On the basis of the myriad benefits of nanotechnology in agriculture, nanofertilizers (or nanonutrients) have become promising tools for agricultural sustainability. Nanofertilizers are also the proper solution to overcoming the environmental and health problems that can result from conventional fertilizers. The role of nanofertilizers has increased, especially under different environmental stresses, which can include individual, combined, and multiple stresses. The stresses are most commonly the result of nature; however, studies are still needed on the different stress levels. Nanofertilizers can play a crucial role in supporting cultivated plants under stress and in improving the plant yield, both quantitatively and qualitatively.
1. Introduction
Nowadays, food security faces a great challenge, which is persistently represented in the rapid increase in the global population and the drastic changes in the climate
[1]. This food security mainly depends on the agricultural sector, and particularly on crop production, which supplies the human diet with the major sources of the bioactive compounds for human nutrition
[2][3]. This productivity faces different stresses, which may produce losses of up to 50–70% in the case of abiotic stresses, and of up to 40–60% in the case of biotic stresses
[4]. The most common abiotic stresses include salinity, drought, flooding, and heat stress, whereas bacteria, viruses, and fungi are the key biotic stresses. These stresses can be mitigated in cultivated plants through the application of a variety of antistressors, such as nitric oxide
[5]; pyraclostrobin
[6]; rhizobia, or plant-growth-promoting rhizobacteria (PGPR)
[7][8]; melatonin
[4]; strigolactones
[9]; phytohormones
[10]; and nutrients such as silicon
[11][12] and selenium
[13], as well as their nanoforms
[9][14].
Nanofertilizers are considered to be promising candidates for the fertilizer industry, and they have the considerable potential to improve the retention of different nutrients for optimal crop production
[15]. Nanofertilizers may be crucial for plant nutrition and human health
[2], particularly the current nanofertilizers, which are highly efficient (50–70%) compared to traditional fertilizers (40–50%) in terms of the controlled release of the nutrients
[16]. These nanofertilizers can solve the main problem of traditional fertilizers (i.e., the high nutrient losses in the soil) by allowing the slow and sustained release of nutrients over an extended period. They also have high reactivities because of their small sizes, high surface areas, and increased productivity
[17]. Several nutrients have been used through soil and/or foliar application in cultivated plants (e.g., Cu, Mn, and Zn, which support crop productivity
[15]), as has been reported for the following studied crops: wheat
[18], maize
[19], tomato
[20], sweet basil
[21], lettuce
[22], cabbage
[23], and strawberry
[24].
2. Nanofertilizers and Agriculture
2.1. Nanofertilizers for Crop Production
Nanotechnology can be defined as the science of the production of nanoparticles through the modification and/or self-assembly of individual atoms, molecules, or molecular clusters, which are endowed with new or drastically different properties
[25]. Nanotechnology has several applications in all sectors, including agriculture, industry, medicine, pharmacology, etc. In the agriculture sector, nanotechnology applications can be broadly classified into the following main sectors: (1) Crop production through the use of nanoherbicides/nanopesticides and nanomaterials to control the viral and fungal diseases of plants; (2) Precision farming; and (3) Crop improvement using nanofertilizers and nanobiosensors for soil/plant systems, and for the nanoremediation of soils, water, and the food sector
[26][27][28]. Although agro-nanotechnology has several benefits, nanomaterials may also be associated with many risks, which include: (1) Safety issues that are due to the penetration of the cells, which are small sizes; (2) The degradation of the nanomaterials and/or nanocomposites under environmental conditions, with the release of the inserted nanomaterials into the environment; (3) A significant amount of leaching of the nanoparticles into the agro-environment, which may cause nanotoxicity; and (4) The integration of many nanoparticles into the human body via inhalation, ingestion, or cutaneous contact
[28].
