Infrared radiation (IR), also known as infrared light, consists of electromagnetic radiation with wavelengths but shorter than microwave radiation and longer than visible light (750 nm to 1 mm). IR derives from thermal energy; hence, material bodies emitting heat can produce it. Higher temperatures lead to the production of higher amounts of IR of higher frequency and shorter wavelength. IR consists of a promising complementary treatment for a wide range of musculoskeletal conditions, including knee osteoarthritis, fibromyalgia, chronic low back pain, chronic myofascial syndrome, sacroiliitis, and Gulf War Illness. Infrared therapy can be delivered with light-emitting devices (photobiomodulation), wires, ceramic materials, and IR saunas. In a number of studies, infrared therapy has also been associated with clinical and perceptual improvement in chronic-pain-related psychosomatic symptoms such as fatigue and insomnia.
1. Introduction
The effort to treat musculoskeletal conditions with physical means is as old as humanity
[1]. During the last decades, a growing body of research has focused on the use of infrared radiation (IR). The International Commission on Illumination (CIE) has divided IR into three bands according to its physical properties; near-infrared (IR-A, 700–1400 nm), mid-infrared (IR-B, 1400–3000 nm), and far-infrared (IR-C, 3000 nm–0.1 mm)
[2][3].
1.1. Production and Provision of IR in Clinical Settings
IR for clinical use can be produced by luminous and non-luminous generators
[2]. In the first case, a pre-heated wire in a non-light emitting lamp is used as a generator. In the second case, a lamp is used to produce a combination of infrared, visible, and ultraviolet light. Visible and ultraviolet light are filtered on the surface of the lamp
[2]. The penetration of IR to the human body varies depending on the wavelength of infrared light and to the structure, vasculature, and pigmentation of the skin. The shorter the wavelength, the greater the penetration depth; in particular, IR-A can penetrate up to 5 mm. IR-C and IR-B have smaller penetration depths
[4]. Although IR can directly reach only the epidermis, mechanical conduction of heat enables it to affect deeper layers of the skin and the fascia, with some textbooks reporting a penetration depth of up to 4 cm
[2].
IR therapies can be performed with luminous or non–luminous lamps, clothing with IR–emitting ceramics, and IR saunas. Depending on their intensity, lamps need to be placed at a distance between 45 and 75 cm from the body. Exposure should be limited to 10–15 min, and eye coverage is recommended
[2]. Tourmaline and nephrite-based ceramics have been used by patients with chronic pain or elite athletes
[4], while far-infrared saunas entail 15 min long rest in a heated chamber (60 °C) followed by 40 min long recumbency and wrapping in thermal blankets
[3].
1.2. Effects of Infrared Light on Health
From a mechanistic point of view, IR can affect living tissues from cellular to organ system level. At a molecular level, IR affects the rotation of molecules contained in body fluids and tissues. The potential clinical and physiological effect depends on the composition of the tissue and the proportion of biomolecules contained in body fluids
[5][6]. At the cellular level, the interaction between IR and living tissue boils down to heat–induced alterations of cell membrane potentials by means of a rise in intracellular Ca
2+ levels. This occurs due to increased membrane permeability for Ca
2+ and increased Ca
2+ release from the endoplasmic reticulum as a response to reactive oxygen species (ROS) generated because of the exposure of cells to radiation
[7][8][9]. At the tissue level, a rise in the levels of Nitric Oxide (NO), an endogenous antioxidant substance countering the production of ROS, is associated with oxidative stress reduction and vasodilation. Simultaneously, it stimulates growth factor production and extracellular matrix deposition promoting tissue repair
[10]. In this sense, IR-associated improvement in blood circulation can promote injury and pressure sores healing, decrease muscle spasms and improve the sensory nerve conduction velocity, and potentially increase endorphins modulating pain—the latter might be regulated by other factors as well
[5]. At large, exposure to IR has been considered as an analog of exercise conveying similar benefits to either athletes or patients receiving IR sessions. Certainly, several aspects of the interaction of IR with the neuromusculoskeletal tissue are yet to be clarified.
