Magnetic nanoparticles (MNPs) have great potential in various areas such as medicine, cancer therapy and diagnostics, biosensing, and material science. In particular, magnetite (Fe3O4) nanoparticles are extensively used for numerous bioapplications due to their biocompatibility, high saturation magnetization, chemical stability, large surface area, and easy functionalization. This paper describes magnetic nanoparticle physical and biological properties, emphasizing synthesis approaches, toxicity, and various biomedical applications, focusing on the most recent advancements in the areas of therapy, diagnostics, theranostics, magnetic separation, and biosensing.
Nanotechnology combines various areas of science. The small sizes of nanomaterials possess unique chemical, physical, and biological properties. To date, many nanomaterial types have been described, and many more will be developed for various applications. Magnetic nanoparticles have great potential in biochemistry, nanomedicine, and bio-inspired material areas [
1,
2,
3,
4,
5,
6,
7,
8]. One of the most promising magnetic nanoparticles is iron oxide (II, III) due to their ferrimagnetism [
1,
9,
10,
11]. In particular, Fe
3O
4 magnetite nanoparticles (MNPs) have demonstrated a promising effect in numerous applications [
1,
2,
3,
4,
5,
6,
7,
8].
MNPs have become a vital tool for material science, biochemistry, diagnostics, magnetic drug and gene delivery, hyperthermia, magnetic resonance imaging (MRI), and theranostics [
1,
3,
4,
5,
7,
9,
12,
13,
14,
15,
16,
17,
18,
19,
20,
21,
22,
23,
24,
25,
26,
27,
28,
29,
30]. The manipulation of MNPs by an external magnetic field is essential for bioseparation and biosensing areas [
1,
6,
17,
30,
31,
32,
33]. Moreover, magnetic transport of MNPs to the tissue allows targeted therapy and diagnostics (theranostics) applications [
1,
2,
3,
4,
5,
6,
7,
8,
34]. A combination of possible local heating (hyperthermia), anticancer drug delivery, and monitoring by MRI or other imaging technology open the tremendous potential for cancer treatment [
1,
2,
3,
4,
5,
8,
11,
16,
21,
35].
Many papers about the synthesis, coating, and applications of MNPs have been reported [
1,
2,
3,
4,
5,
6,
7,
8,
26,
34,
35]. The number of articles with the key term “magnetic nanoparticles” increases every year (
Figure 1). The actual number of papers in the area is much higher, which can be calculated using other keywords. However, before 1996, less than 100 articles per year were published annually by the Scopus database. After the first successful clinical trial in 1996, the number of papers greatly increased. Since 2013, more than 5000 manuscripts have been published annually. Such results are associated with the increasing recognition of MNPs in achieving excellent results in various applications.
Figure 1. The number of articles published per year in PubMed (Medline) and Scopus databases under the search phrase “magnetic nanoparticle”. The lower number of papers in 2022 is because the literature search was conducted in September 2022. An upward trend is expected for 2022. PubMed comprises biomedical literature from the MEDLINE database and life science journals. Scopus database is the largest abstract and citation database, which covers much more than PubMed scientific journals, books, and conference proceedings.
MNPs show high field irreversibility, high saturation field, and superparamagnetism, which are highly dependent on particle size and surface coating. The relationship between MNP size and magnetism (coercivity) has been extensively reported [
36,
37,
38,
39,
40]. The coercivity gradually increases for bulk nanoparticles to a maximum value at a particular size. In this region, the magnetization is stable and nonuniform (Weiss domains, magnetic multi-domain state). The critical size of the magnetite nanoparticles, above which they become multi-domain, has been theoretically calculated and is 76 nm and 128 nm, respectively, for cubic and spherical nanoparticles [
38]. However, the experimental data indicate that the critical size of transition between single- and multi-domain magnetic structure highly depends on the crystal structure and coating [
37,
38,
41]. By reducing the size of the nanoparticles, the coercivity rapidly decreases to zero, reaching a superparamagnetic state [
36,
37]. Superparamagnetism is especially important in applications such as drug delivery and imaging. Particle sizes below 20 nm are required to achieve superparamagnetism for magnetite MNPs. Superparamagnetic MNPs provide a stronger response to external magnetic fields than simple MNPs. Frenkel J. and Doefman J. in 1930 predicted that, below a critical size, MNPs would consist of a single magnetic domain [
42]. However, superparamagnetic MNP synthesis was achieved half a century later.
