1. Introduction
Sirtuins are a group of proteins regulating many physiological functions and life processes. They have become an essential factor in research focused on aging processes as the deletion in the Sir-2 gene, which is responsible for the expression of sirtuins, results in a reduced lifespan of
Saccharomyces cerevisiae [1]. With high probability, they may be one of the many missing elements on the way to longevity, significantly since the activity of individual sirtuins in cells characteristically decreases with age
[2][3]. Geneticist Amar Klar first described sirtuins in the 1970s by discovering the Sir-2 (Yeast Silent Information Regulators II, SIR2) gene in
Saccharomyces cerevisiae cells. Still, research indicating their potential impact on longevity was carried out about 20 years later, in 1991, by Leonard P. Guarante
[4][5]. In 1999, by adding SIR2 to the DNA, it was possible to extend the replication life of yeast compared to the wild line. It was also confirmed two years later, extending the life cycle of worms. In 2003, David Sinclair showed that the activity of sirtuins could be modified by other substances, for example, resveratrol. A year later, they showed the connection of SIRT1 with FOXO proteins and proved their neuroprotective effect
[6][7][8]. In the following years, there was further research on sirtuins, compounds modulating their activity, bioavailability, and their connection with metabolic functions (
Figure 1). The focus was on classifying them and analyzing the mechanisms of their impact on various organisms, which, according to many scientists, may be crucial in determining them as regulators of aging
[9][10][11].
Figure 1. Timeline of sirtuin history
[4][5][6][7][8][9][10][11].
2. General Characteristics of Sirtuins: Classification, Structure, and Localization
Sirtuins are NAD+ cofactor-dependent histone deacetylases (class III–HDAC). Seven mammalian sirtuins have been identified so far (SIRT1 to SIRT7)
[4][12]. The catalytic domain of the NAD+ sirtuins contains 258 amino acid residues on average (the shortest is attributed to SIRT6 of 239 amino acid residues, while the longest is to SIRT2–275 amino acid residues composing)
[13][14]. They occur in both bacteria and complex organisms
[15] and take part in many processes regulating biological functions (cell cycle, cellular metabolism, genome homeostasis)
[4][16][17][18]. It is considered that sirtuins can prevent DNA damage, the formation of cancer cells, and inflammatory reactions. They play a crucial role in the antioxidant defense
[19][20]. In the human body, sirtuins are present in many different organs. From the level of the liver and kidneys, they can be involved in reducing stress and inflammatory reactions
[21][22] because they have a unique role in the production of cytokines
[18]. Assigning individual types of sirtuins to organisms is difficult since data on their presence in specific species are unclear or incomplete and constantly updated. Bioinformatics analysis and spatial visualization of the sirtuin particle show that a sirtuin’s N- and C-terminal regions may be unique within a species. Thus, the catalytic activity and dynamics may differ in unrelated species
[23][24]. The current classification of sirtuins depends on the sirtuin location in the cell and is still updated through new research. Three main localization sites have been determined: cytoplasm, mitochondrion, and cell nuclei (
Figure 2)
[18].
Figure 2. Intracellular localization of mammalian sirtuins
[2][25][26][27][28][29][30].
Nuclear sirtuins are histone-modifying deacetylases and are responsible for gene expression. These include SIRT1, SIRT6, and SIRT7, but there are reports of SIRT2 and SIRT3 that can migrate between organelles. Their mission is to determine in the cell when aging will begin
[18][31], and probably have cytoprotective activity
[32]. In turn, mitochondrial sirtuins are also called imported mitochondrial sirtuins (mtSIRT) with an N-terminal sequence
[33]. Mitochondrial sirtuins include: SIRT3, SIRT4 and SIRT5. Regulation of their function occurs in post-translational modification of substrate proteins through deacetylation, demalonylation, lipoamidation, and poly ADP-ribosylation
[12]. They can modify and regulate oxidative stress in cells and reduce reactive oxygen species directly in the organism
[34][35]. Mitochondrial sirtuins reduced expression may contribute to the disturbance of the body’s homeostasis, ultimately leading to the formation of pathologies and diseases related to the aging process, mainly affecting organs such as skeletal muscles, liver, pancreas, heart, or bones
[31][36][37]. Cytoplasmic sirtuins coordinate the processes associated with cell apoptosis and DNA repair
[38]. Sirtuin 2, deacetylating α-tubulin, is mainly located in the cytosol
[39]. Sirtuin 1, on the other hand, is most found in the cell nucleus but can migrate and be present in the cytoplasm
[25][40]. It regulates the activity of proteins responsible for chromatin structure, holds the cell cycle, and maintains homeostasis
[41]. Explicit determination and understanding of the biological role of sirtuins are difficult due to their function in many biochemical pathways and the lack of inhibitors directed against specific types of sirtuins. Current studies are based on presumptions, defining newly discovered compounds as potential or promising activators
[42][43].
