Breast cancer (BC) is the second leading cause of death among women, and it has become a global health issue due to the increasing number of cases. Different treatment options, including radiotherapy, surgery, chemotherapy and anti-estrogen therapy, aromatase inhibitors, anti-angiogenesis drugs, and anthracyclines, are available for BC treatment. However, due to its high occurrence and disease progression, effective therapeutic options for metastatic BC are still lacking. Considering this scenario, there is an urgent need for an effective therapeutic strategy to meet the current challenges of BC. Natural products have been screened as anticancer agents as they are cost-effective, possess low toxicity and fewer side effects, and are considered alternative therapeutic options for BC therapy. Natural products showed anticancer activities against BC through the inhibition of angiogenesis, cell migrations, proliferations, and tumor growth; cell cycle arrest by inducing apoptosis and cell death, the downstream regulation of signaling pathways (such as Notch, NF-κB, PI3K/Akt/mTOR, MAPK/ERK, and NFAT-MDM2), and the regulation of EMT processes. Natural products also acted synergistically to overcome the drug resistance issue, thus improving their efficacy as an emerging therapeutic option for BC therapy.
1. Pathogenesis of Breast Cancer
Breast cancer (BC) is the most common cause of malignant tumors worldwide. BC can occur from any mutational defect in breast ducts
[1]. Generally, BC is categorized on the basis of estrogen receptors into ER-positive and ER-negative breast cancers
[2]. Based on specific biomarkers, BC is further categorized into subtypes, such as luminal A, B, and basal-like. TNBC is the basal-like and most severe form (see
Figure 1)
[3].
Figure 1. The initiation and progression of BC by obese breast adipose-derived factors. Factors released by obese breast adipose tissue may operate as mutagens, for example by activating intracellular reactive oxygen species (ROS), which can lead to DNA damage in normal breast epithelial cells and other inflammatory mediators. DNA damage may occur as a result of estrogen’s mitogenic actions, which can lead to replication stress and stress. Unresolved DNA damage, which is associated with mutagenesis and the onset of cancer, may result from increased DNA damage and possible estrogen-induced defective DNA repair. An obese breast adipose tissue microenvironment promotes BC proliferation, migration, and invasion by releasing inflammatory mediators, increasing leptin, decreasing adiponectin, and increasing estrogen levels. This figure is reproduced from Bhardwaj et al.
[4] (Creative Commons Attribution License (CC BY 4.0)).
Many environmental and genetic factors increase the risk of BC. These factors include increased damage to DNA, unusual hereditary mutations, exposure to estrogen, and a lifestyle that can increase the development of BC. Patients with a family history already in the malignant phase can also increase the chances of developing BC
[5]. Most of the patients inherit their susceptible genes viz
p53,
BRCA1, and
BRCA2 [6]. Unusual mutation in CDH1 and overexpression of p53 can also increase the risk of developing BC
[7]. RAS/MEK/ERK, PI3K/AKT, and RAS/MEK/ERK are the main pathways that help the normal cells to defend against cell death. Sometimes, mutational gene events in these pathways increase the chances of BC, as normal cells are incapable of committing cell suicide. For example, mutations in the PTEN gene activate the PI3K/AKT pathway, and cancerous cells are unable to go in commit suicide (see
Figure 2)
[8].
Figure 2. The representation of aberrant signaling pathways involved in BC. The green arrow represents the upregulation/activation, while the red arrow represents downregulation/inhibition. This figure is reproduced from Varghese et al.
[9] (Creative Commons Attribution License (CC BY 4.0)).
During BC development, epithelial cells change from neoplastic cells into cancerous cells. Adipose tissues mainly contribute to the initiation and progression of BC
[4]. The main factors include the inflammatory mediators and mutagens in the form of estrogens that stimulate the production of ROS that ultimately causes severe damage to DNA in epithelial cells of the breast. Increased damage to DNA induced by estrogen causes severe defects and might lead to dysfunctional DNA repair. These unusual changes in DNA increased the risk of mutagenesis of BC. BC progression is accelerated by excess estrogens leptin and inflammatory mediators which promote BC cell proliferation, migration, and invasion
[10].
