Pancreatic ductal adenocarcinoma (PDAC) is a lethal cancer type as it is prone to metastases and is difficult to diagnose at an early stage. Despite advances in molecular detection, its clinical prognosis remains poor and it is expected to become the second leading cause of cancer-related deaths. Approximately 85% of patients develop glucose metabolism disorders, most commonly diabetes mellitus, within three years prior to their pancreatic cancer diagnosis. Diabetes, or glucose metabolism disorders related to PDAC, are typically associated with insulin resistance, and beta cell damage, among other factors. From the perspective of molecular regulatory mechanisms, glucose metabolism disorders are closely related to PDAC initiation and development and to late invasion and metastasis.
1. Clinical Association between Pancreatic Ductal Adenocarcinoma and Diabetes
Approximately 80% of clinical patients with pancreatic ductal adenocarcinoma (PDAC) are diagnosed with weight loss that will progress to a cachectic state if left untreated
[1]. Pancreatic cancer involves the disruption of intestinal nutrient absorption and glucose metabolism by influencing endocrine and exocrine function. Approximately 85% of patients with pancreatic cancer exhibit glucose metabolism disorders, with the majority (45–67%) having been diagnosed with diabetes mellitus, typically within three years before receiving their pancreatic cancer diagnosis
[2][3]. However, the relationship between pancreatic cancer and diabetes is complex and the mechanism is unclear. Indeed, abnormal glucose metabolism is closely related to the nutritional status and prognosis of patients with pancreatic cancer.
In a 10-year follow-up of more than 90,000 people, Inoue et al. found that the incidences of pancreatic, liver, and kidney cancers were significantly higher in patients with diabetes than in those without diabetes
[4]. In another study of more than 30,000 individuals, the incidence of pancreatic cancer in diabetic patients was 2.5 times higher than that in non-diabetic patients
[5]. In this study, the incidence of esophageal and colorectal cancers was significantly higher in diabetic patients than in controls. The blood glucose range affects the incidence of different cancers to some extent, suggesting that the development of malignancies due to diabetes is caused by chronic hyperglycemia or hyperinsulinemia. Stolzenberg et al. showed that hyperglycemia, hyperinsulinemia, and insulin resistance are all risk factors for pancreatic cancer; however, the effects of these factors on the development of pancreatic cancer were not statistically significant
[6].
In a study by Mizuno et al., the median survival time for pancreatic cancer was improved by approximately 10 months (20.2 months vs. 10.2 months) in patients with new onset diabetes mellitus alone compared to patients without diabetes mellitus
[7]. This improved prognosis may be associated with the early diagnosis and treatment of pancreatic cancer due to diabetes mellitus. Therefore, the identification of new-onset diabetes is of immense clinical importance for the early diagnosis and management of pancreatic cancer. However, long-term diabetes has been found to be a poor prognostic factor for patients with pancreatic cancer, while postoperative glycemic recovery in patients with new-onset diabetes is a protective prognostic factor. Hence, glucose metabolism disorders are highly related to pancreatic cancer prognosis
[8].
Diabetes mellitus may contribute to pancreatic cancer prognosis through various potential mechanisms. First, type 2 diabetes (T2D) is often associated with insulin resistance, unstable insulin concentrations, reduced ability of the liver to inhibit inappropriate hepatic glycogen release, and reduced ability of β-cells to overcome insulin resistance. However, the causes of β cell dysfunction are complex. Typically, β cell dysfunction is caused by an elevated glucose concentration due to insufficient glucose sensing to stimulate insulin secretion. Glucose concentrations that remain persistently higher than the normal physiological range can lead to the manifestation of hyperglycemia
[9].