2.2. Nanofertilizers vs. Traditional Fertilizers
There are many problems that result from the intensive use of traditional or chemical fertilizers that have led to many negative impacts on the agroecosystem, such as the low efficiency of the nutrient use by crops, and the high losses of nutrients to groundwater, especially NPK (by 40–70%, 80–90%, and 50–90%, respectively
[29][30]). Thus, nanotechnology has become an emerging approach in the revolution of agricultural systems through its controlled-release manner of delivering nutrients to cultivated plants
[31]. Nanofertilizers are a type of fertilizer, and they are synthesized using physical, chemical, or biological methods that are based on nanotechnology. The physical methods induce rapid synthesis, but they produce many impurities; the chemical methods are precise in terms of the size of the nanoparticles, but they may produce toxicity; and the biological methods are eco-friendly and low toxic, but they induce slow synthesis
[29]. These nanofertilizers can enhance crop production by supplying one or more nutrients to the cultivated plants via smart delivery, which results in sustained nutrient release, improved plant growth, high nutrient uptake efficiency, and improved soil quality
[32][33].
2.3. The Nanofertilizer Industry and Its Obstacles
However, the nanofertilizer industry faces many challenges, which represent important and real obstacles. These risks include the release and reactivity of nanofertilizers, which depend on the environmental factors and that can cause phytotoxicity effects, depending on their doses and the durations of long-term exposure. This may lead to chronic effects on health of consumers
[25]. The physical and chemical methods of nanofertilizer synthesis have recorded higher toxicities compared to the biological methods, which are still under intensive investigations
[34].
2.4. Nanofertilizers for the Mitigation of Stress on Plants
Nanofertilizers have a great ability to mitigate the abiotic/biotic stresses on cultivated plants through many mechanisms because of their vast surface areas and their nanoscale size. Nanofertilizers can improve the morphological, biochemical, and physiological indices of cultivated plants, such as the photosynthetic rate and its efficiency, the nutrient uptake efficiency, the regulation of phytohormones, and the enhancement of the plant defense system (
Figure 1)
[35]. Therefore, the mechanisms of nanofertilizers on the crop productivity under stress may include: a reduction in the oxidative stress, which leads to an increase in the stress tolerance of the plants; the enhancement of several biochemical activities in stressed plants (e.g., by increasing the contents of the proline, chlorophyll, and relative water); the regulation of the salt toxicity; a reduction in the accumulation of malondialdehyde and H
