Zinc–iron redox flow batteries (ZIRFBs) possess intrinsic safety and stability and have low electrolyte cost. ZBRFB refers to an RFB in which zinc is used as the electrochemically active substance in the electrolyte solutions. The zinc electrode has a reversible anode potential. Zinc ions are stable in both alkaline and acidic environments, even in a neutral electrolyte, and the electrochemical reaction rate is relatively fast.
1. Introduction
As a result of the depletion of fossil fuels, the concerns over energy sustainability and environmental issues are given a more and more vital position [
1,
2,
3,
4]. The power generation of renewable energy, for instance, solar and wind energy, will surely become the main energy sources of future energy strategy. However, the unique intermittence and instability of renewable energy have brought major challenges to the stable operation of the power system, opening temporal and spatial gaps between the consumption of the energy by end-users and its availability, thus, energy storage technology is an effective means that can help achieve stable and efficient renewable energy [
5,
6,
7]. Compared with physical techniques (e.g., pumped storage), secondary batteries with higher flexibility have gradually attracted people’s attention [
8,
9,
10]. Among the various battery techniques, redox flow batteries (RFBs) have proved to have considerable development potential in large-scale energy storage as a result of their long lifetime, high safety, and high-energy efficiency [
11,
12,
13,
14].
According to the electrolyte used, the RFB system mainly includes vanadium-based RFB, iron-based RFBs, zinc-based RFBs, organic RFBs, polysulfide-based RFBs, etc. [
9,
15,
16,
17,
18,
19,
20,
21,
22]. To date, the vanadium RFB (VRFB) has become the most mature large-scale energy storage technique, which is suitable for large- and medium-sized energy storage scenarios [
23,
24,
25,
26,
27]. VRFBs have characteristics whose energy efficiency (EE) and cycle life exceed 80% and 200,000 cycles, respectively [
28,
29,
30,
31]. However, for VRFBs, the cost of vanadium electrolyte accounts for approximately 60% of the battery cost, which greatly increases the initial investment threshold [
32]. In VRFBs, the adopted acidic electrolyte is prone to corrode the components of the stack, and the choice of the membrane is quite restricted. At present, the Dupont Nafion
® is mainly used due to its outstanding chemical stability and proton conductivity. Nevertheless, the high cost of Nafion and vanadium makes VRFBs an expensive energy storage technique among various RFBs.
2. Characteristics of ZIRFB
2.1. The Basic Principle of ZIRFB
ZIRFB has the general characteristics of RFBs. That is to say, the ZIRFBs mainly use the changes in the redox state of active substances in the solutions on both sides of the Fe-based cathode and Zn-based anode to realize the charge–discharge process. A ZIRFB is mainly composed of a stack and two electrolyte storage tanks [
45]. The electrolyte is stored in a storage tank outside the stack, and then is transported to the inside and outside of the stack by the pump. The redox reaction occurs at the electrodes, and the reactive species flow back to the external storage tank with the electrolyte. The cathode and anode are separated by a separator/membrane, which can optionally allow the supporting electrolyte to pass through to maintain electrolyte balance. The separator/membrane not only separates the half-cells and avoids the cross-mixing of active species, but also provides the required ionic conductivity accompanied by the electrons transfer during the charge–discharge process.
2.2. Wide pH Range
Metallic Zn can be electroplated in four various ways with different forms of reactants in the solution depending on the pH from 0 to 16. The relevant reaction equations are as follows:
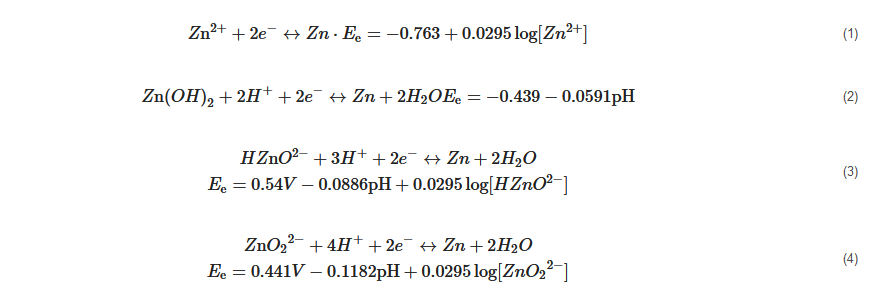
Unlike other RFBs, the electrolyte of ZIRFB can work in a wide pH range. A higher pH value is conducive to the dissolution and deposition of metallic Zn, despite that the Fe2+/Fe3+ redox couple tends to precipitate more easily at high pH. Hence, the appropriate pH range is very important. According to the difference in electrolyte acidity and alkalinity, ZIRFBs are normally divided into three types: alkaline, acidic, and neutral ZIRFBs.
