed that aerobic systems were more efficient for a broad group of EOCs. However, due to their chemical structure, certain EOCs, such as naproxen or sulfamethoxazole, show higher degradation under anaerobic conditions
[12]. These authors also highlighted that the combination of different redox conditions could be a feasible alternative to improve EOC removal efficiencies.