1. Introduction
Sustainable architectures are integrating the inhabitants into natural environments and micro-environment controls and adopting efficient material resources and energy in buildings. Sustainable designs aim to foster the coexistence and welfare of human beings, inorganic elements, and living things that make up the ecosystem and find architectural responses
[1][2][3]. An integral portion of infection inhibition and management mechanisms should be the hospital’s architectural design process. Apart from low carbon emissions and low energy, Van-Khai
[4] supported the idea that the sustainable architecture purpose for health facility designs should incorporate design methods for the prevention and control of communicable infections. The World Health Organization (WHO) emphasizes that combating infectious diseases and protecting population centers from their spread is a scientific strategy, based on well-studied solutions aimed at preventing the health risks and harms caused by the infection of people and health workers
[5]. Given the quick and ongoing changes in the healthcare environment in the context of advances in medicine, innovation, and strategic culture, this requires a strategy to operate the facilities throughout their lifespan
[6]. It is also reported that the architectural designs of the hospitals took a significant share of the pandemic in the Tugela Ferry outbreak in South Africa in 2006, which showed the importance of facility design to expand hospitalization
[7]. Hospitals must be built and designed using strong human-factor concepts; they must also be able to adapt to emerging problems and urgent demands, as well as accommodate future requirements, particularly when dealing with emergency situations such as COVID-19
[8][9][10][11]. The implications are that the well-being and health of humans are strongly linked with constructed environments. Based on the current reports from the disease control center regarding the Coronavirus transmission mode, it has become essential to balance the need for control measures and the open-access design concept to reduce the infection rates
[12]. Modular and quick building, repurposing of places, and temporary setting equipment have all evolved as ways to address the pressing demand for adaptable and robust solutions
[13][14]. Recent and past research has indicated that unstable climates and climate change play essential roles in driving global emergence, resurgence, and redistributions of communicable infections
[15][16]. In addition to that, the climate’s continuous change has a significant impact on the constructed environments
[17][18]. Today’s healthcare infrastructures are developed and built with the idea in mind that they should be important for a long lifetime, which does not allow for changes in service need on a regular basis
[19].
The impact of buildings’ indoor environmental quality (IAQ) on residents’ health is an important topic in civil, architectural, mechanical engineering, and public health. The concept that air pollution can raise human exposure to viral infections such as SARS-CoV-2 is currently supported by recent data
[20]. In previous studies, IAQ indicators were included in the suitability of ambient temperature, visual visibility, acoustic properties, humidity, and ventilation on the health of the inhabitants. It is believed that the impact of the IAQ on the health of the population is closely related to the factors and characteristics of the built environment
[21][22]. The accumulation of indoor air fluid in buildings has exacerbated the phenomenon of sick building syndrome. Therefore, many believe that people living in areas with a green and healthy environment would have better health
[23][24]. The linked relationship between environmental health, the projected results, and the consequences are depicted in
Figure 1. As indicated in
Figure 1 [22], several methodologies collaborate to produce a shared outcome regarding the health of the occupants
[22].
Figure 1. The tangled links between environmental health and projected human health consequences.
2. Controls, Mitigations, and Health Care Setting Special Consideration
Filtration and ventilation are vital in minimizing SARS-CoV-2 transmission potential, although viruses can be found in aerosols of sizes that can penetrate high-efficiency filters
[25] (see
Figure 2a,b)
[26]. Proper installations and maintenance of filters can minimize the airborne transmission risks. Higher air-exchange rates and outside air fractions in houses can assist in diluting the indoor pollutants from the air that is inhaled in the built environments
[27][28]. This can be achieved by further ventilating the external air damper position on air-handling units, therefore draining greater ratios of any airborne viral particles present and indoor air. It is stated that poor interior air quality is characteristically caused by sources that release particles, viruses, gases, and diseases into the air. Insufficient ventilation is widely regarded as the single most prevalent cause of pollution buildup (
Figure 3a)
[26], as it can enhance indoor levels of pollution by failing to bring in enough outdoor air to dilute emissions from inside sources and failing to remove indoor air pollutants to the outside. High temperatures and humidity levels can also raise pollution concentrations. Inadequate filtering is the second most common source of pollution accumulation (
Figure 3b)
[26]. Notwithstanding fundamental advances in air filter technologies, a great many buildings continue to rely on ineffective filters or are neglectful in the maintenance of acceptable filters.