2.1. Sirtuin 1 (SIRT1)
Sirtuin 1 is the human homolog to Sir2, derived from
Saccharomyces cerevisiae (yeast)
[26]. It is localized in the cell nucleus, but numerous reports in the literature about its occurrence in the cytoplasm
[4]. It is the best-tested and well-known sirtuin so far
[27]. SIRT1 affects insulin secretion from pancreatic beta cells, oxidation, fatty acid synthase induction, transcriptional activity, and deacetylation of histones and non-histone proteins
[28][29][30]. It may inhibit inflammation, produce pro-inflammatory cytokines, have anti-apoptotic effects, and increase resistance to cellular stress. Moreover, it inhibits the p53 protein
[44][45] and helps to maintain organelles integrity (peroxisomes and mitochondria)
[46].
2.2. Sirtuin 2 (SIRT2)
Sirtuin 2 plays an essential role in oxidative stress protection, reducing its intensity through the deacetylation of FOXO and Nrf2 transcription factor
[17][47]. It interacts with the protein p53, histone H4 lysine 16, and α-tubulin
[48] and acts as a mitotic checkpoint protein that prevents the formation of hyperploid cells in early metaphase
[49]. SIRT2 is also an epigenetic regulator because its dysregulation affects tumor progression (its expression is reduced in reproductive, urinary, or digestive systems cancer)
[50][51]. Park et al. (2021) showed that SIRT2 has functions in lipid metabolism in mice, activating hepatocytes and hepatic stellate cells
[52]. Furthermore, it is associated with cell proliferation and neurological disorders
[53]. Jeong and Cho (2017) state that SIRT2 can regulate neuronal differentiation through the deacetylation of α-tubulin but the mechanisms of this process are not clarified
[54].
2.3. Sirtuin 3 (SIRT3)
The primary location of sirtuin 3 is the mitochondria. However, it can migrate to the cell nucleus or cytoplasm
[55]. It regulates lysine acetylation in mitochondria
[56]. In humans, it affects the stabilization of heterochromatin and cytosol
[31][57]. Some studies indicate that it appears in the mitochondrion only due to cellular stress, moving there from the cell nucleus
[58]. Sirtuin 3 activates the mitochondrial genes PGC-1a and UCP-1, which suggests its essential role in thermogenesis
[59]. It is associated with the longevity gene in men
[60] and may prevent bone loss in mice by regulating E11/gp38
[61]. According to Liu et al. (2022), SIRT3 activation might reduce the anti-inflammatory effect by weakening NF-κB signaling
[62]. SIRT3 is a kind of mitochondrial protector that can prevent cardiovascular diseases because it participates in their metabolism and dynamics by regulating the acetylation of mitochondrial proteins
[63]. Its connection to cardiovascular diseases was also demonstrated by Liu et al. (2021) in studies on the development and regression of atherosclerosis. SIRT3 participating in acyl modifications maintains mitochondrial homeostasis and prevents vasculitis. However, there is scarce information about its use as a therapeutic against atherosclerotic lesions, which suggests the need for further research
[64].
2.4. Sirtuin 4 (SIRT4)
Jaiswal et al. (2022) reported that SIRT4 is localized only in the mitochondrial matrix
[57]. It is known that due to its location, it does not participate directly in DNA repair, but it shows strong enzymatic activity against oxidative stress generated during cellular respiration. It can protect the genome indirectly by lowering glutamate dehydrogenase activity and arresting the cell cycle. It participates in mitochondrial ATP homeostasis and, with other sirtuins, in stem cell differentiation
[57]. Aside from its mitochondrial role, further enzymatic activity is puzzling.