In BC, chronic inflammation is mediated by tumor-infiltrating lymphocytes, cancer-associated fibroblasts, tumor cells, and tumor-associated macrophages. Inflammatory actions are triggered by either necrotic cells, such as damage-associated molecular patterns (DAMPS), or products released by microorganisms, such as pathogen-associated molecular patterns (PAMPS), in the case of breast cancer. As a result, innate and adaptive immune cells secreted the chemokines and cytokines that mediate inflammatory responses
[11]. Angiogenesis is initiated by the activation of angiogenesis regulators IL-6, TNF-α, NF-κB, VEGF, Jun/Fos, and LPO. Different pathways are linked with chronic inflammation in BC, such as Notch, NF-κB, PI3K/Akt/mTOR, MAPK/ERK, and NFAT-MDM2 signaling pathways, as well as the regulation of EMT processes
[12]. NF-κB is the most critical mediator during chronic inflammation in breast cancer. In NF-κB pathway, activation of different genes such as
IER3L,
COX2,
CXCL12, and
CCND3 is a critical inflammatory mediator in patients with BC
[13]. Various signaling pathways are activated during the inflammation of BC, which then leads to the activation of transcription factors, such as the signal transducer and activator of transcription signaling (STAT) and the activator protein 1 (AP-1) transcription factor
[14].
Chronic inflammation in BC represented the seventh hallmark of cancer as compared to other malignancies. Various cellular events are major consequences of tumor progression, proliferation, and survival. Chronic inflammation indicates different events of BC, such as initiation and progression stages. Identifying different events would be helpful as an important strategy for controlling the BC among high-risk populations and prevention
[15][16].
2. Recent Discoveries and Developments of Natural Products against BC
2.1. Novel Verminoside and Their Derivatives
Various adjuvant therapies have been used, including combinations of natural products, in order to improve their susceptibility to BC. These novel products have replaced or limited the use of conventional therapies due to increasing drug resistance. Different natural products have been reported influenced positive effects and could be used as adjuvant therapy by targeting tumors or affecting the EMT process
[17]. For example, verminoside (VMS) from
Pseudolysimachion rotundum interferes with the EMT process by suppressing the invasion and tumor growth; thus, it is widely used for BC treatment
[18].
VMS has been used as an anticancer agent and exhibited inflammatory properties. Its anticancer activities have been evaluated in in vivo and in vitro models. Lee et al.
[18] carried out an in vivo study in animal models (PyMT/FP635 mouse model) to investigate the anticancer potentials of VMS and showed maximum activity against MDA-MB-231 and MCF7 cells with an IC
50 value of 10 µM. They found that VMS suppresses epithelial lining growth and the transition of mesenchymal breast cells without activating the ERK signaling pathway (see
Table 1). Therefore, VMS can be used as a chemo-adjuvant for the treatment of BC.
Table 1. The in vitro and in vivo studies on the role of natural products in downregulating the signaling pathways against various types of BC models.
Extracted Compound |
Biochemical Structure |
Biochemical Nature |
Source |
Study Type |
BC Type |
Animal Model |
Key Finding |
Mechanism of Action |
Reference |
VOA |
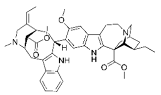 |
Alkaloid |
V. africana |
In vitro |
ER-positive, TNBC, and HER2-positive BC |
--- |
Downregulating the PI3K/Akt/mTOR. VOA showed its usefulness against MCF-7 and 4T1 cells with IC50 values (0.99, 1.48 μM). |
VOA significantly inhibits the phosphorylated AKT and mTOR in BC cells and also decreases the expression of CDK2, cyclin A, E. It also induces apoptosis and cell death in MCF-7 and 4T1 cells by arresting the S phase of the cell cycle. |
