One Health describes the importance of considering humans, animals, and the environment in health research. One Health and the 3R concept, i.e., the replacement, reduction, and refinement of animal experimentation, shape today’s research more and more. The development of organoids from many different organs and animals led to the development of highly sophisticated model systems trying to replace animal experiments. Organoids may be used for disease modelling in various ways elucidating the manifold host–pathogen interactions.
1. Introduction
The concept of ‘One Health’ has become increasingly important over the last few years. In contrast to specific scientific disciplines such as human medicine, veterinary medicine, or environmental sciences, One Health is an approach taking more than one of these factors into account
[1]. This also includes the political implications of the surveillance of diseases and the prevention thereof and not only scientific research on pathogens and their interaction with host organisms. COVID-19, for instance, is a very prominent and current example. SARS-CoV-2 infections are diagnosed in humans as well as many different species of animals
[2], and viral particles can be found in wastewater
[3]. Referring to the global problem of SARS-CoV-2 infections for humans, animals, and environmental contamination, one can appreciate the importance of One Health in a global context. In vitro research methods can neither fully model these complex interactions nor entirely replace animal experimentation but are of great importance to reduce the need for animals in today’s research.
More than 60 years ago, researchers were looking for ways to reduce pain and distress for laboratory animals. In 1959, Russel and Burch first explained the principle of the three Rs (3R), i.e., Replacement, Reduction, and Refinement of animal experimentation
[4]. Since then, the 3R principle has been implicitly included in animal welfare laws in the United States of America
[5] as well as in Europe
[6], and researchers are obliged to consider these laws when planning and carrying out experiments involving live animals. Recently, the Max Planck Society for the Advancement of Science e.V. has taken the next step and expanded the classic 3R principle to the 4R principle, also taking ‘Responsibility’ into account. Researchers commit to using their knowledge in order to further promote animal welfare by engaging in public discourse, improving the social structure of housed experimental animals and expanding the knowledge about the experience of pain, intelligence and consciousness in animals
[7]. Animal experimentation is not limited to laboratory mice and rats but also includes other vertebrates such as fish, rabbits, cats, dogs, pigs, and others.
Dogs, for instance, are mainly used for toxicology studies. In the European Union, the number of dogs used for any scientific purpose for the first time accounted for 17.711 in 2018, adding up to 25.717, including dogs already in use
[8]. By far, the number is exceeded by the United States, with them having used 58.511 dogs for research in 2019
[9]. These numbers clearly demonstrate the need for replacing animal experimentation with meaningful in vitro or in silico methods according to the 3Rs (and 4R concept) principle or at least reducing them to an absolute minimum. This leads to the improvement of the state-of-the-art in vitro methods to reduce the animal numbers used for research and minimise the pain experienced during experiments. These comprise but are not limited to the use of classical cell culture models as well as more advanced methods such as three-dimensional model systems such as tumour spheroids, organoids, organ-on-a-chip technologies, or computer-based models such as prediction methods based on artificial intelligence (AI), as previously applied to diabetes
[10], cardiovascular disease
[11], tuberculosis
[12], and drug discovery
[13]. Spheroids pose a model of compact three-dimensional cell aggregates consisting of cells at different states, e.g., proliferating, hypoxic, and quiescent, which are generated on non-adherent surfaces. These do not necessarily represent complex organ architecture on a miniature scale
[14]. On the other hand, organoids are three-dimensional models of organ systems reflecting organ microanatomy. Due to their stem-cell-originating nature, organoids are usually indefinitely expandable
[15][16]. Modelling different organ systems of various animals will help to replace animal experimentation in accordance with the 3Rs (and 4R concept) principle. This leads to an improved understanding of the biological principles in a broader context, as humans and different species of animals may react differently to various irritants (
Figure 1).