Studies indicate its function in the oxidation of fatty acids in the muscles and liver and regulating insulin secretion. It probably also plays the role of a mitochondrial tumor suppressor
[65]. SIRT4 is little known and described in vertebrates
[66][67]. He et al. (2022) suggested that it may inhibit doxorubicin (DOX)-induced cardiotoxicity by regulating the AKT/mTOR/autophagy pathway
[68] or prevent the release of pro-inflammatory cytokines IL-1β, TNF-α and IL-6
[69].
2.5. Sirtuin 5 (SIRT5)
SIRT5 is classified as mitochondrial and regulates metabolic pathways such as the Krebs and fatty acid cycles. Probably SIRT5 polymorphisms result in shortened human lifespan
[34]. Sirtuin 5 affects brown adipose tissue activity as it can regulate mitochondrial respiration and protein kinase activity after treatment with the sirtuin inhibitor MC3482
[70]. It can catalyze deglutarylation, demalonylation, and desuccinylation reactions while exhibiting low deacetylation activity
[42][71]. Jung et al. (2022) showed the influence of SIRT5 on mitochondrial respiration, and the TNF-α factor induces its expression, contributing to the delaying of the aging process
[72].
2.6. Sirtuin 6 (SIRT6)
Sirtuin 6 has broad molecular functions and is a critical factor in the aging process due to its increased activity in the cell
[73]. It is most often found in the cell nucleus. However, there are many reports of its occurrence in the cytosol
[31]. It is related to glucose and lipid metabolism in the liver and pancreas via FoxO1
[74]. Research conducted on SIRT6 a few years earlier indicated its genetic instability, and some studies even proved that it caused premature aging processes. SIRT62/2 mice die prematurely and exhibit severe defects such as lymphopenia, loss of subcutaneous fat, reduced bone mineral density, and impaired glucose homeostasis. These are similar pathologies to those occurring in seniors
[75]. Then, it was shown how its overexpression affects the extension of the life of mice
[3]. According to Carreno et al. (2020), age-related diseases may lead to oxidative DNA damage. Sirtuin 6 may prevent these processes while stabilizing the genome and serving as a diagnostic biomarker
[76][77]. SIRT6 has become one of the leading research subjects, thus diverting attention from SIRT 1 due to its more significant therapeutic potential, especially in neurodegenerative diseases
[2].
2.7. Sirtuin 7 (SIRT7)
It is the only sirtuin located in the nucleolus, is involved in stress response and ribosome biogenesis, and is the least described among the sirtuins
[78]. Sirtuin 7 interacts with RNA polymerase (Pol I) and histones to regulate rDNA transcription (transcript elongation). Its inhibition induces a decrease in transcription, stops cell proliferation, and initiates apoptosis
[79]. Presumably, it may also inhibit cell apoptosis under stress conditions (e.g., hypoxia)
[78]. According to Li et al. (2022), sirtuin 7 may inhibit the NF-κB signaling pathway, activated after entering an external factor into the body, resulting in the inhibition of inflammation caused by inflammation lipopolysaccharides
[80]. SIRT7 activity is associated with the pathology of the cardiovascular system in the form of heart failure, atherosclerotic changes, and myocardial hypertrophy, thus is considered a potential therapeutic target against cardiovascular and kidney diseases
[81][82]. There have also been reports that it may affect mitochondrial activity
[83].
2.8. Sirtuin 8—Myth or Fact?
There are few reports on the discovery of SIRT8 expression. Sun et al. reported its activity in thyroid cancer, which was later contradicted by classifying this sirtuin as SIRT7
[84]. Other studies on the
Locusta migratoria genome showed that this species has a higher amount of enzymes affecting the modification of histones compared to other insects and indicated the presence of a gene similar to SIRT3 in mammals (regulation of mitochondrial fatty acid oxidation). It was proposed to create a new subfamily for SIR2-Sirt8, but from the experiment until 2022, no new classification was recorded due to the lack of subsequent independent analyses
[85].
