4. Tilled and Untilled Soil States
The natural state, untilled lands attain steady physio-structural and bio-mechanical stability and functioning due to complex biological aggregation, accumulation of bio pores, particle stabilization, and organic residue binding and enmeshing. This is promoted by ecological activity, soil bound organic carbon, and embedded plant roots that reuse and preferentially exploit existing biological hotspots, such as bio pores
[25]. Moreover, the untilled natural state biologically formed soil conglomerations and aggregates are pedogenetically embedded within the structure of the soil matrix and become ecologically stable, biochemically active, and edaphically rich. However, natural untilled structural state of soil is highly fragile and can easily be disrupted by an instance passage of a heavy tractive device or tillage implement.
In the converse, a different ill-defined structure results from machine-induced perturbations in tillage of arable soils due to soil tooling, tractive wheeling, and trailed tandem traversing. The hazy structure undergoes seasonal soil throws, removal, movement and displacement, vertical and lateral layer mixing, forward and backward ruptures, cut edge soil flows, upheaval, and topsoil burial. As such, tilled soil structure is characterized by mechanical fragmentation and subsequent coalescence, consolidation, and integration of loose soil fragments that deter the edaphic functionality and crop-oriented goals of soil
[20].
4.1. Dynamics of Soil Properties in Tillage
Due to the spatial temporal, heterogeneous, and multivariate characteristic of soils, researchers have reported variable and multifaceted response of soil properties while trying to characterize soil processing in tillage. For instance, Kahlon
[26] investigated infiltration characteristics, ρ, porosity, Ksat, and penetration resistance and reported numerous effects of varying degrees as a consequence of soil tooling. However, there has been an overwhelming consensus to some extent on the influence of tillage and its principal effects on some constitutive characteristics of a healthy soil in agricultural farmlands, as exhibited by the resultant cropping characteristics. According to Ahmad et al.
[27], there was an inverse relationship between ρ and concentration and uptake of NPK nutrients, dry matter accumulation, grain yield, and press wheel penetration resistances. Unlike the inherent characteristics the dynamic physio-mechanical properties were among the most sensitive to tillage and soil-tool-interactions. However, due to limited empirical data and due to soil and climate variabilities, the temporal changes of physio-mechanical properties of soil that result from specific arable management practices under soil tillage are poorly understood. For instance, there was increased
[28], decreased
[29], and no effect
[30] on Ksat from NT compared to intensive tillage systems. Moreover, several studies have reported improved
[31], reduced
[32], and no effect
[33] of soil water holding capacity under NT systems. In a separate work, Hu et al.
[34] conducted a short-term study on dynamic behavior of soil characteristics as affected by tillage and penetration impedance under silt loams. Due to temporal changes, there were significant (p < 0.01) effects on interactions between tillage with time for all soil properties
[35] indicating that the effects were changing with time. According to Hu et al.
[34], there was a significant deterioration of soil physical quality by decreased macro-porosity, available soil water content (θ), and Ksat due to soil compaction in tillage. Further, there are reports that the topsoil (0–10 cm) was quite susceptible compared with subsurface soils (10–20 cm) in connection to characteristic changes and physical soil degradation irrespective of tillage practice
[36]. More studies on the dynamics of soil properties by Hu et al.
[34] showed that although penetration resistance effects were short lived, other soil physical characteristics varied significantly for every two adjacent measurement periods.
Most of the soil tillage studies have focused on the dynamic properties of soils and their changes in relation to the inherent characteristics and with land use management practices and tillage methods. Researchers have reported the need to consider temporal effects on, for instance, hydraulic properties when representing soil processes and modeling agricultural systems. This is because tillage effects, say for instance, compaction, gradually disappeared with time due to natural recovery of physical properties over time to their original undisturbed structural states. Some of the responses of plant rooting systems to mechanical impedance have been associated with morphological modifications viz. decreased root sizes, radial increments, and swelling of the cortex and sloughing of the cap cell
[36]–
[37]. However, different plants responded differently to temporal and spatial changes to impeded external impedance pressure, thus, there was no generalized and universal root morphological response to externally applied mechanical impedance pressure. Further studies response of root anatomy and plants rooting biomaterial viz. cell wall structure and root tissue responses to soil mechanical properties are encouraged.
4.2 Soil Tillage and Induced Mechanical Impedance
There exist significant efforts among researchers to understand the effects and response of arable soils and edaphic oriented soil properties to mechanical perturbation from tractive wheeling and soil-tool interactions. Specific research has focused on establishing the effects of soil perturbation on mechanical impedance, soil characteristics and their edaphic functionality as well as crop morphological development and yield. For instance, Chen et al.