[19] |
Lin A |
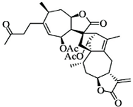 |
Sesquiterpenoid |
I. lineariifolia |
In vitro |
TNBC, and HER2-positive BC |
--- |
Lin A induced apoptosis at a higher concentration of 50% in BC cells (MCF7 and MDA-MB-231 with IC50 (4.5 ± 0.3, 7.8 ± 0.6). |
Lin A arrests the cell cycle at the G2/M phase, and inhibits cell invasion and cell proliferation in BC cells. |
[20][21] |
Fisetin |
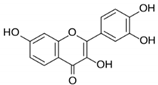 |
Flavonol |
Cucumber, apple, strawberry |
In vitro and in vivo |
ER-positive, TNBC, and HER2-positive BC |
BALB/c mice |
Fisetin induced apoptosis in MCF-7, 4T1, and MDA-MB-231 at 40 and 80 μM. |
Fisetin acts as an inhibitor of PI3K/Akt/mTOR signaling and inhibits the proliferation and dysregulation of this signaling pathway. |
[22] |
WG |
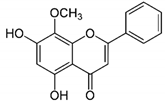 |
Flavone |
S. baicalensis |
In vitro and in vivo |
ER-positive, TNBC, and HER2-positive BC |
Chicken chorioallantoic membrane (CAM) |
WG showed inhibitory effects on MCF-7 and MDA-MB-231 at 20 and 40 μM. |
WG acts as an inhibitor of PI3K/Akt/mTOR signaling and shows inhibition in cell proliferation. |
[23] |
AP |
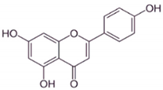 |
Flavone |
A. cepa, C. sinensis |
In vitro |
ER-positive, HER2-positive BC |
--- |
It influenced the NF-κB pathway by suppressing the VEGF through deactivating progesterone receptors in BC cells. |
It inhibits cell proliferation and migrations by arresting the cell cycle at the G2/M phase. It also suppresses the cyclin A, B, and CDK1 which controls the G2/M phase. |
[24] |
Oridonin |
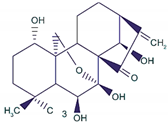 |
Diterpenoid |
R. rubescens |
In vivo |
--- |
BALB/C athymic nude mice |
It induced apoptosis and cell death in BC cells. |
Notch 1-4 protein expression is lowered by oridonin therapy, which hinders cancer cell migration and invasion. |
[25] |
Genistein |
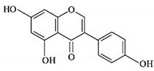 |
Isoflavones |
Soy-based foods |
In vitro |
ER-positive, TNBC |
--- |
Activation of NF-κB showed potential against MCF-7 at an IC50 value of 20 µM. |
It inhibits the phosphorylation of IκBα in MCF-7/T47D/MDA-MB-231 cell lines, thus playing a significant role in the regulation of IκBα to the p50. |
[26] |
GLA |
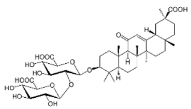 |
Terpenoid |
G. glabra |
In vitro |
ER-positive, TNBC, and HER2-positive BC |
--- |
It showed anticancer activity against MDA-MB-231/BT549. |
It inhibits invasion and cell proliferation, as well as promote the expression of E-cadherin. |
[27] |
ATG |
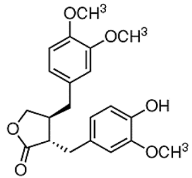 |
Isoflavones |
S. heteromalla |
In vitro and in vivo |
ER-positive, TNBC |
BALB/cA-nu |
It showed anticancer potentials in MDA-MB-231 cells at 200 μM. |
Inhibiting the phosphorylation of MAPK/ERK in MDA-MB-231 cells. |
[28] |
PPD |
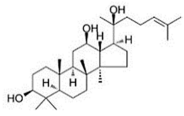 |
Glycoside |
P. notoginseng |
In vitro and in vivo |
TNBC, and HER2-positive BC |
BALB/C nude mice |
It showed maximum activity below 20 μM against MDA-MB-231. |
PPD targets BC cell lines by suppressing the MAPK pathway through the deactivation of ERK1/2, p38, and JNK. |
[29] |
Kaempferol |
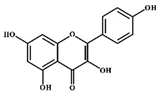 |
Flavonols |
Onions, lettuce |
In vitro |
ER-positive, TNBC |
--- |
The number of cancerous cells decreased from 85.2% to 50.32% in the G1 phase of the cell cycle. Kaempferol significantly inhibited the BC cells (BT474 and MDA-MB-231) by blocking the critical phases of cell cycles. |
Inhibitory actions against different breast cell lines can inhibit the expression of genes involved in MAPK/ERK. This shows that binding with estradiol causes degradation of Erα. |
[30] |
Cimigenoside |
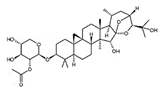 |
Glycoside |
C. dahurica |
In vitro and in vivo |
ER-positive, TNBC |
BALB/C nude Crlj mice |