2.9. Sirtuins and Invertebrates
Sirtuins are mainly described in vertebrates, where mice (
Mus musculus) are a significant research object. So far, it has not been possible to identify sirtuins in invertebrates fully, and the information, sometimes contradictory, relates only to selected species. It was found that insect sirtuins are significantly different from mammalian ones. Research conducted on
Caenorhabditis elegans confirmed the presence of the SIR2 (Sir-2.1) ortholog, which increases the viability of individuals by almost 50%. It depends on the transcription factor Daf-16 and is regulated in the insulin/IGF-1 pathway
[86]. Other results concern
Drosophila melanogaster. It has been described that the dSir2 gene does not play a significant role in the viability of individuals but is only a regulator of heterochromatin formation. Individuals with a mutation in the dSir-2 gene are not characterized by a shorter life cycle like it is in worms
[87]. Shukla et al. (2022) proved that the SIRT6 protein in
D. melanogaster can potentially slow down larval development
[88]. Other studies on this species have shown that SIRT4 has a regulatory effect on oxidative metabolism and may promote longevity. Overexpression of SIRT2 in muscles and neurons enables restoration of exercise capacity in
Drosophila with mitochondrial dysfunction
[89][90][91][92]. Wang et al. (2021) identified in
Ruditapes philippinarum five types of sirtuins: SIRT1, SIRT2, SIRT4, SIRT6, and SIRT7, the expression of which depends on the developmental stage of the individual and environmental conditions. They play a role in restoring homeostasis after exposure of individuals to air
[15]. Zhang et al. (2022) showed that in
Bombyx mori (silkworm), SIRT2 and SIRT5 could enhance antiviral immunity, and the SIRT5 inhibitor (suramin) promotes nucleopolyhedrovirus replication
[93]. Three years earlier, in 2019, Brent et al. reported inhibition of arbovirus genetic replication in adult flies treated with SIRT1 and SIRT2 inhibitors, which may contribute to a more efficient fight against diseases transmitted by forceps and mosquitoes (yellow fever, dengue fever, West Nile fever)
[94]. In turn, May et al. (2021) presume that sirtuins play a role in regulating heat shock in
Mytilus californianus (Conrad). However, determining the role of specific sirtuins in regulating this process requires more precise and targeted research
[95].
3. Modulators of Sirtuin Activity
Due to the participation of sirtuins in the aging process, determining which compounds have the most effective effect on the modulation of their activity is an urgent issue
[96]. The mutually antagonistic mechanisms of sirtuins initiated by proper modulators can prevent many different diseases and thus become a valuable therapeutic agent. Information on sirtuin activators and inhibitors is still unclear, which has changed and even become mutually exclusive over the years. The action mechanism of these compounds is vague, and the different strengths directed against other members of the sirtuin family show that they do not target only one conserved enzyme
[97], making their classification difficult. For example, suramin, initially considered a synthetic inhibitor of SIRT1, has also shown inhibition of SIRT5 through virtual screening data. Due to its lack of specificity and chemical multifunctionality, it is not considered for further research
[97][98]. Sirtuins engage in many metabolic pathways, so changing their activity may affect one area positively but another negatively. For example, inhibiting sirtuin activity by sirtinol causes platelet aggregation and causes thrombocytopenia, but at the same time may be beneficial in cancer therapy
[99]. Sirtinol, similarly to suramin, acts non-specifically by inhibiting SIRT2 and, to a lesser extent, SIRT1
[100].
Studies on the use of sirtuin activators focus on resveratrol (RV), which is found as a flavonoid in food and beverages
[101][102][103]. Quercetin and fisetin, as natural polyphenols structurally similar to RV, also have many health benefits and the ability to activate SIRT1. Unfortunately, all of them have been shown to have low bioavailability, which is insufficient for clinical use. Moreover, their metabolites may be pharmacologically active
[76][104][105]. In recent years, (until 2022), there has been a noticeable increase in interest in synthetic activators and inhibitors of sirtuins that can support natural modulators in action. Several effective synthetic SIRT1 activators have been developed that mimic the health benefits of resveratrol (SRT1720, SRT1460, SRT2183) and are in clinical trials in combination with other drugs
[104].