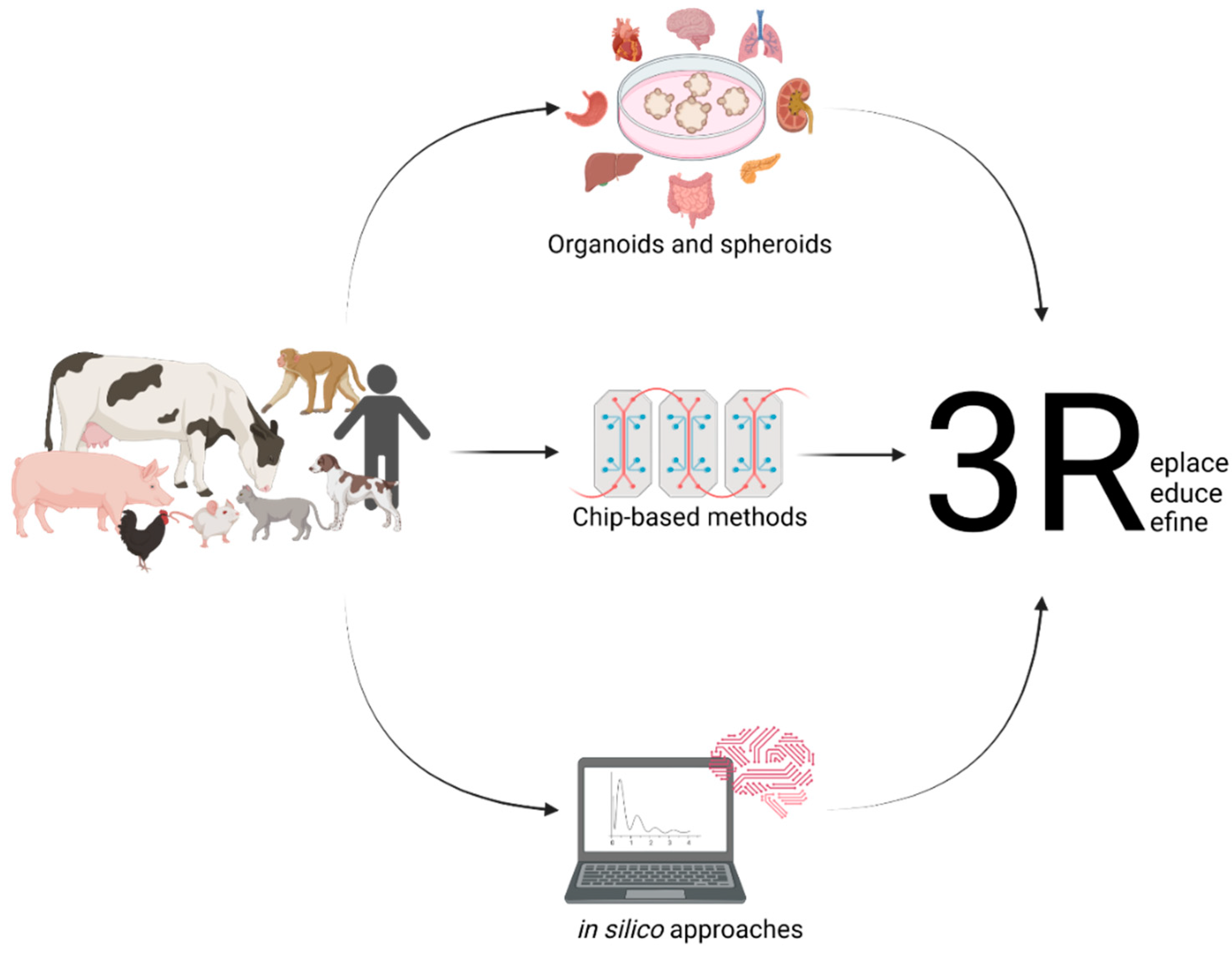
Figure 1. Setting up useful in vitro models from different animals and their various organs and the use of in silico modelling will help to replace the need for animal experimentation.