2O
2; and the maintenance of the ionic equilibrium, depending on the type of stress
[36].
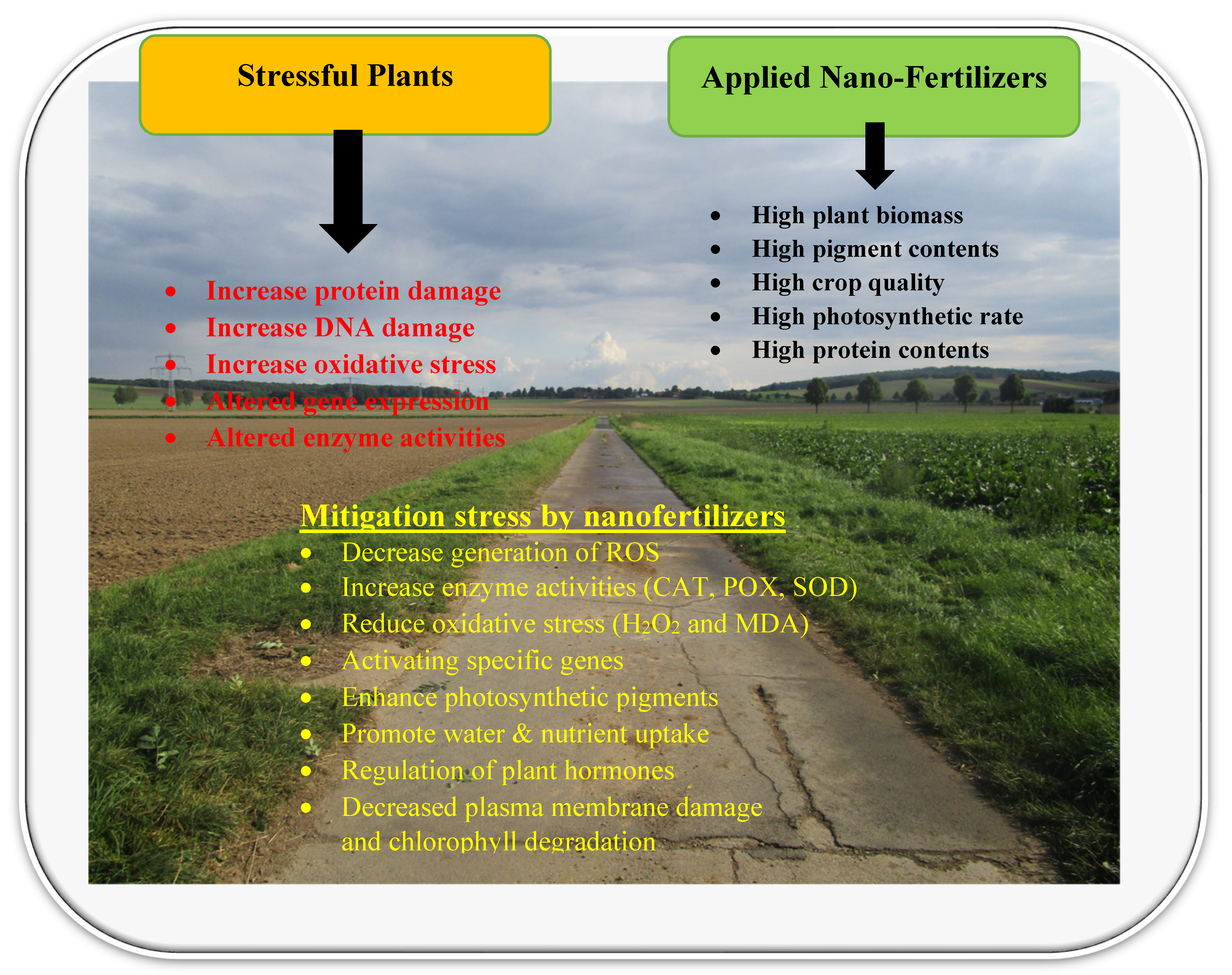
2.5. Nanofertilizers and Their Research Gap
It is well known that cultivated plants need many essential and beneficial nutrients for their growth, which can be classified on the basis of their relative essentiality, their function, and their mobility in plants and soils
[37]. There are three groups of essential mineral nutrients that uptake in ionic form from the soil solution. These groups include (1) Essential and beneficial nutrients (based on their relative essentiality); (2) A group of basic, accessary, regulatory, and catalyst nutrients (based on their physiological function); and (3) A group of mobile, intermediate, or immobile nutrients (based on their mobility in plants or soils).
2.6. Nanofertilizers and Phytotoxicity
There are research gaps that are related to nanofertilizer production, including with regard to the energy use and the technology expense, as well as with regard to their unknown interactions in the environment and their toxicities. There are several reports that are based on the phytotoxicities of nanoparticles in crops, which are common, and which result from the induction of the synthesis of reactive oxygen species (ROS) and the oxidative damage from the use of many metal-oxide nanoparticles, such as AgO, CeO
2, CuO, NiO, TiO
2, and ZnO (e.g.,
[35][36][38]).
2.7. Nanofertilizers and Climate Change
It is well known that nutrients can be lost from agricultural fields through the leaching process and/or through gaseous emissions, which may lead to environmental pollution and climate change. These environmental issues may be mitigated by applying nanofertilizers, even under stress
[39]. There are a few studies that have been published on the impact of applied nanofertilizers on reducing gas emissions to the atmosphere (such as
[40][41]). Nanoparticles are the most important adsorbents in soil, and they can affect the nutrient transportation, the organic matter fixation, and the precipitation of the new mineral phase. In the future, the presence of nanoparticles in intact soil formations will be crucial. Furthermore, nanoparticles have direct effects on plants, which include increased enzyme activity, better seed germination, increased plant tolerance to adverse conditions, increased carbon sequestration and nitrogen fixation, and increased photosynthetic and respiratory activities
[42]. Therefore, the role of nanofertilizers in mitigating climate change is an urgent global concern.