[38] reported lower root biomass in compacted soil cores. Olubanjo & Yessoufou
[39] conducted experiments to determine the wet and dry mass of the maize roots after harvest. The highest wet and dry root masses (15.7 g and 13.6 g) were, respectively, recorded in treatments with 2.0 Mpa and lowest values (7.5 g and 5.9 g), respectively, in the 5.2 Mpa compaction readings of the soil penetrometer
[39].
According to Botta et al.
[40], topsoil compaction was a more limiting factor to root growth than the subsoil compaction. Taylor & Brar
[41] reported that the root dry matter and diameter increased linearly with increase in soil penetration resistance. However,according to Chen et al.
[38], higher root biomasses were observed in un-compacted soils compared to soils with higher mechanical impedance. Kumar et al.
[42] reported incongruencies of rooting depth with penetration resistance. This was regarded and evidenced as a compensatory response of plants to increased penetration resistance that led to reduced total porosity and aeration due to mechanical impedance
[38]. Some researchers have, however, reported that mechanical impedance led to significantly increasing root biomass up to some reasonable depth
[43]. This suggests that increase in penetration resistance as a result of soil compaction may sometimes have a favorable effect on root biomass (
Figure 1). This is probable because penetration resistance may induce vigorous root proliferation and growth as evidenced in more and relatively thicker roots in bulk soils.
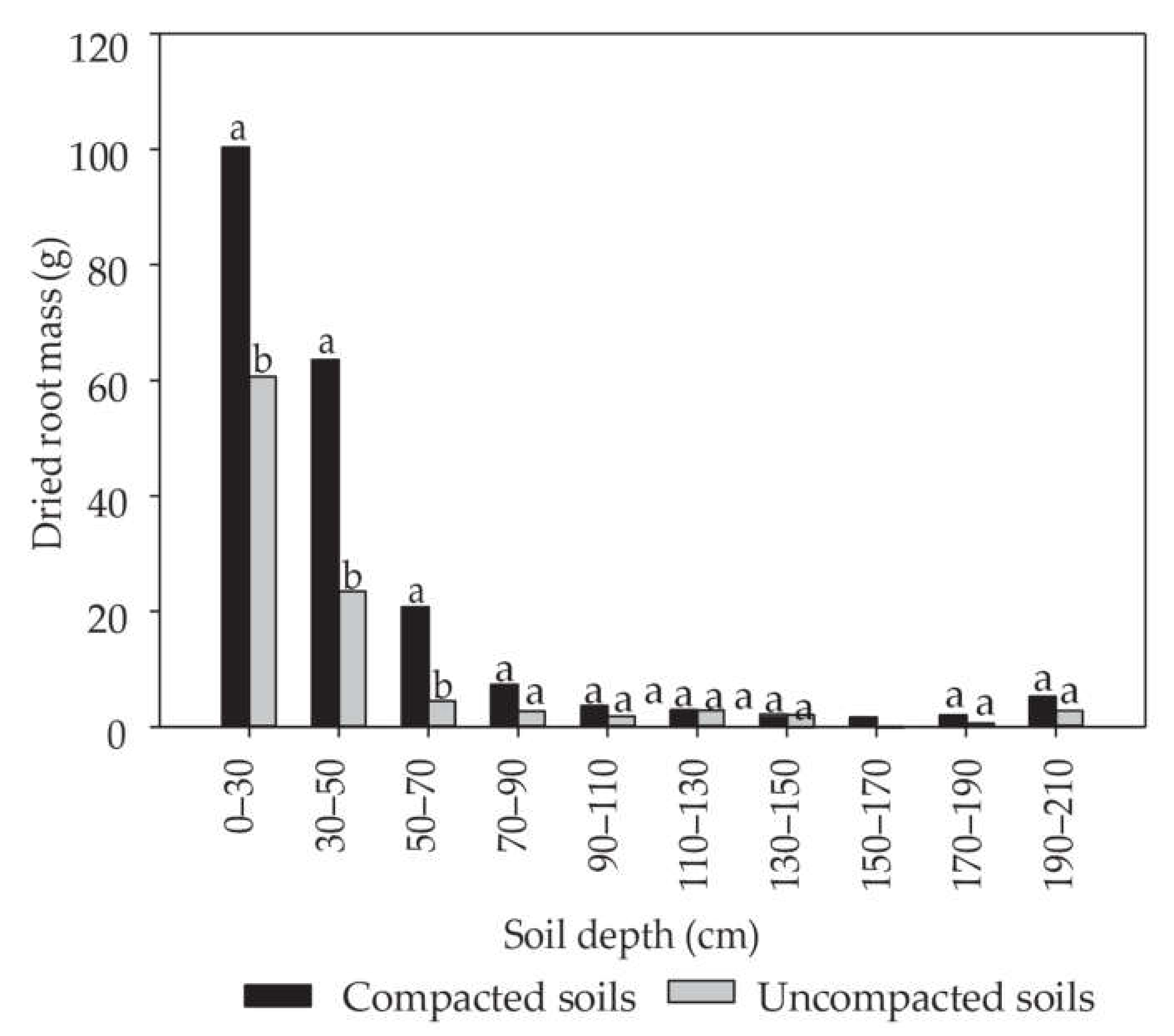
Figure 1. Rare positive correlation of soil compaction and root biomass. Different letters for the same depth indicate significant differences in root biomass (ab—Significant difference in root biomass and aa-No significant differences)
[43]
Numerous researchers have conducted studies to establish the influence of soil compaction specifically on crop development and yield. For instance, Ramazan et al.