Cimigenoside showed maximum anticancer activity against BC cell lines (MDA-MB-231, MCF-7) with IC50 (12.6 ± 1.47, 15.6 ± 2.47 μM). |
Cimigenoside induces apoptosis in BC cells by arresting the G2/M phase of the cell cycle. An in vitro study of cimigenoside also inhibits/attenuates BC cell proliferation and invasion. An in vivo study inhibited the growth of tumor growth in mice models. |
[31][32] |
Ginsenosides |
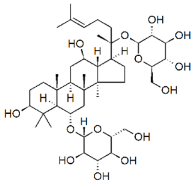 |
Glycosides |
P. notoginseng |
In vitro and in vivo |
ER-positive, TNBC |
Nu/nu mice |
It showed maximum anticancer activity against BC cell lines (MDA-MB-231). |
25-OCH3-PPD is involved in arresting the G1 phase of the cell cycle and induces apoptosis in BC cells by downregulating MDM2. |
[33] |
BA |
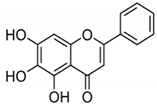 |
Flavonoid |
Scutellaria baicalensis |
In vitro and in vivo |
ER-positive, HER2-positive BC |
BALB/c mice |
It showed suppression of the NF-κB pathway in the development of human breast epithelial cells (MCF10A). |
Suppress the NF-κB signaling pathway, as well as IL-1β, Bcl-2, and VEGF. |
[34][35] |
VMS |
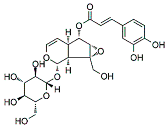 |
Monoterpenoids |
P. rotundum |
In vitro and in vivo |
ER-positive, TNBC, and HER2-positive BC |
PyMT/FP635 mouse |
It showed maximum activity against MDA-MB-231 and MCF7 cells with IC50 value (10 µM). |
VMS suppresses the growth of epithelial lining and the transition of mesenchymal breast cells. |
[18] |
Calcitrinone A |
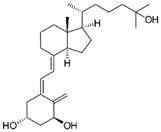 |
Phloroglucinol |
C. citrinus |
In vivo and in vitro |
ER-positive |
Chick chorioallantoic membrane (CAM) |
Calcitrinone A induced apoptosis and cell death in MDA-MB-231 cells. |
Calcitrinone A interferes with mitochondrial function by blocking succinate coenzyme Q reductase and ultimately inhibits the complex II that increases the production of ROS. |
[36] |
Vulpinic acid |
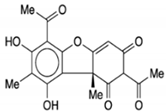 |
Butenolide |
Lichens |
In vitro |
ER-positive, TNBC, and HER2-positive BC |
--- |
Vulpinic acid induced apoptosis in MCF-7. |
Elevate the levels of FOXO-3 and Bax, and suppress the expression of Bcl-2 and procaspase-3/9 to enhance the activity of tumor suppressor miRNAs. |
[37] |
Genistein |
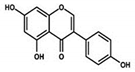 |
Isoflavone |
Fabaceae family |
In vitro |
TNBC, and HER2-positive BC |
--- |
It induced apoptosis and cell death in MCF-7 and MDA-MB-231 cells. It also inhibited cell proliferation and progression in BC. |
Arresting the cell cycle at G2/M phase, downregulating CDK-1, and inhibiting the expression of Bcl-2 and the function of DNA polymerase II. |
[38][39] |
CUR + BBR |
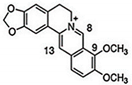 |
Diarylheptanoid, isoquinoline alkaloid |
Curcuma longa, berberine from Rhizoma coptidis |
In vitro |
ER-positive, TNBC, and HER2-positive BC |
--- |
It showed effects against BC cell lines (MDA-MB-231 and MDA-MB-468) at p ≤ 0.010. |
The EMT process in the case of BC is impaired. |
[40][41] |
BA + 5-FU |
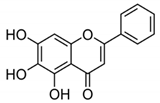 |
Flavonoid (BA) |
Scutellaria baicalensis |
In vivo |
--- |
Swiss albino mice |
It showed inflammation by inhibiting the VEGF, IL-1β, and NF-κB. |
Inflammation is inhibited by the VEGF, IL-1β, and NF-κB, which play significant roles in preventing BC. |
[42] |
MG + 5-FU |
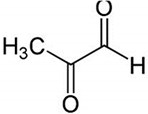 |
Polyphenolic (MG) |
Coffee, wine |
In vivo and in vitro |
ER-positive, TNBC, and HER2-positive BC |
BALB/c mice and Swiss albino mice |
It showed anticancer activity against the BC cell line (MCF-7). |
Arresting the cell cycle at MG G0/G1 phase induces apoptosis and cell death by increasing Bac-2 and caspase 9. |
[43] |
RSVL + SAL |
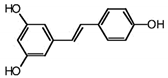 |
Polyphenol |
Red grapes |
In vivo and in vitro |
ER-positive, TNBC, and HER2-positive BC |
Swiss albino mice |
It showed anticancer activity against MDA-MB-231. |