Moreover, diabetes may act as a contributor to pancreatic cancer development, preceding the diagnosis of cancer. This may be related to several mechanisms. First, the release of exosomes that deliver adrenomedullin to β-cells induces endoplasmic reticulum stress and perturbation of the unfolded protein response, leading to β-cell dysfunction and death
[10]. Second, inflammatory responses mediate β-cell dysfunction; the abnormal activation of oxidative stress and the unfolded protein response leads to a reduction in β-cells and subsequent apoptosis and senescence
[11][12]. Third, T2D is often concurrent with obesity, which represents another high-risk factor for pancreatic cancer
[13]. These potential mechanisms can lead to increased insulin levels in the pancreatic microenvironment and further contribute to tumor development.
Pancreatic-derived diabetes mellitus, also known as type 3c diabetes mellitus (T3cD), typically occurs secondary to hereditary or acquired pancreatic disease or following pancreatectomy. T3cD differs from traditional or T2D in that it is often caused by exocrine pancreatic injury, such as infection, acute or chronic pancreatitis, trauma, or surgery resulting in reduction of islet cells; its main symptoms include hypoinsulinemia, hepatic insulin resistance, decreased pancreatic polypeptide levels, and increased peripheral insulin sensitivity. T3cD is also associated with pancreatic cancer. Pancreatic cancer-associated diabetes has unique hormonal and metabolic features. That is, insulin levels may be mildly elevated and peripheral insulin resistance occurs, which more closely resembles T2D than T3cD of other etiologies
[14]. In cases of chronic pancreatitis, pancreatic cancer, and pancreatic resection, pancreatic polypeptide (PP) is also deficient. Indeed, the amount of PP—primarily produced in the head of the pancreas—plays an important role in pancreatic-derived diabetes. Therefore, the ADA guidelines specifically indicate that pancreatic cancer-associated diabetes differs from other T3cD in that it may occur by mechanisms other than a decrease in the number of islet β cells
[15]. The above research advances may improve the diagnostic strategy of pancreatic cancer.
2. Promotion of Pancreatic Ductal Adenocarcinoma through a High Glycolytic Phenotype
While differentiated cells rely primarily on mitochondrial oxidative phosphorylation to generate energy for physiological activities, pancreatic cancer cells rely on aerobic glycolysis, a phenomenon known as the “Warburg effect”
[16][17][18]. Aerobic glycolysis is an inefficient way to produce ATP; however, it facilitates the formation of substances required for pancreatic cancer cell proliferation, such as nucleotides, amino acids, and lipids
[19]. In this regard, hyperglycemia can provide an adequate source of glucose for rapidly proliferating pancreatic cancer cells. The aberrant expression of oncogenes/tumor suppressors (e.g., PI3K, AKT, p53, and RAS) results in increased levels of glucose transporters (GLUTs) and glycolytic enzymes at the cell membrane, as well as increased glucose uptake
[20][21]. In particular, Chikamoto et al. reported that GLUT1 is an unfavorable prognostic factor in pancreatic cancer
[22].
In PDAC, distant metastasis is typically associated with a poor prognosis
[23]. The glycolytic phenotype can promote the EMT of cancer cells, thus, enhancing aggressiveness and promoting metastasis
[24][25][26]. Induction of the glycolytic phenotype of PDAC is regulated by multiple pathways
[27]. More specifically, increases in the expression of oncogenes
[28], mitochondrial dysfunction
[29][30], and the abnormal expression or activation of glycolytic enzymes all contribute to the enhanced glycolytic phenotype in PDAC
[31][32].
Warburg noted that a deficiency in irreversible oxidative phosphorylation (OXPHOS) can lead to aerobic glycolysis. Moreover, a decrease in the OXPOHS energy supply is often accompanied by structural abnormalities in mitochondrial DNA (mtDNA)
[33][34]. Alterations in mitochondrial function caused by mtDNA mutations or copy number changes, as well as defective mitochondrial respiratory chain complexes and ROS, trigger retrograde signals from the mitochondria to the nucleus. When the mtDNA copy number is reduced, membrane potential (ΔΨm) is disrupted, which mimics Ca
2+-regulated neurophosphatase signaling and activates the upregulation of many oncogenic factors and kinases, such as IGF-1R/NF-κB/PI3K, and glycolytic enzymes
[31][32][35] (
Figure 1). In contrast, glucose restriction inhibits various energy-dependent pathways in pancreatic cancer cells, such as IGF-1/PI3K/Akt/mTOR, inhibits cell metabolism and growth, and promotes G1 phase block and apoptosis
[36][37].