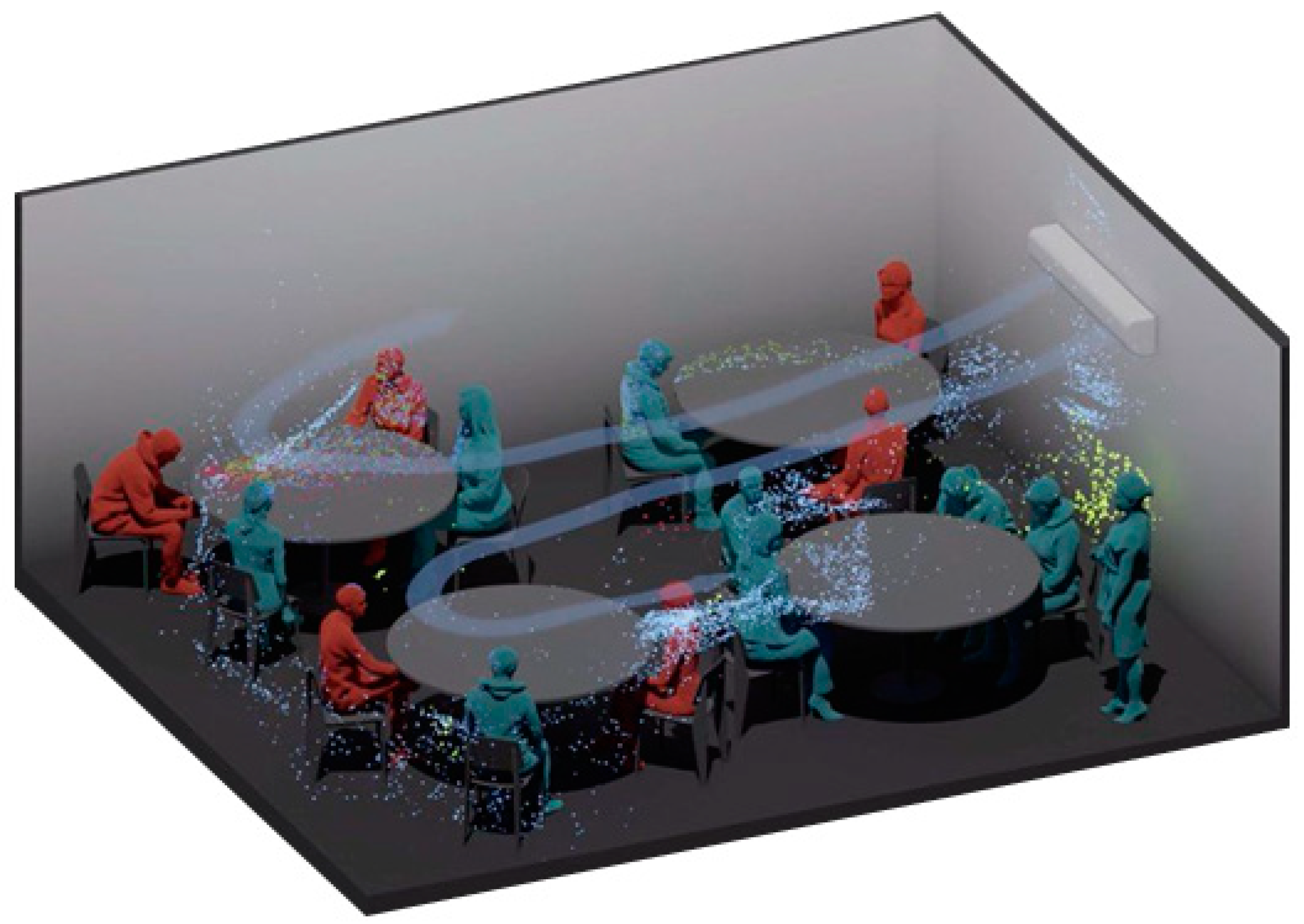
Figure 2. Computer simulation at Oregon University displaying the possible spread of COVID-19 in a restaurant with an air recirculation system (Adapted from [
74]).
Figure 3. Rate of architectural design efficiency of the indoor environmental quality: (
a) insufficient ventilation and (
b) ineffective filtration (data adapted from
[26]).
Relative to these modified building operation parameters, there are some cautions to consider
[29]. First, improving external air factions can come with a raised consumption of energy. This is a valuable mitigation method for supporting human health in the short term. After the risk period has passed, construction workers are advised to return to ordinary ratios. Secondly, not all air-handling models have the capacity to significantly increase external air ratios. Those air-handling systems that have the capacities may need more regular filter-maintenance protocols. Thirdly, increasing airflow rates that just improve the recirculated indoor air delivery might possibly raise the transmission possibility. Moreover, the spatial configuration of buildings can discourage or encourage social interaction
[30]. Western societies in recent years have appreciated designs that focus on feelings of “spaciousness” indoors, whether in workplaces or homes, via the application of open-area ideas that connect open-office ideas that deliberately direct inhabitants to chance encounter nodes and are thought to improve innovations and collaborations among workers. Spatial configurations can unintentionally improve transmission opportunities of viruses via designed human interactions.