Arresting the S1 phase of the cell cycle also induces apoptosis in BC cells. Other activities include inhibition and cell proliferation. It acts as an antioxidant by preventing the DNA dame and suppressing the tumor growth. SAL inhibits the epithelial mesenchymal transition, and suppresses p53, COX-2, and Beclin. |
[44][45] |
VMS is a monoterpenoid that also has been isolated from
K. pinanta. The biochemical nature of VMS is greatly reflected due to the presence of two hydroxyl groups that are responsible for anticancer and inflammatory properties. It is widely used for inhibiting the EMT process in BC
[46]. BC cells under the EMT process have increased mesenchymal characteristics and decreased the formation of epithelial cells and cell linings
[47]. These events promoted invasion and migration among BC cells. In the previous studies, it was shown that the excessive use of chemotherapeutic drugs promoted the EMT process. As a result, chemoresistance is one of the major issues tackled through the safe use of natural products as they can inhibit invasion, migration, and EMT in BC development
[18].
2.2. Novel Phloroglucinol and Derivatives
Phloroglucinols are phenolic compounds that exhibit anticancer and anti-inflammatory activity. Phloroglucinol is the most important novel natural product, and its chemical structure reveals that it has an aromatic ring surrounded by hydroxyl groups. It is composed of two phloroglucinol units linked by a methylene bridge. Calcitrinone A is a type of natural product, novel phloroglucinol, isolated from
C. citrinus. Calcitrinone A showed potential against MDA-MB-231 BC cells
[48].
Calcitrinone A inhibited cell proliferation, invasion, and tumor growth, and stimulated apoptosis in MDA-MB-231 cells. Calcitrinone A is less toxic as compared to other drugs used for the treatment of BC. Calcitrinone A bound with the succinate-coenzyme Q reductase located at complex II of the mitochondrial membrane and blocks their activity. It resulted in the depletion of ATP levels. Calcitrinone A is the most promising anticancer agent used for BC therapy as it interferes with complex II of the mitochondria
[49].
An in vivo and in vitro study was conducted by Gaafary et al.
[36] to investigate the role of calcitrinone A in the chick chorioallantoic membrane (CAM) and MDA-MB-231 cells, respectively. They found that calcitrinone A interferes with mitochondrial function by blocking succinate coenzyme Q reductase and inhibiting complex II, which can untimely increase ROS production. These events induced apoptosis and cell death in MDA-MB-231 cells (see
Table 1). Kim et al.
[50] found that phloroglucinol inhibited the epithelial–mesenchymal transition in BC; they also found that phloroglucinol increases ROS production. They found that phloroglucinol also induced apoptosis and cell death in metastatic BC cells.
2.3. Role of Viridiflorol against BC
Viridiflorol is a natural organic compound, and its chemical structure contains a cyclopropazulen in its carbon skeleton, responsible for anticancer activity against BC. Viridiflorol, which was isolated from
S. algeriensis. Furthermore, the Lamiaceae family, including the
Senecio rowleyanus Jacob,
Mentha aquatica L, and
Ballota undulata, are excellent sources of viridiflorol derivatives
[51][52]. Previous studies showed that oil secretions from
S. rowleyanus contained viridiflorol (11%) that possesses anticancer and anti-inflammatory activities
[53]. The oil secretions from
Salvia leriifolia showed anticancer activity against BC (MCF-7 and MBA-MD-231)
[54]. Viridiflorol is a class of sesquiterpenoid that isolated various aromatic plants, such as
M. quinquenervia and
A. edulis. Extracts of viridiflorol from these plants exhibited anticancer activities against different BC cell lines (MCF-7 and MDA-MB-231)
[55]. Essential oil from
Blepharocalyx salicifolius and
Cyperus longus is an excellent source of viridiflorol
[56][57].