Gozelle et al. (2022) used compounds based on 5-benzyl-1,3,4-thiadiazole-2-carboxamide, obtaining effective sirtuin 2 inhibitors-ST29 and ST30, which may also potentially support the treatment of cancer
[105]. Additionally, Laaroussi et al. (2022) indicated indole analogs EX-527 as cytotoxic to cancer cells and many times more efficient than nicotinamide and other mentioned inhibitors
[97][99][106].
Table 1 summarizes the latest sirtuin modulators with effects on selected model species.
Table 1. Summary of studies (from 2017 to 2022) on sirtuin activators and inhibitors (1–7).
3.1. Resveratrol—An Antidote for Aging?
Resveratrol (RSV) (3,5,4-trihydroksystylben) (
Table 2) is a natural chemical compound of plant origin (phytoalexin). It is most found in the skin and seeds of
Vitis vinifera grapes. It occurs in the form of two geometric isomers -cis and -trans, where only the trans isomer shows biological activity. In red wine, the concentration of resveratrol ranges from 0.1 to 15 mg/L and is 3–10 times higher than in white wine
[137]. Resveratrol has a broad, positive physiological effect, but it remains in cells for a limited time. Therefore, its effect may not be sufficient
[138]. Due to its nephroprotective effect in diabetes patients, it may reduce the risk of its occurrence. RV is toxic when an inappropriate therapeutic dose is used
[139]. It is noted that resveratrol may inhibit L1-RTP, which in turn is dependent on SIRT1, SIRT6, and SIRT7. An increase in SIRT6 was observed after the use of resveratrol as a therapeutic agent
[140]. RV in mice increases LSK ex vivo and reduces T cell proliferation in vivo and ex vivo
[18]. In addition, its neuroprotective effect is constantly being assessed. Recent research shows that it mediates multiple molecular pathways related to aging and central nervous system function. It contributes to beneficial epigenetic changes that last for generations. It prevents cognitive impairment and cell neurodegeneration primarily due to its potent antioxidant properties
[141]. In addition to scavenging free radicals, it modulates synergistic pathways responsible for anti-inflammatory and anti-apoptotic effects
[142]. Demonstrated reduced neuroinflammatory stress with decreased pro-inflammatory cytokines (TNF-α) in rats. Moreover, after experimental central nervous system injury, RSV can ameliorate functional deficits when administered on an ad hoc basis
[143]. Studies also indicate the inhibitory effect of resveratrol on the activity of SIRT1, SIRT3, and SIRT5, depending on the substrate used
[42].
3.2. Polydatin—An Improved Resveratrol?
Polydatin (PD) (
Table 2), the glycosidic form of resveratrol isolated from
Polygonum cuspidatum is considered more effective because its concentration in plants can be up to 15 times higher than resveratrol. It is found in wine, grapes, and peanuts
[84][144] in two forms, cis- and trans-. Trans-polydatin is attractive due to its anti-inflammatory and antioxidant properties
[145]. It has a protective effect against numerous diseases. Like RV, it is promising in treating many diseases of the nervous system due to its neuroprotective effects (Alzheimer’s disease, Parkinson’s disease, brain, and spinal cord injuries, strokes). The polydatin mechanism works through several pathways Nrf2/Keap1/ARE, PI3K/Akt, ERK/MAPK, TLR/NF-κB/TNF-α/ILs or Bax/Bcl-2/caspases Oral administration of polydatin reduces malondialdehyde (MDA) production and increases antioxidant activity (SOD, CAT)
[146]. Tong et al. 2020 showed an effective reduction in cognitive impairment caused by chemotherapy. Doxorubicin-induced stress was reduced by activating the NF-κB pathway and reducing apoptosis
[147]. Bao et al. (2022) recognized polydatin as an effective agent in liver damage by NF-κB signaling
[148]. Jin et al. (2022) showed that polydatin could increase body weight in mice while reducing insulin resistance
[149]. It is considered a potential therapeutic agent in case of oxidative stress and radiation-induced lung damage (SIRT 3 activation)
[150][151]. Zhang et al. (2017), based on their research and associations with SIRT3 expression, suggested its use as a drug against diabetic cardiomyopathy
[152]. Moreover, by upregulating SIRT1 expression, PD may prevent mitochondrial dysfunction and stimulate IRT mRNA. Unfortunately, polydatin show pharmacological disadvantages such as low solubility, low plasma concentration, or rapid chemical degradation, which makes therapeutic applications difficult
[153].
