One of the most important applications of AWJ technology is AWJ milling. An efficient stream of liquid, with an increased pressure at a value of about 1300–4000 bar, is usually employed on a CNC machine, which allows the rendering of a large variety of shapes, while also guaranteeing precision and the repeatability of dimensions
[2]. This method of material processing ensures low production costs, translating into a lower price for the finished element. Although AWJ is mostly used for cutting through material, usually it is also being employed for Control Depth Cutting (CDC). This can be achieved by controlling the process parameters, namely the traverse speed (v
t), the stand-off distance (h), the abrasive flow rate (m
a), and the jet pressure (p)
[3][4][5]. The erosion process that eventually leads to the desired material removal during AWJM occurs due to the pressure of the water and the impact of the abrasive. AWJM is a time-dependent process, since the traverse feed rate is an essential parameter for controlling the Material Removal Rate (MRR). As the jet moves faster, fewer particles will strike the material, and the MRR will be lower
[6][7][8].
2. Sustainability Assessment in Manufacturing
According to Jayal et al.
[9], various manufacturing concepts related to sustainability or at least some of its elements evolved as distinct concepts around 2010 and exhibit different levels of potential. These concepts are lean manufacturing, which is mainly based on waste reduction; green manufacturing, which is based on the 3R concept; and finally, sustainable manufacturing, which is the most innovative concept, based on the 6R concept. The 3R concept, namely “reduce, reuse, recycle,” and the 6R concept, namely “reduce, reuse, recover, redesign, remanufacture, recycle,” are two of the most frequently used concepts in order to establish a more green approach in manufacturing
[10]. It is important to note that sustainability is related not only to the manufacturing stage, but to all the product life-cycle stages such as pre-manufacturing, use, and post-use as well
[9][11][12].
An important categorization of sustainability dimensions is the well-known concept of the “three pillars”, namely the economic, social, and environmental dimensions. These three dimensions are related to the aspects of economy, ecology, equity, work, and knowledge
[13]. More specifically, the first pillar is related to industry, the second is related to the human capital society, and the third one to nature
[13]. A relevant sustainability framework is termed as the “Triple Bottom Line” (TBL or 3E) and aims at the amelioration of the state of the three pillars, which will subsequently ameliorate the condition of the technological and natural ecosystems. Each dimension has a specific function
[13]; for example, the role of the economic dimension is to relate the sustainability of machining processes with financial strategies and ensure that these processes are feasible from a techno-economic point of view. The environmental dimension is related to the reduction of the negative environmental impact caused by machining processes, e.g., by decreasing energy and resource consumption. Furthermore, the social dimension includes efforts towards achieving safer workplaces, elimination of health threats, and improvement of productivity.
In some cases, the concept of the three dimensions can be expanded with additional categories and their respective indicators. For example, Jamil et al.
[14], in an assessment of the sustainability of welding processes, included the final properties of the weld as an additional category, termed physical performance, and Bork et al.
[15] related the economic pillar to a technological one in a unified “economic-technological” pillar.
In the relevant literature on conventional machining processes, another commonly accepted categorization of sustainability aspects is that of Jawahir et al.
[16], who selected the six most significant parameters regarding sustainability of manufacturing processes: waste management, manufacturing cost, environmental impact, energy consumption, personnel health, and operational safety. These six parameters or elements are interacting, and their contributions constitute the total process sustainability index
[9][17]. Waste management is relevant to recycling and disposal of wastes produced during the manufacturing processes, and it is necessary to develop means to reduce waste and its subsequent emissions and treat it properly before disposal, especially that which is toxic or otherwise harmful
[11][16]. Manufacturing cost is relevant to the costs of the equipment required for the manufacturing process and the costs during the process
[16]. However, indirect costs related to environmental or social factors can be also incorporated into the total cost
[11]. The environmental impact is related to factors which lead to environmental pollution such as toxic materials, metallic dust, and emissions. Energy consumption can be estimated by monitoring the power consumption during manufacturing processes and can be minimized by various means. Finally, personnel health and operational safety are two important aspects that are not so frequently taken into consideration in relevant studies. Workers should not be exposed to harmful mist and vapors or various chemical substances, and their workplace should be properly designed in order to ensure safety during various manufacturing processes.
Apart from the general concepts related to sustainability, it is necessary to define more specific quantities or indicators in order to evaluate the sustainability of various systems. According to Singh et al.
[18], sustainability indicators are appropriate criteria aiming to quantify the different states and dimensions of the concept of sustainability and can be divided into two different categories, namely quantitative and qualitative indicators, or according an alternative definition by Bork
[15], objective and subjective metrics. Examples of quantitative indicators or objective metrics are power consumption and carbon dioxide emissions, which can be directly measured, whereas qualitative indicators or subjective metrics are those which cannot be directly quantified, such as personnel safety and health risk
[19]. These indicators can also be quantified by appropriate scoring systems, which are used by experts in the respective field in order to assign a numerical value to each different state of a qualitative indicator. Suitable criteria should meet several requirements
[19] and can be categorized in different dimensions such as the aforementioned 3E.