[44] studied the impact of soil compaction on the yield of maize and reported significant results. In their initial findings Ramazan et al.
[44] established that soil bulk density was congruent with number of tractors passes. Further, the researchers established a decrease in plant height and grain yield with increase in tractor passes and concluded that soil compaction reduces grain yield
[44]. Research conducted by Ahmad et al.
[27] showed an inverse relationship between bulk density and concentration and uptake of NPK, dry matter (DM) accumulation, and grain yield. According to Ahmad et al.
[27], the compacted treatments decreased the DM accumulation in the range of 2 to 9%, whereas grain yield showed a reduction of 5 to 48%. In a separate study aimed at investigating the effect of compaction on yield of silage maize, Altuntas et al.
[45] reported that soil compaction treatments negatively affected DM yield and caused a reduction on DM yield by 16%. Further, the DM yield of silage maize was negatively affected by the press wheel compaction treatments [
53]. Conversely, while not impeding root growth, Taylor & Brar
[41] verified that values of topsoil penetration resistance between 1.03 and 5.69 MPa affected the root system morphology, reducing the crop yield by 2.581 Mg ha-
1.
Bergamin et al.
[46] studied the relationship between physical quality parameters of soil and maize yield under treatment levels of induced mechanical impedance using a randomized complete block design with five replicates and reported a significant correlation (
p < 0.01) between all soil physical attributes under study. Grain yield was positively correlated to macro porosity (r = 0.41) and negatively to penetration impedance (r = -0.42), while the emergence speed index, stem diameters, plant heights, and grain mass yield decreased with an increased mechanical impedance of soil
[46]. From their study the researchers concluded that increased soil macro-porosity and reduction of penetration resistance benefited maize yield. It was argued that soil physical attributes viz. aggregate stability, macro porosity, and penetration impedance indices were responsive and closely correlated with maize grain yields and were sensitive enough to be adopted as indicators of soil physical quality
[46].
In a different experiment Onwualu & Anazodo
[47] studied three levels of soil compaction viz. heavy, medium, and no compaction and their effects on maize production under various tillage methods in a loamy sandy soil derived from savannah zone of Nigeria. Results showed that soil moisture content and porosity decreased with an increase in soil compaction, while bulk density and soil resistant to penetrometer pressure increased. However, maize emergence increased with an increase in soil compaction, but the highest yield was obtained under medium compaction. Thus, deep tillage may not be of any special benefits when a loamy sandy soil is compacted
[47]. Similarly, Sivarajan et al.
[48] established the impact of soil compaction due to wheel traffic on corn growth, development, and yield. The yield data showed a significant difference between the soil transects, but no difference was observed between most trafficked (MT) and least trafficked (LT). A review by Kumar et al.
[49] reported decreased crop production with an increased draft of tillage operations. The dry fresh and dry weights of the plants were reduced due to a delay in growth processes caused by compaction of the soil, which ultimately led to poor yield
[49]. Igoni & Jumbo
[50] carried out field investigations aimed at predicting the effects of soil compaction on the growth and yield of maize on a sandy loam soil in tropical climates. The researchers reported a statistically significant effect of soil compaction at
p < 0.05 on the growth and yield of maize in a tropical sandy loam soil and the model prediction model correlated with experimental data up to about 99.5%
[50].
Having known the effect of reduced plant growth and yield as a result of soil resistance, Wang et al.
[51] studied the interaction of mechanical impedance with nutrient stress on wheat seedlings growth under contrasting phosphorus (P) availability. The study was carried out in a sand culture allowing for independence of mechanical impedance from water and nutrient supply
[51]. Under sufficiently supplied P, the researchers reported significantly reduced growth, shoot, root biomass, total root length, and leaf area attributed to mechanical impedance. Contrastingly, low P supply under mechanical impedance had no effect on biomass, leaf length, nodal root number, and tiller number. It was thus adverted that growth restriction by mechanical impedance depended on P supply and was significant on most plant traits to evidence a physical, structural, and nutritional interaction of arable soils
[51].
Cairns et al.