A summary of the hypothesized and investigated biological and clinical effects of IR therapy with a focus on the musculoskeletal system is presented in Figure 1.
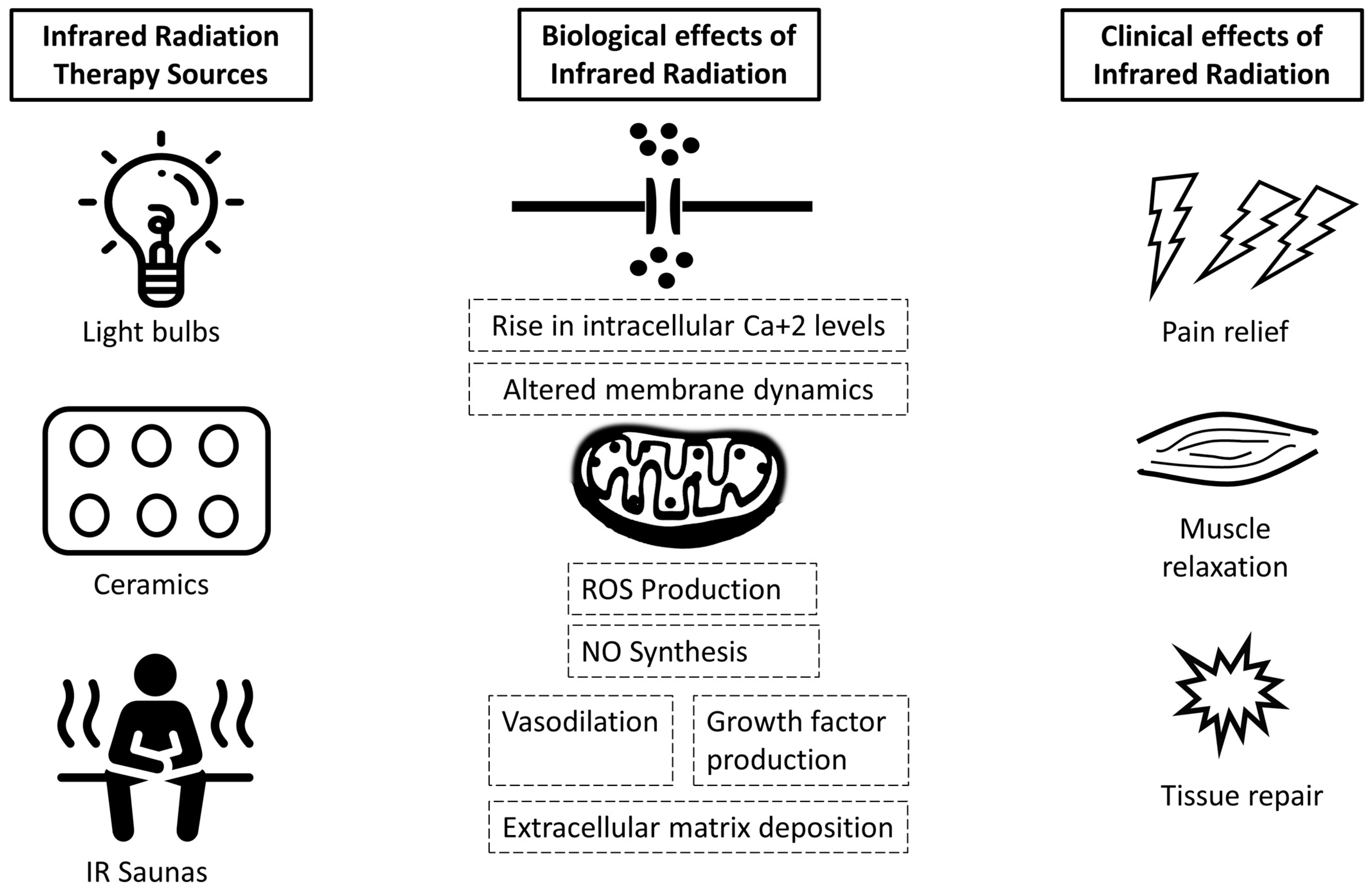
Figure 1. Schematic presentation of IR therapy means of treatment and hypothesized biological and clinical effects with a focus on the musculoskeletal system. Briefly, exposure to infrared radiation leads to an intracellular increase in reactive oxygen species (ROS) and a subsequent rise in nitric oxide (NO) synthesis and calcium intracellular levels (Ca2+). Eventually, this decreases oxidative stress, induces vasodilation and stimulates growth factor production and extracellular matrix deposition leading to tissue repair. Abbreviations: reactive oxygen species: ROS, nitric oxide: NO, calcium: Ca2+.