Najiha, Rahman, and Kadirgama
[20] argued that there is a non-deterministic component of sustainability that also needs to be taken into consideration by fuzzy logic models. In fact, half of the aforementioned elements of sustainability, namely manufacturing cost, power consumption, and waste management, can be considered as deterministic and can be calculated by analytical formulas, whereas the other three can be considered as non-deterministic and thus can be described by fuzzy variables
[9][17].
In order to calculate the total sustainability index for a specific process, the sustainability dimensions and their indicators should be properly organized into various hierarchical levels. In the relevant literature, various alternative procedures exist regarding the number and theme of the hierarchical levels. The first level usually includes general sustainability aspects, followed by their subcategories in the second level, and more specific indicators in the final levels. In the work of Najiha, Rahman, and Kadirgama
[20], three hierarchical levels of sustainability factors used for the calculation of the sustainability index were defined, with the first one including aspects such as environmental friendliness, the second one including factors such as level of recycling, and the third one including more specific factors such as the chip recycling rate. A hierarchical structure of three levels was also proposed by Bork et al.
[15] and Kim, Leong, and Chen
[21], including a level of individual metrics, a level of sub-indices, and the level of sustainability index. The sub-index level is related to individual aspects of sustainability based on the 3E. Badurdeen et al.
[12] included five different levels from the index to the individual metric level, such as the sub-index, cluster and sub-cluster levels. In the sub-index level, the three pillars of sustainability can be considered, and then they are further analyzed in the next levels. For example, the economy can be analyzed as initial investment, costs, benefits, and losses; initial investment can be analyzed as capital, R&D, and employee training cost; and finally, capital cost is calculated based on equipment and facility costs.
An alternative categorization of sustainability aspects into different levels is relevant to the different areas of importance or different stages of production. Various researchers have included different numbers of hierarchical levels. For example, Jayal et al.
[9] included three different levels, namely product, process, and system. In each level, different methodologies can apply, and the weighting of similar indicators may be different as affects this level to a higher or lesser degree. Moreover, the sustainability index (SI) can be defined in different levels
[12], e.g., the ProdSI in the product level or the ProcSI in the process level. Especially in the product level, the 3R and 6R concepts are considered important. Feng, Joung, and Li
[22] proposed a much broader analysis including seven different levels, namely global, country, sector, corporation, facility, process, and product, whereas Kim, Leong, and Chen
[21] used five of them, excluding sector and facility. In a machining workshop
[23], the sustainability assessment process can include layers such as machine tool, manufacturing unit, manufacturing task, and workshop layer. Although a hierarchy exists between the four layers, each layer interacts with the others, and finally the energy consumption state is determined through effective energy and specific energy consumption indicators.