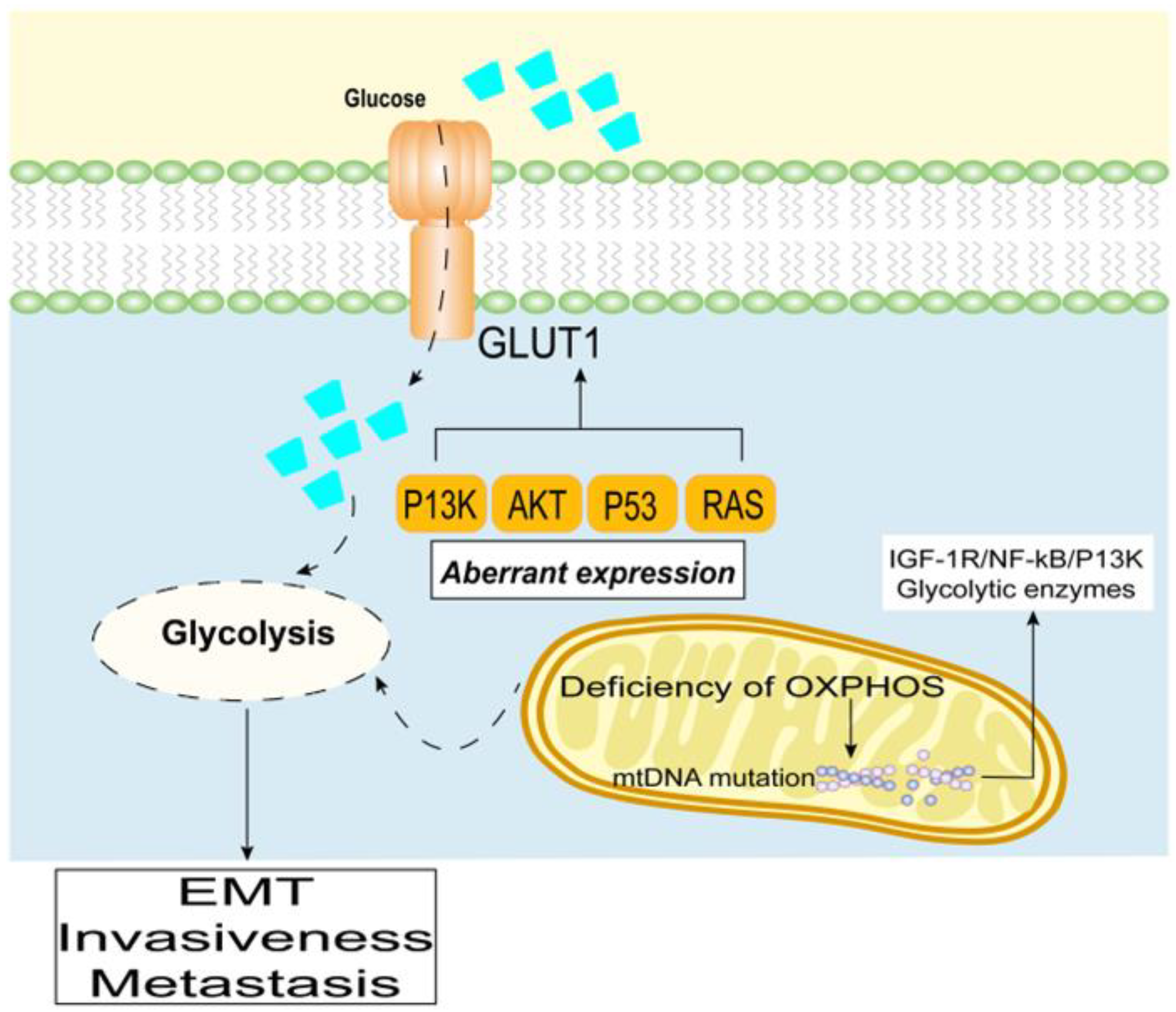
Figure 1. Promotion of PDAC through a high glycolytic phenotype.