Hospitals have constrained alternatives for social-distancing controls to prevent virus spread
[31]. Moreover, health care and hospital facilities are usually buildings of patients with massively different necessities from the built environments adjacent to them. For instance, people with underlying conditions are usually put within protective environment (PE) spaces, positively pressured to reduce external airborne communicable microbes from accessing the room
[32]. Nevertheless, these pressurization differentials also increase the probability that infectious agents in the patient rooms will migrate into the spaces of the higher traffic corridors when the doors are open, involuntarily exposing visitors, health care workers, and other patients.
Airborne infection isolations (AII) rooms, in comparison, use negative pressure differential comparative to neighboring rooms and corridor spaces, directly draining building air to the room exteriors
[33]. The same negative pressure may compulsorily expose the building dwellers to airborne microbes from the corridor-space occupants. Airborne infection isolation rooms and protective environments can be designed with anterooms that are utilized as extra buffers between protected spaces and common areas to offer locations for health care practitioners to use and eliminate PPE and prevent virus spread. Nevertheless, an anteroom is not needed for airborne infection isolation rooms and protective environments and exists in certain buildings. Anterooms use extra floor areas, thus increasing the visual barriers among medical care teams and patients and increasing costs. In future operational protocols and designs, these trade-offs might be proposed given the critical roles of health care settings and the high costs of the epidemic during this time.
It can be concluded that ventilation is necessary to purify the air by developing the means to eliminate infection through filters or rays. In addition, ventilation and air purification are among the most important factors that limit the spread of infection. It requires reducing air pollution and the surrounding environment, in addition to increasing environmental awareness and paying attention to public health.
3. Architectural and Design Engineering for the Controls in Post-Pandemic
Health-associated infections highlight the need for more excellent knowledge of how the built environment influences infection transmission. Furthermore, people must recognize what design, structural, and operational improvements in the built environment can be made for better infection prevention and control.
Figure 4 shows a multidisciplinary framework for mitigating the urban and social impacts of the measures of COVID-19
[34].
Figure 4. A multidisciplinary framework for mitigating the urban and social impacts of the measures of COVID-19 (Adapted from
[34]).
3.1. Future Human-Centered Design
To prevent sick building syndrome, chronic diseases, and allergies, architectural engineering should seek natural ventilation and avoid relying on artificial environmental conditions and mechanical ventilation. In the future built environment, more human-centered designs are required in architecture and urban design.
Thus, preparing a long-term design plan to avoid disease outbreaks in the future is very important to protect the population from infectious diseases
[35]. Focusing on the human-centered approach can save energy in this case to achieve sustainability and a healthy environment. In addition, future designs for low-income communities must include cost-effective and health-secure alternatives
[36]. The distribution of air pollutants around buildings can be redesigned using a building morphology and a passive urban approach contingent on an analysis of detailed local climate and site factors. In addition, inside the building, passive design principles allow natural ventilation and air distribution
[37]. However, it is advised that areas with high levels of pollution adopt adequate disinfection and purifying processes
[38].
Buildings need a comprehensive IAQ management plan that incorporates an optimal temperature and controls humidity regulation, ventilation, and air filtration for future human-centered designs. These are regarded as critical techniques for improving IAQ and protecting citizens from diseases spread by the air
[37]. This strategy should also include the selection of building materials that are safe for human health, especially the respiratory system, in the long term, and the effective regular operation and maintenance of ventilation and air conditioning systems to ensure air purification. To avoid cross-contamination, internal filters must be disposed of as medical waste during air purifier maintenance. As a result, alternative biofiltration technologies are currently in the works
[38]. In terms of temperature regulation, most climates may reduce or remove automated temperature management by incorporating a temperature control approach into the architectural design process of buildings
[39][40].
The majority of the hospitals spaces and wards are not integrally designed with infectious diseases in mind. During both disease outbreaks and routine conditions, renewed considerations should be given to general building designs to meet different specifications for diverse operational requirements and patient needs. Separating the thermal space conditioning means from ventilation provisions is one such consideration and is an approach that can also promote improved energy efficiencies. Moreover, future designs need to review the best ways of triaging and completing original examinations of patients that present symptoms associated with infectious diseases to reduce exposures to spaces with other types of patients. The healthcare administrators, building operators, designers, and architects in future planning must aspire to building designs that can reduce the flow and connection between common areas and accommodate periods of improved social distancing, while also affording efficient space-use flexibility during ordinary working conditions.