After the levels and the metrics in each level are selected, the sustainability assessment can be carried out. Usually, the whole procedure includes the following five steps, depicted also in Figure 1:
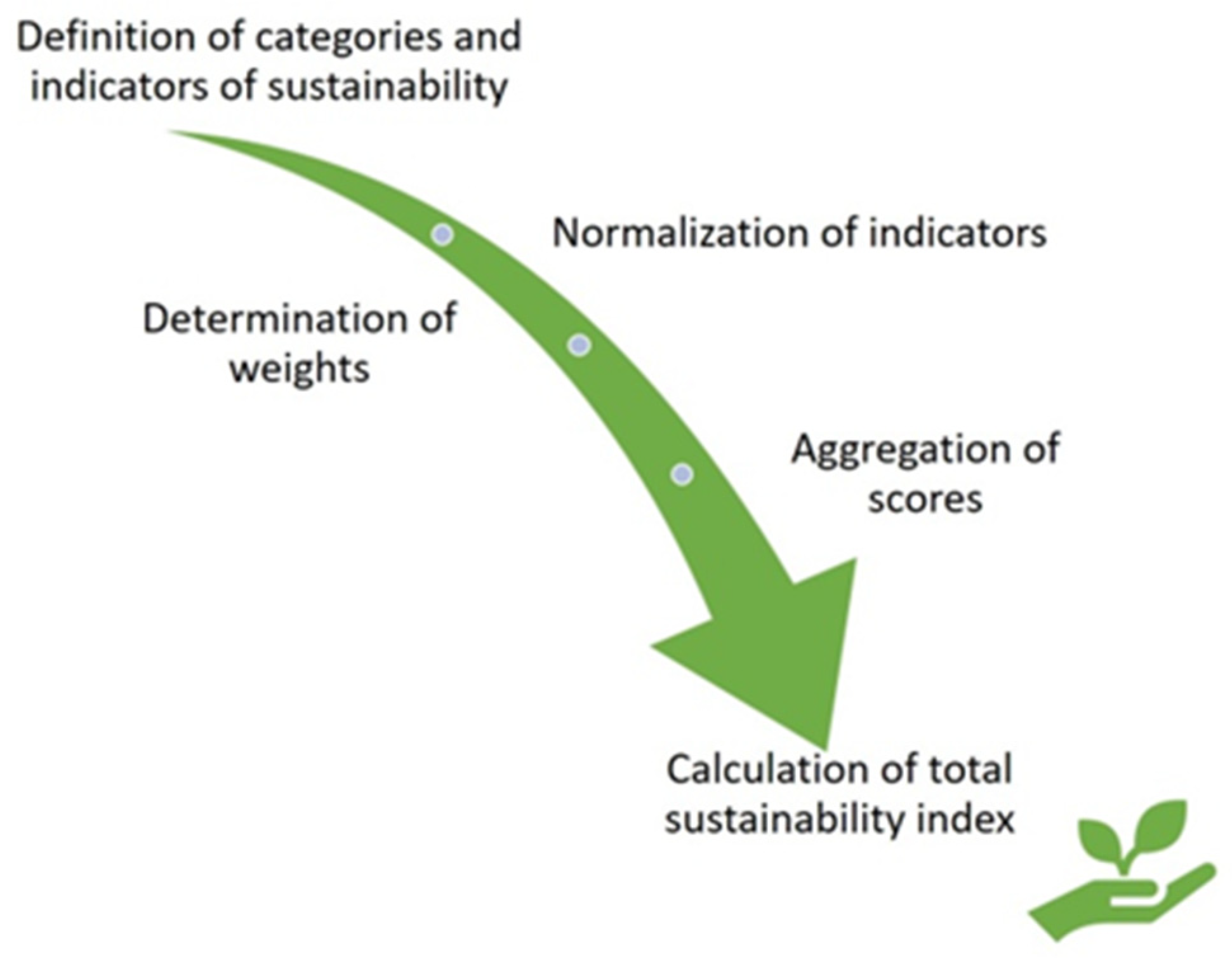
Figure 1. Sustainability assessment steps.
- (1) Categories and indicators of sustainability are defined. Indicators which are not useful or do not have a noticeable effect for the specific system are not included.
- (2) The values of all indicators are properly normalized in order to eliminate any artificially created relatively higher influence of some indicators due to their absolute values.
- (3) The indicators of each category are assigned weights according to their importance towards the final goal.
- (4) An aggregation procedure is carried out, using scores and weights of each category to calculate an overall sustainability score.
- (5) Finally, the sustainability scores of all assessed processes are compared.
In order to carry out the evaluation process or critical parts of it such as the determination of weights and aggregation of scores, various established methodologies can be used. In the relevant literature, sustainability-specific methods such as LCA (Life Cycle Assessment); general application methodologies such as TOPSIS
[24], AHP
[25], fuzzy AHP
[26], and GRA
[27]; and optimization approaches such as desirability approach or genetic algorithms
[10] are used.
3. Conclusions
Experimental tests regarding AWJM of Ti-6Al-4V were carried out in order to conduct a comprehensive evaluation of the sustainability of AWJM of hard-to-cut materials. In the experimental tests, glass beads were selected as the abrasive materials, and various important quantities such as depth of penetration, kerf width, and kerf taper angle were measured. After the results were analyzed, various conclusions were drawn.
First, AWJM of titanium alloys with glass beads was shown to be relatively effective, although this abrasive material is not as hard as common abrasives. The anticipated trends were observed regarding the depth of penetration, and jet pressure was determined to be the most important parameter, followed by traverse speed and abrasive mass flow rate. Kerf width was also affected mainly by jet pressure for constant stand-off distance values, as well as kerf taper angle. The variation of abrasive mass flow rate in all cases showed different behaviors for different ranges of values, as the depth of penetration remained almost constant for larger values of abrasive mass flow rate, kerf width increased clearly for values of ma only above 2 g/s, and kerf taper angle was reduced for values of ma up to 5 g/s.
Using GRA method, sustainability assessment was able to be carried out, taking into consideration various indicators both from the economic and environmental dimensions of sustainability. For the economic dimension, workpiece quality, productivity, and cost indicators were considered, whereas, for the environmental dimension, material and power consumption were used. The results revealed that the case with a traverse speed of 600 mm/min, abrasive mass flow rate of 0.5 g/s, and jet pressure 150 MPa provided the highest sustainability index value, leading to the best possible performance in both the economic and environmental dimensions. Moreover, it was observed that the GRA method was simple to use but also very effective towards the handling of multiple objectives, leading to the creation of a flexible framework that can be altered by adding or removing various indicators if it is required.