3. Application of Nanofertilizers
The intensive global production of foods requires the correct and proper amount of applied nutrients. However, the primary sources of nutrients were, for a long time, the chemical forms of the nutrients, which caused an environmental crisis. Nowadays, the organic, bio-, and nanoforms of these nutrients are applied at an ascent rate to replace the chemical forms of the nutrients
[43]. Nanofertilizers may achieve sustainability in agriculture through their high use efficiency and by minimizing the pollution of the environment
[44]. These nanofertilizers, as nanofertilizers, may also improve the crop productivity by increasing the bioavailability of these nutrients in the soil, as well as their uptake by cultivated plants. The advantage is the self-regulated, time-controlled, and spatially targeted delivery of the active ingredients
[45]. Nanofertilizers can be defined as a type of fertilizer that contains nutrients in the nanoscale form, and/or nutrients in encapsulation, which can systematically release different nutrients to targeted plants
[22]. Nanofertilizers represent any applied nanomaterials for which the nutrients are converted into the nanoscale, and that act as plant nutritional mediators that mainly provide nutrients to the plant in order to support its growth
[45][46]. These nanofertilizers can be encapsulated through nanocoating nanoporous polymeric materials, which are supplied in the nanoforms of emulsions, or as slow-release fertilizer particles
[45].
4. Crop Response to Applied Nanofertilizers under Individual Stress
In nature, plants may be exposed to one or more stresses (i.e., biotic and abiotic stresses), either individually or in combination, which ultimately cause losses in the crop yields. These stresses include abiotic stresses, such as drought, salinity, flooding, water deficits, and low and high temperatures (cold and heat stress), as well as biotic stresses, such as diseases or the pathogens of viruses, bacteria, and fungi
[47]. These plants can face individual or multiple stresses during their lifecycles, as is reported by several studies
[48]. Many antistressors, such as nanofertilizers, have been applied in order to support plant growth under stress, particularly nanoselenium for salinity stress
[49], nano-silicon for drought
[50], and nano-copper for salinity stress
[51], which can increase the plant tolerance against abiotic and biotic stresses (
Figure 2;
[17]). Plant nutrients have a prominent role in ameliorating several stresses, as is cited by many researchers, such as Ahmed et al.
[52]. Several reports confirm the significant role of plant nutrients under different stress conditions, such as salinity stress
[28], heat stress
[53], heat and drought
[54], and drought
[55].
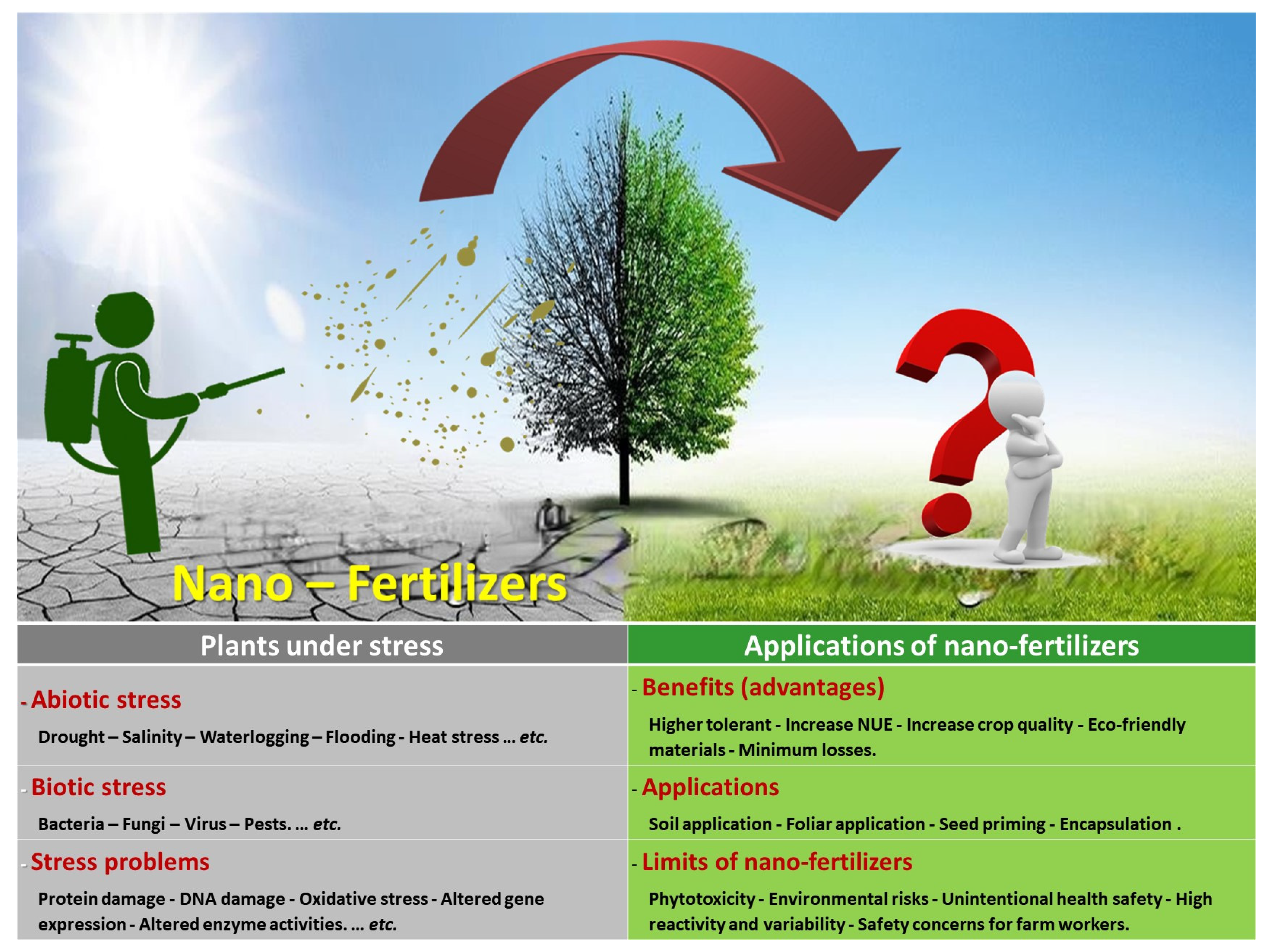
5. Combined Stress and Applied Nanofertilizers
After several studies on the effects of individual stresses on cultivated plants, many studies have begun to investigate combined stress, which is more representative of the reality of nature