2. The Importance of Organoids for One Health
The establishment of meaningful in vitro systems to model complex diseases is very important. At the moment, the world is progressing from using classical cell culture models to more sophisticated three-dimensional models to investigate the effects of commensal or pathogenic organisms on certain cells/organs of humans, companion animals as well as farm animals. In humans, many different organs are available as organoid systems, e.g., the brain
[17], retina
[18], salivary gland
[19], thyroid
[20], lung
[21], blood vessels
[22] and the heart
[23], mammary gland
[24], stomach
[25], liver
[26], kidney
[27], pancreas
[28], intestine
[29][30], fallopian tube
[31], endometrium
[32], bladder
[33] and the prostate
[34]. Many of these can be adapted to cancer organoid cultures, and some have been translated to animal organoid models. There are also very sophisticated air–liquid interface models of patient-derived cancer organoids. One of these models even includes the complex tumour microenvironment with immune cells, making it a very attractive and complex model
[35]. A lot of work has been undertaken on organoids from companion animals, including the canine and feline intestine
[36][37][38][39], the canine and feline liver
[40][41], and canine kidney
[42], bladder cancer
[43], prostate cancer
[44], skin
[45], and thyroid tissue
[46]. These companion animal models are further complemented by organoids derived from farm animals. Among them are primarily intestinal organoids from several species such as pigs, cattle, sheep, horses, and chickens
[47], which have recently been reviewed more in-depth elsewhere
[48]. In this context, organoids may develop towards a central model connecting the three cornerstones of the One Health concept regarding the physiological and pathophysiological interrelation of human, animal, and environmental health.
Gastrointestinal (GI) diseases do not only affect humans but also constitute a major threat to farm and companion animals and are associated with high costs to healthcare systems and animal owners. Just as in humans, conceivably lethal GI diseases also affect animals. Enteropathogenic viruses and bacteria are frequently responsible for the initiation or further impairment of GI afflictions
[49][50][51]. There are numerous examples of the pathogenic organisms involved in the development of health problems in humans as well as animals. Several reviews have recently highlighted the importance of One Health approaches putting surveillance, monitoring, and treatment options in a broader context compared to studies investigating only one aspect of potentially zoonotic pathogens. Due to the fact that some pathogens can survive in the environment or animal products consumed by humans, the transmission routes should be examined more closely.
Especially, enteric pathogens are a major threat in a zoonotic One Health context, including parasites such as helminths
[52],
Giardia duodenalis,
Blastocystis, and
Cryptosporidium spp.
[53], as well as bacteria such as
Clostridioides difficile (
C. difficile)
[54][55][56],
Bacillus cereus sensu lato
[57], and
Salmonella [58], which all affect humans as well as animals. Particularly, the widespread
C. difficile has been well studied, with the faeces of animals contaminating soil and water with
C. difficile spores, leading to the spread of the disease to other animals. Alike, the spores from infected humans show up in wastewater, highlighting the importance of
C. difficile for the environment as well as human and veterinary medicine
[59]. This is complemented by reports that animals may be important asymptomatic carriers of toxigenic
C. difficile [60][61]. Additionally, the co-clustering of isolates from cattle and dogs with isolates from human newborns has been documented, indicating the opportunity for inter-species transmission, either directly or indirectly, via contaminated environments
[62]. How food intake shapes gut health has also been reviewed many times. Especially, fermented foods have received a lot of attention because of their ability to substantially change gut microbiota composition and therefore influence physiologic as well as pathologic processes
[63].
In recent years, intestinal organoids have become increasingly important in research. They do not only represent a more complex system than classical two-dimensional cell cultures, but their three-dimensional nature also allows for the long-term maintenance and differentiation of many different cell types within one dish. Despite their complexity, intestinal organoids bear the advantage of only consisting of one layer of epithelial cells, thus putting the intestinal epithelial lining at the heart of the research. Intestinal organoids are not only valuable models for the investigation of complex diseases, such as IBD
[64][65], but also represent a system which makes it possible to propagate pathogens in vitro, which previously could not be cultured, such as
Cryptosporidium [66]. Beyond that, organoids even open up opportunities for precision medicine, as any effects can be studied in a patient-specific manner. Organoids can be the missing piece in the puzzle of performing research in a One Health context (
Figure 2).
Figure 2. Organoids in One Health Research: Organoids are a possible way to work with all parts of One Health in one platform. Using organoids, one can learn about animal and human health and disease as well as interactions with the environment and bacteria, fungi, parasites, and even viruses.
This entry is adapted from the peer-reviewed paper 10.3390/ani12182461