2. Infrared Radiation in the Management of Musculoskeletal Conditions
Herein showed that IR has significant potential in reducing musculoskeletal pain. The majority of the reported studies suggest that IR could be beneficial as a complementary therapy for patients with FM, osteoarthritis, and other musculoskeletal conditions and athletic injuries associated with chronic pain and physical and psychological burden. IR efficacy in low back pain is ambivalent, with IR appearing more effective than low-level laser therapy in the treatment of chronic low back pain
[11], but not significantly better than moxibustion therapy
[12] and placebo visual-wavelength phototherapy
[13]. The latter was observed in a one-year-long RCT involving a double-sized patient population, and, therefore, it creates considerable concerns about the efficacy of IR in low back pain
[13].
The role of IR in facilitating recovery following sports injuries was also ambivalent. The clinical, neuromuscular, and biochemical findings of Nunes et al.
[14] were in line with recent analyses focusing on phototherapy in sports injuries
[15]. This was in contrast to the findings of Foley et al. that indicated IR phototherapy as an effective means of pain relief and RTP time acceleration. Although a definitive comparison is not feasible, it seems that regular phototherapy sessions are preferable to ceramic clothing
[16].
The majority of the included studies focused on phototherapy and IR emitting ceramic materials, while fewer studies reported on IR saunas. Given the heterogeneity in methods and health conditions, it is not possible to reach a definite conclusion regarding the preferred IR treatment means. The heterogeneity of population sizes and characteristics does not lead to decisive evidence about age, gender, or patient groups that can benefit more from IF therapy.
Previous research has expressed safety concerns, implying that IR may be associated with thermal burns, skin irritation, eye damage, dehydration, hypotension, and defective arterial blood flow. On these grounds, IR has been contraindicated in patients with impaired cutaneous thermal sensations, superficial circulation defects, dermatitis or eczema, cancer, ionizing–radiation-associated skin damage, fever, and active infections
[17][18][19]. Here, both the studies that showed the effectiveness or the supremacy of IR over other treatments and those that considered IR inferior to other therapeutic modalities did not express concerns regarding its safety. Previous research has identified pain, burn injury, and infection as key safety concerns
[20]. Nevertheless, such adverse effects were not documented in the studies that herein concluded. Therefore, it appears that IR is safe in the above-mentioned conditions and delivery methods. These results encourage the clinical use of IR and urge for larger studies focusing on patients rather than healthy individuals in order to outline the indications and the expected benefits from the use of IR.
From a methodological point of view, the majority of the studies employed perceptual and clinical indexes in order to measure the effectiveness of IR, with only a few studies involving biochemical and/or neuromuscular parameters
[14]. In most cases, IR was evaluated as a complementary therapy, and the conventional treatment of the reported patients was not discontinued. Although this can be considered as a source of controversy, pre- and post-treatment measurements served as a comparison that elucidated the effect of IR therapy. On top of this, discontinuing the mainstay treatment of patients with chronic conditions might not have been possible on ethical grounds.
3. Conclusions
Overall, IR appears as a safe and effective complementary therapy for a number of musculoskeletal conditions, including knee OA, fibromyalgia (FM), and chronic myofascial pain. Its efficacy remains debatable when it comes to low back pain and muscle damage in sports injuries. Given the diversity of therapeutic means and applications, further research is necessary in order to establish the optimal indications and treatment plan for the clinical use of IR in musculoskeletal medicine.
This entry is adapted from the peer-reviewed paper 10.3390/ejihpe12030024