Research on nano-emulsion began in 1943 (
Figure 2, historical timeline) [
43]. However, the nanotechnology concept was first proposed in 1959 by Richard Feynman in the lecture entitled “Plenty of Room at the Bottom”. This was a historical event in nanoscience. The first synthesis of iron nanoparticles by gas condensation was achieved in 1981. The concept of using magnetic forces for enhanced therapeutic and imaging performance has evolved over the years. Two of the milestones were the development of MNPs for imaging purposes in 1990 and silica-coated MNPs in 1995. Since 2000, numerous studies have investigated the potential applications of MNPs and nanocomposites with magnetic cores. Another essential development was the successful magnetic hyperthermia clinical trials in 2010. Magnetic hyperthermia utilizes MNPs that are exposed to an alternating magnetic field to generate heat in local regions [
23,
44]. Magnetic hyperthermia therapy was first proposed much earlier, in 1957. However, about fifty years were required to synthesize stable and non-toxic MNPs with optimal physical properties. Colloidal stability, biocompatibility, and toxicity studies are crucial for in vivo application. Recently, numerous MNP-based “Smart” nanocomposites with pH-stimuli-responsive drug release, theranostics, and multimodal constructions have been developed [
5,
8,
13,
24,
25,
45,
46,
47,
48,
49]. Numerous research papers have focused on the possible procedures for MNPs synthesis, coating, drug-loading, toxicity studies, and clinical trials [
1,
2,
8,
20,
44,
50,
51,
52,
53,
54].
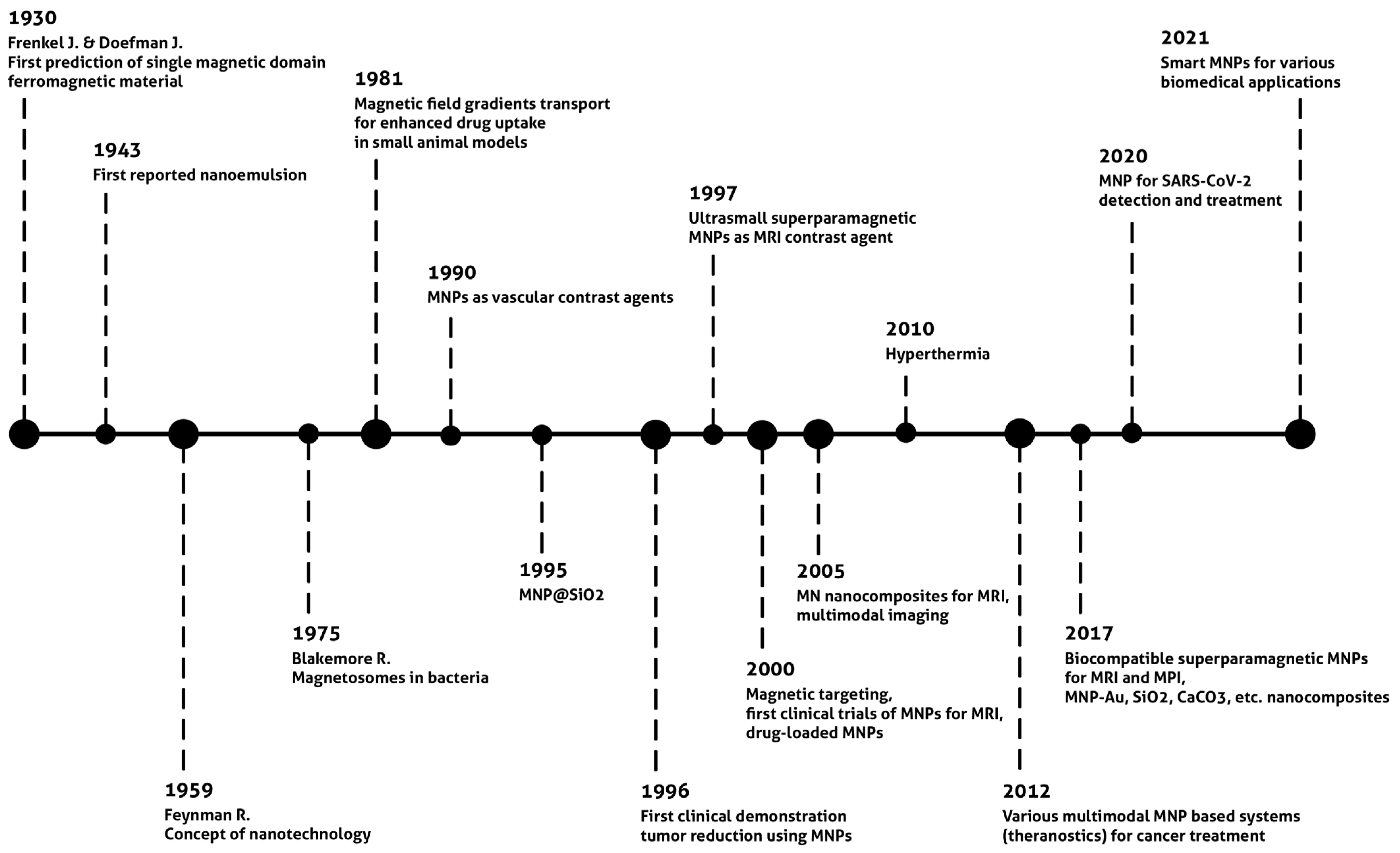
Figure 2. Historic timeline for development of MNPs.
This entry is adapted from the peer-reviewed paper 10.3390/encyclopedia2040125