Akiel et al.
[58] performed an in vitro study in searching for anticancer activities of viridiflorol against BC MCF-7 cells. The tested viridiflorol showed maximum anticancer activity against MCF-7 with IC
50 value of 0.1 µM. Memariani et al.
[56] conducted in vitro study to investigate the anticancer potentials and apoptosis of viridiflorol. The tested derivatives of viridiflorol showed maximum anticancer activity against MCF7 with IC
50 value of 31.60% and induced apoptosis at concentrations of 78.23%. Furtado et al.
[57] also conducted in vitro study and successfully isolated viridiflorol from
Blepharocalyx salicifolius that showed anticancer potentials against MDA-MB-231 at concentrations of 46.60 µg/mL. The isolated viridiflorol is also involved in suppressing the cellular metabolism and biological activities of BC cells.
2.4. Effect of Vulpinic Acid against BC
Vulpinic acid is a natural product that was isolated from lichens and exhibited anticancer potentials against BC. Lichens secreted a large variety of secondary metabolites effective for the treatment of BC. For example, vulpinic acid is secreted by lichenized fungi which demonstrates anticancer and anti-proliferative activities
[59]. Vulpinic acid is a safe and easily assessable metabolite in nature. Vulpinic acid inhibits cell proliferation and invasion, inhibits tumor growth, and stimulates apoptosis in MC-231 cells. Vulpinic acid interacts with the FOXO-3 gene and suppresses their activity, leading to decreased expression of miRNAs
[60].
In the previous studies, vulpinic acid has been used for breast treatment as it induces apoptosis and suppresses Bcl-2
[61]. Cansaran-Duman et al.
[37] conducted an in vitro study and investigated the role of vulpinic acid on BC by regulating the expression of miRNA levels. They found that vulpinic acid induced apoptosis in MCF-7 by elevating the level of FOXO-3 and Bax, and suppressing Bcl-2 and pro-caspase-3,9, thus altering the tumor suppressor miRNAs (see
Table 1). Kilic et al.
[62] investigated the anticancer potentials of vulpinic acid against MCF-7, BT-474, MDA-MB-231, and SK-BR-3. They found that vulpinic acid showed anti-proliferative and significant inhibitory effects on the MCF-7. They also found that vulpinic acid induced apoptosis and cell death in MCF-7.
2.5. Action of Genistein against BC
Genistein is a naturally occurring compound and possesses anticancer, anti-proliferative, and anti-tumor activities. Fabaceae families are rich in genistein and its derivatives. It is also found in soybeans. It is used to overcome the drug resistance caused by BC and control the reoccurrence of metastatic invasion. It also suppresses the activities of DNA polymerase II
[63]. It also arrested the cell cycle at the G2/M phase and induced apoptosis and cell death in MCF-7 and MDA-MB-231 cells. It also inhibited the cell proliferation and progression of BC. Genistein has been used for clinical uses for the prevention of BC because it increases survival rates
[38].
Other studies investigated the role of genistein against BC and found that it downregulated CDK-1 and inhibited the expression of Bcl-2, as well as the function of DNA polymerase II. They also found that genistein also increased the expression of p21 and p51
[39][64]. Genistein also showed inhibitory effects on tyrosine kinases and inhibited the cancer progression. Xie et al.
[65] investigated that genistein is an effective therapeutic agent that inhibits DNA methylation in MCF-7 and MDA-MB-231 cells by blocking DNA methyltransferase activity. Liu et al.
[38] studied that genistein showed inhibitory potentials in the deactivation of IGF-1R and p-Akt. They also found that it decreases the level of Bcl-2 by promoting apoptosis.
This entry is adapted from the peer-reviewed paper 10.3390/molecules27113412