3.2. In Post-COVID-19 Architectural Design: An Auxiliary Decision-Making Strategy
The present pandemic has strengthened modeling skills, and architects are being sought to aid in research using digital simulation techniques to analyze the dynamics of COVID-19 particles
[41]. Architects must comprehend the possible threats in and around a building at the scale of a building. Recently, the researchers’ understanding has steadily increased through computer applications (CAD) to simulate air dispersal as well as natural ventilation. Furthermore, the advancement of computational fluid dynamics (CFD) and building information modeling (BIM) has made airflow modeling tools more accessible to architects. CFD models are commonly used by mechanical engineers to depict how air travels across space and the efficacy of ventilation systems. These techniques have now been repurposed for a better understanding of how infections spread through structures
[37]. The architectural design process is considerably advanced by cooperation between the engineering staff of architects and mechanical engineers and by using CFD models. These technologies can provide researchers with predictive models and help them fill the information gap about airborne viral transmissions in built settings and the efficiency of artificial ventilation systems, leading to a more resilient architecture
[42][43].
Future designers and architects should also gain a better understanding of modeling applications. Modeling applications use various digital technologies for analysis, simulation, and evaluation of the characteristics that help ventilation work well, to alleviate the airborne spread of viruses
[37]. In short, the COVID-19 pandemic has been a considerable shock; on the other hand, the good news is that it has highlighted the importance of social science. The designers and architects collaborate to produce novel concepts for the community’s amenities.
4. Proposed Roles by Real Estate to Mitigate Transmission of COVID-19
It is found that with the outbreak of the COVID-19 pandemic, the relationship between human health and the built and ocean environment is becoming more apparent. While it takes a lot to know about this virus, there are several urgent precautions that real estate professionals may take to limit their chance of infection.
4.1. Purification of Indoor Air and Accentuating Ventilation of Buildings
Modifications to air purification procedures may take longer to implement; this strategy is worth noting since it can assist architects, engineers, and property managers in better planning for the future. Filtration of recirculated air, according to research, may be useful in preventing the transmission of airborne infectious diseases. The HEPA system can remove up to 99.97% of particles with a size of 0.3 μm and larger when operating at total capacity. These filters effectively trap viral particles dispersed by droplet nuclei, as well as dust, vapors, germs, and fungus
[44]. According to research, the use of the HEPA system to recycle air reduces the particulate concentration in indoor air just as much as using a full outside air system under certain situations
[45]. It is reported that ventilation rates significantly influence the droplet nuclei concentration decay
[46]. With the concentration decay equation, it is reported that with 12ACH 1200 s is needed to reduce concentrations to 1.80%. On the other hand
[46], with 24ACH, it requires only 600 s. Moreover, it is reported that since recycled air has become a significant part of the modern building system
[47], providing fresh air to the population is critical to preventing airborne infectious diseases. In contrast, buildings without HVAC systems adopt another alternative to relying on natural ventilation by allowing the entry of natural air
[48].
4.2. Airborne Dissemination
Pathogens spread via the air via droplets and aerosols produced by coughing, sneezing, screaming, breathing, toilet flushing, some surgical treatments, music, and speaking
[49]. Most larger released droplets are gravitationally pulled to surfaces within 1–2 m of the source
[50][51]. Short-range transmission is unaffected by general dilution ventilating or pressure differentials. Airflow patterns in an area in general, and airflow patterns around the origin in particular, might influence the spread of smaller infectious aerosols, particularly droplet nuclei caused by desiccation
[52].
Small aerosols with 10 µm are especially important because they can remain for long periods in the air and have the potential to transmit infection (lasting up to several days in some cases). In addition, their micro size allows them to travel great distances, exposing unaware people with the original infection host
[50]. Droplet-borne infectious diseases have much higher rates of transmission when healthy people are close to infected people, within 1–2 m of each other The surrounding environment conditions have an essential role in reducing the size and number of droplets through evaporation before they stick to surfaces with a diameter of 100 μm and converting them to aerosols of about 10 μm
[53]. Droplet nucleation is used to describe droplet moisture loss due to evaporation and its transformation into an aerosol
[54]. While ventilators cannot stop the quick settling of big droplets, they directly affect the spread of infectious disease droplet nuclei. Clean flow patterns can be induced into and transported by infective aerosols, to be trapped or expelled by directed airflow patterns
[50].
4.3. Preserving Optimal Humidity and Emphasizing Cleaning Protocols
Viruses appear to do better in low-humidity environments, according to the evidence. Therefore, buildings can enhance humidity by installing new portable humidifiers throughout the facility to maintain an appropriate range of 40 to 60%
[55]. Another viable option is to adjust cleaning processes to match the situation’s needs and increase the frequency of cleaning, restock cleaning supplies in advance, and keep hand soap, paper towels, hand sanitizer, and tissues on hand in the bathrooms
[56].
It can be concluded that there is a need for complete coordination between all engineering disciplines to reach healthy and safe urban designs, facilities, buildings, etc. This is done by using simulation programs to match all engineering disciplines and their needs to reach a safe and healthy built environment.
This entry is adapted from the peer-reviewed paper 10.3390/buildings12050582