Furthermore, fluctuations in the levels of ROS produced by mitochondria induce mtDNA mutations and enhance the metastatic potential of cancer cells
[38][39]. In fact, reduction of NAPDH oxidase expression—a specific enzyme that regulates ROS production—inhibits the glucose uptake capacity of PANC-1 cells, and attenuates tumor growth in vivo
[40][41][42].
3. Therapeutic Strategies Targeting Glycolysis
A basic approach to preventing the development of malignant tumors in patients with abnormal glucose metabolism is to prevent risk factors, i.e., to control blood glucose concentrations and to actively treat obesity, diabetes, and hypertension. Meanwhile, the risk of malignancy in the healthy population can be reduced by correcting, or improving, abnormalities in glucose metabolism by lifestyle changes, including weight loss and adoption of a healthy diet (
Table 1)
[43]. Similar results can be achieved through surgery; for example, obese patients that have undergone bariatric surgery have a lower incidence of malignant tumors than that of the control group, comprising obese patients without surgery (
Table 1)
[44].
Metformin has a strong protective effect against myriad malignancies in the diabetic population
[45]. Although most basic experimental studies have shown some degree of protection against certain malignancies (e.g., breast, colorectal, and prostate cancers), recent clinical trials and meta-analyses have yielded inconsistent findings (
Table 1)
[46]. Hence, further studies are needed to determine whether metformin can be developed as a complementary therapy to other anticancer therapies. Moreover, cardamonin has been shown to inhibit the growth of a triple-negative breast cancer cell line, MDA-MB-231, in vitro and in vivo by inhibiting HIF-1α-mediated cellular metabolism. In addition, cardamonin treatment reduces glucose uptake and lactate production and efflux, suggesting that it contributes to the inhibition of the glycolytic process (
Table 1)
[47]. Meanwhile, Yan et al. demonstrated the critical role of glucose metabolism in KRAS-driven PDAC resistant to MAPK inhibition, revealing potential therapeutic approaches to treat this aggressive disease (
Table 1)
[48].
It is important to note that glucose metabolism disorders caused by pancreatic cancer commonly result in exocrine pancreatic dysfunction. A study of 248 patients has shown that up to 92% of patients with pancreatic exocrine abnormalities, with various causes (pancreatic cancer or chronic pancreatitis), exhibit vitamin D deficiency (
Table 1)
[49]. The level of vitamin D in the body is correlated with blood glucose control. Hence, monitoring and supplementation of 25-hydroxyvitamin D levels is beneficial for treatment. The function and physiological levels of enteroglycemic hormone in normal beta cells depends on the exocrine function of the pancreas and sound lipolysis. Hence, impaired function of the enteroglycemic system plays a role in glucose metabolism dysfunction in pancreatic cancer, and the maintenance of the enteroglycemic system function may be a therapeutic target
[50].
In conclusion, the dysfunction of glucose metabolism is closely related to the development of malignant tumors, and the associations between various components of abnormal glucose metabolism and different types of malignant tumors vary. Abnormal glucose metabolism is both a risk factor for malignant tumor development and an accelerant of tumor development that inhibits tumor cell apoptosis and enhances tumor cell metabolism, proliferation, and metastasis, among other processes. Although a variety of molecular mechanisms have been investigated, conflicting experimental findings have been reported on related therapeutic approaches. For instance, basic research has shown that metformin and statins are effective for the prevention or treatment of malignancies; however, no such effect has been found in clinical trials, and the specific reasons for these conflicting results warrant further exploration.
This entry is adapted from the peer-reviewed paper 10.3390/cancers15020498