In alkaline ZIRFB, zinc and ferricyanide are used as active substances in the anolyte and catholyte, respectively [51]. The system possesses the electrolyte with relatively low cost and high open-circuit voltage (OCV) of 1.74 V. In the discharge state, the anode side is transformed from Zn to zincate solution (alkaline), while the cathode side ferrocyanide is formed from the previous ferricyanide. When charging, it is the opposite process, which is a reversible reaction compared to the discharge process. However, the cycle performance of the ZIRFB is poor due to the issue of zinc dendrites in the alkaline medium.
In theory, the acidic ZIRFB (Ecell = 1.53 V) can have a higher energy density [54]. However, in the acidic ZIRFB, the excessive acidity of the solution will affect the deposition of zinc and the hydrolysis of the Fe2+/Fe3+ pair, thus, the hydrogen evolution reaction (HER) is prone to occur. For an acidic system with HAc/NaAc as the buffer solution to keep the pH value of the negative electrolyte between 2–6, a high CE (coulombic/current efficiency) can be realized [53].
Compared with alkaline and acidic systems, the neutral ZIRFB system (Ecell = 1.43 V) is mild and non-corrosive, which has lower requirements for the membrane/separator and other components [44]. The neutral ZIRFB has a lower battery cost than the other two systems, to a certain extent. Nevertheless, regarding the neutral ZIRFB system, it also has to be taken into account that the hydrolysis of Fen+ ions may lead to the decline of battery cycle performance, which is one of the primary challenges for this type of battery.
2.3. Zinc Dendrites
In comparison to other battery systems, for instance, lead-based and lithium-based batteries, the capacity/energy/power of the liquid–liquid RFBs can be designed independently [
45,
74,
75]. In fact, the ZIRFB is a kind of “half-RFB”. In the electrode reaction, the iron-based active substance on the cathode side is always present in ionic form, while the zinc-based active substance on the anode side is under the plating–stripping process of zinc. This indicates that the power and capacity of the ZIRFBs are not devised flexibly in comparison with the liquid–liquid RFB because the capacity of the ZIRFB is restricted by the surface area of the electrode during the plating–stripping process [
36].
The essential problem during the plating-stripping transversion is that the zinc dendrites mainly formed during battery charging. The existence of zinc dendrites can easily lead to problems such as a reduction in battery coulombic efficiency (CE) and capacity, and the shortening of battery life. In severe cases, it will impale the separator/membrane and lead to a battery short-circuit.
The primary reason is that Zn dendrites are more grievous when the operating current density is high. Under higher current densities, the concentration of zincate or Zn
n+ in the electrode interface area is extremely low, as the transfer rate of zincate or zinc ions in the electrolyte is obviously slower compared to the reaction rate on the electrode. This may bring about severe concentration polarization [
36]. Furthermore, the diffusion of zincate/Zn
n+ tends to realize on the protrusions of the electrode compared to the flat surface, making it easier for zincate or zinc ions to undergo a plating process on the protrusions, and further results in the generation of Zn dendrites. Due to the presence of severe zinc dendrites at high operating current densities, ZIRFB usually operates at relatively lower current densities.
2.4. Fe(III) Hydrolysis
The hydrolytic reactions of Fe
3+ are much stronger than those of Fe
2+ and, consequently, hydrolysis occurs at a much lower pH. There are few reliable investigations of the hydrolytic reactions of Fe
2+ because of both the low solubility and its propensity to be oxidised to Fe
3+, which can greatly interfere with the ability to measure Fe
2+ hydrolysis reactions. There have been several investigations that have examined the hydrolytic reactions of Fe
3+, particularly that of the monomeric species, FeOH
2+. It is surprising, therefore, to find that a substantial amount of conjecture remains which concerns the stability of the Fe
3+ hydrolytic species and phases [
80]. The hydrolysis reaction of Fe
3+ can be described by Reactions (5)–(7) [
80]. Similar to standard hydrolysis reactions, the interaction of Fe
3+ with water takes place in several stages. Firstly, the iron cation reacts with water.
The resulting product will continue to bind to another water molecule.
This entry is adapted from the peer-reviewed paper 10.3390/batteries8110202