3.3. Honokiol—An Effective Antioxidant
Honokiol (HKL) (
Table 2) is a polyphenol extracted from the bark and leaves of magnolia (
Magnolia officinalis). Classified as an antioxidant and is traditionally used in treating inflammatory diseases
[154]. Recent studies indicate its effectiveness against SARS-CoV-2 by inhibiting furin activity and blocking the function of S-protein (spike)
[155]. Due to the ability to cross the blood–brain barrier and the blood-cerebrospinal fluid barrier is characterized by significant bioavailability. Studies on a mouse model by Zhou et al. (2022) report its effectiveness in the treatment of neurodegenerative diseases and the ability to improve mitochondrial function and antioxidant properties. It exerts therapeutic effects in several neurological diseases, including amyotrophic lateral sclerosis (ALS), in both in vitro and in vivo models. It improves the viability of NSC-34 motoneuron cells, characterized by mutated G93A SOD1 proteins, and the morphology of mitochondria in SOD1-G93A cells. This effect has also been shown in the spinal cord. Moreover, honokiol extended the lifespan of SOD1-G93A transgenic mice and improved their motor function
[156]. As a GABA modulator and CB1 agonist, it is being considered for treating mood disorders. In the treatment of depression and anxiety disorders, it has a selective anxiolytic effect
[157]. By SIRT1 expression, HKL modulates endoplasmic reticulum stress and promotes cell viability
[156][158]. HKL applied to lung cancer cells destabilizes Hif-1a, induces apoptosis and arrests the G1 phase. Its anticancer properties are regulated by the SIRT3/Hif-1a pathway
[159]. Activating SIRT3 alleviates cardiac cell impairment
[160]. Li et al. (2016) showed its effectiveness in increasing the activity of SIRT3 in the smooth muscles of the mouse liver and reducing its steatosis
[161]. Moreover, the use of honokiol in older mice stopped bone loss, and the effectiveness was dose-dependent
[61].
3.4. Triclosan-Synthetic Sirtuin Activator
Triclosan (TCS) (5-chloro-2-(2,4-dichlorophenoxy)phenol (
Table 2) is a synthetic chemical compound with broad bacteriostatic (in higher concentrations also bactericidal), antifungal, and antiviral properties. Due to its properties, it is often used in cosmetics, and it was overused during the COVID-19 pandemic, which raised concerns about environmental safety because it shows high bioavailability through the skin and oral administration
[162][163]. Excessive exposure to triclosan may contribute to thyroid homeostasis disorders, metabolic disorders, cardiotoxicity, and increased cancer risk
[163][164][165]. It has been proven that triclosan in rodents can impair the immune response and contribute to liver diseases. At environmental concentrations, it modulates the transcriptional response of Nrf2, SIRT1, and SIRT2 in the livers of
Gambusia affinis fish, negatively affecting their antioxidant system
[166]. Szychowski et al. (2022) showed that short and long triclosan exposure increases the expression of SIRT1 and SIRT3 in neurons of the neural cortex of mice, which initially increased the level of neurosteroids in the cells, and then, despite further stimulation of the aryl hydrocarbon receptor (Ahr), caused their significant decrease
[165][166]. So far, there is little information in the literature about triclosan and its effect on sirtuins, which requires further research to explain its action mechanism.
3.5. Cambinol–SIRT 1 and SIRT 2 Inhibitor
Cambinol ((5-((2-hydroxynaphthalen-1-yl)methyl)-6-phenyl-2-thioxo-2,3-dihydropyrimidin-4(1H)-one) (
Table 2) is a β-naphthol derivative with an anticancer effect, defined as a sirtuin 1 and 2 inhibitor
[167][168] because it inhibits NAD-dependant deacetylases to reduce cell survival under stress. It has anti-inflammatory effects and is involved in the immune response and metabolic control
[169]. Chowdhury et al. (2020) identified cambinol as cytotoxic against cancer cells in vitro
[170]. Its inhibition of sirtuins 1 and 2 have been shown to increase p53, FOXO3a, and Ku70 acetylation; however, its effect’s complete mechanism is unknown
[171]. Its inhibitory effect is related to the simultaneous inhibition of histone 4 and NAD+
[172]. There are papers on more effective inhibitors than cambinol and better selectivity for SIRT 1 and 2, but further studies in this area are required
[173].