[52] screened two rice varieties for root growth under drought and irrigated treatments and reported high mechanical impedance as a more fundamental constraint on root growth than soil water availability during drought. Researchers reported that varietal ability difference for soil penetration could be very important for drought avoidance in soils
[52]. Mechanical impedance of soils developed rapidly near the surface as drought increased. As such, drying stresses and mechanical impedance nearly stopped the elongation of crop roots
[53]. Since drought led to a reduction in soil water content, which increased mechanical impedance of arable soils, researchers have emphasized incorporation of root penetration ability in developing drought resistant superior cultivars
[52][54].
Compaction free soil structure improves the ability to hold and drain water, avail nutrients, and provide aeration required by plant rooting activities. Moreover, impedance free soils ensure good water retention and drainage for plants roots to draw in all the available nutrients. According to Ahmad et al.
[27], soil compaction was the major cause of decreased crop yield. Continuous plowing at constant depth and associated tractor wheeling was the most significant cause of soil compaction that affected bulk density, porosity and root proliferation, consequently hindering nutrients availability and uptake by plants
[27]. Increased soil density inhibited root growth, even though researchers have reported that soil strength-controlled soil root penetration more than soil bulk density
[55].
Mechanical impedance of soils varies during the crop growing season and this effect and associated crop yield has been investigated under different tillage methods
[56]. Numerous researchers have reported a significant influence of soil compaction on the yield in various cropping systems. Researchers have argued that soil penetration resistance restricts deep root growth and consequently limits plant access to subsoil water and soil nutrients
[43]. Cairns et al.
[57] reported an insignificant effect of water regime compared to penetration resistance that led to physiological stress of two rice varieties.
Experimental results indicated higher mechanical impedance fundamentally constrained root growth compared to water supply during drought
[57]. Researchers revealed that varietal variations in root penetration capability could be very important for easing adverse effects and drought avoidance in mechanically impenetrable soils
[57][58][59]. However, there was little detectable effect of water regime on root distribution, but evidence of lower root numbers at depths below 20 cm in the higher penetration resistance (PR) site was revealed
[60][61].
Whereas increased intensity of mechanical soil loading reduced the total grain yield, Gronle et al.
[62] went ahead to study the effect of mechanical compaction on yield composition and reported a significant decline in crude protein content in peas and oats because of higher mechanical soil loading. Moreover, there were reports of limiting root and plant growth for bulk density values of 1.75–1.80 g cm
3 and 1.60–1.70 g cm
3 under loams and sandy loams, respectively
[63][64]. Some researchers have associated penetration impedance with critical limits for plant rooting
[65]. Cone penetrometer indexes exceeding 2–3 MPa were considered critical limits and restricted crop root development in arable soils
[62]–
[66]. However, some researchers have argued that these limits were dependent on plant species. This is because the rooting of certain crops seemed to be restricted by values above such general limits
[62].
For instance, experiments by Ehlers et al.
[67] reported that rooting in oats was limited to a penetration resistance of 3.6 and 5.1 MPa as opposed to peas (0.06–1.8 MPa). Apart from cone index, soil response to penetration impedance was found to be specific to crop species. According to Bengough & Young
[68], and as reported by Gronle et al.
[69], root elongation of peas was 55% (under 1.8 MPa cone index and 1.5 mgm
3 bulk density) of that of peas variety grown under 0.06 MPa and 0.85 Mg m
3 loamy sand.
Some of the responses of plant rooting systems to mechanical impedance have been associated with morphological modifications viz. decreased root sizes, radial increments, and swelling of the cortex and sloughing of the cap cell
[41]. Different plants responded differently to temporal and spatial changes to impeding external pressure, thus, there was no generalized and universal root morphological response to externally applied mechanical impedance pressure. However according to Mwiti et al.
[70] decades of persistent variable responses of soils to land management and conversion from natural to tilled state, is significant evidence of the adverse impact of mechanical perturbation on edaphic soil functioning.
5. Conclusions and Recommendation
Field crop rooting in arable soils suffer a combination of physio-mechanical and nutritional stresses. The edaphic factors of soil are severely impinged in tilled arable soils compared to naturally pristine conditions in untilled states. There is need for new insights on soil disturbance by integrating both physio-mechanical and ecological nutritional stresses in tillage practice. The future direction of soil disturbance in tillage and associated field scale response of crop root and yield to soil management should focus on exploiting both the physio-mechanical and biological soil health.Further studies response of root anatomy and plants rooting biomaterial viz. cell wall structure and root tissue responses to soil mechanical properties are encouraged.
The focus of soil working in tillage ought to be the soil-root system with greater interactions with soil microorganisms and minimal soil fracturing to further contribute to the quantity of carbon in the soil as opposed to conventional nutrition.