3.6. EX-527—A Precursor of Modern Sirtuin Inhibitors
EX-527 (6-Chloro-2,3,4,9-tetrahydro-1H-Carbazole-1-carboxamide) (
Table 2) as an indole compound is a matrix for synthesizing sirtuin inhibitor analogs. It is mainly used in studies of physiological and cancer cells
[100][106]. Nikseresht et al. (2019) showed the relationship of EX-527 microinjections with the reduction of cerebral ischemic infarcts and proved that by inhibiting SIRT1, they act as an inhibitor of necroptosis in an animal model
[174]. According to Kundu et al. (2020), it protects the organism against the effects of a high-fat diet (diabetic nephropathy), as it contributes to lowering blood glucose levels and reducing SIRT1 activity while increasing SIRT 3 action in the kidneys
[175]. In addition, inhibiting SIRT1 also increases renal allograft survival in mice, which may be related to T cells
[176]. It inhibits the activity of SIRT1 100-fold more strongly than SIRT2 and SIRT3, which is presumably associated with NAD+ as a colligand in the binding of EX-527 in cells
[100]. Kumari et al. (2015) pointed out that it may cause thrombocytopenia as a side effect in research on anticancer therapy, suggesting while sirtuins are involved in platelet aging and the general aging processes
[99].
Table 2. Characteristics of selected sirtuin modulators.
Modulator |
Chemical Formula |
Effect |
Characteristic |
Reference |
Resveratrol |
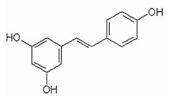 |
activator |
- -
-
biological activity in neurogenic diseases;
- -
-
affects the CNS;
- -
-
neuroprotective effect;
- -
-
antiapoptotic effect;
- -
-
anti-inflammatory effect;
- -
-
modulates the action of the antioxidant system;
- -
-
reduces nicotine genotoxicity in D. melanogaster by activating sirtuins.
|
[138][177] |
Polydatin |
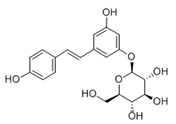 |
activator |
- -
-
liver protection and anti-apoptosis;
- -
-
neuroprotector in chemiotherapy
- -
-
anti-cancer and anti-inflammatory effects;
- -
-
inhibition of oxidative stress and removal of ROS;
- -
-
prevents diseases of the cardiovascular and nervous systems.
|
[84][147][152] |
Honokiol |
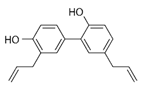 |
activator |
- -
-
regulates the process of acetylation of mitochondrial proteins through SIRT;
- -
-
therapeutic agent against obesity by activating SIRT3 in cells.
|
[154][156][161] |
Triclosan |
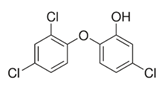 |
activator |
- -
-
antiviral and bacteriostatic effect;
- -
-
widely used in industrial products;
- -
-
toxic to the environment;
- -
-
induces the expression of SIRT1 and SIRT3 in mouse neuronal cells;
- -
-
modulates the expression of SIRT1 and SIRT2 in Gambusia affinis liver.
|
[178][179][180] |
Cambinol |
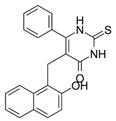 |
inhibitor |
- -
-
inhibition of SIRT1 and 2 activity;
- -
-
inhibition of immune responses and inflammatory reactions;
- -
-
reduces the activity of NF-κB, which is indirectly dependent on sirtuins.
|
[167][168][169][181] |
EX-527 |
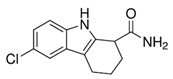 |
inhibitor |
- -
-
inhibitor of SIRT 1 and 2, cytotoxic effect in tumor cells;
- -
-
protective effect in diabetic nephropathy;
- -
-
reduces blood sugar in a rat model;
- -
-
inhibitor of necroptosis in animal model (by inhibiting SIRT1)
|
[106][174][175] |
This entry is adapted from the peer-reviewed paper 10.3390/ijms24010728