[56][57]. This combined stress may include abiotic stress, such as drought; biotic stress, such as bacterial infection (
Pseudomonas syringae)
[58]; or two different abiotic stresses, such as salinity and heat stress
[14]. The damage of this combined stress depends on the duration and the type of stress, the type of plant and its growing stages, the soil and its amendments, and the type of growth medium
[59]. The most common case studies of combined stress include soil salinity and heat stress [14;18], salinity and drought
[60][61][62][63][64][65]; drought and heat stress
[39][66][67]; and soil salinity and heavy metal stress
[68][69]. Nanofertilizers have already been applied to stressed plants, such as nano-Se to cucumber under salinity and heat stress
[14][70], and SiO
2 NPs to common beans under soil salinity and heavy metal stress
[69].
6. Applied Nanofertilizers under Multiple Stresses
Recently, there has been an urgent need for studies on environmentally stressed plants that closely imitate the field conditions (i.e., combinations of stresses instead of individual stresses), as there are serious challenges facing the global crop productivity
[71]. This requires the development of different strategies for making agriculture more resilient while reducing the negative impacts of the combination of drought and heat stress on crop productivity. These strategies may include many practices and superior crop varieties, and they may include the application of biofertilizers, which have the potential to improve the plant tolerance to the combined stresses
[66]. The effects of multiple stresses largely depend on the plant age; on the inherent stress-resistance of the plant, or the susceptibility of its nature; as well as on the severity of the stresses. The plant responses under combined stress comprise the morphophysiological, generic, and molecular features that result from these stresses
[71]. Under salinity, drought, and chilling stresses, osmo-protectants may accumulate, which is due to the induction of the osmotic effect on stressed plants. In general, ROS production may occur under almost all abiotic stress conditions. Moreover, it is well known that salt and heat stress commonly impact the transport and compartmentation of the ions in plants, whereas a physiological water deficit in plants is created because of drought and salinity stress, as well as a decrease in the CO
2 diffusion in the chloroplast because of the reduction in the stomatal opening, which leads to a reduction in the metabolism of the carbon
[71]. Under drought and heat stress, tea plants have resistance to these stresses through the accumulation of metabolites, such as caffeine, catechins, and theanine
[72].
7. Conclusions
Nanofertilizers are an essential source of fertilizers, which can improve the crop production, compared to traditional chemical fertilizers. Nanofertilizers have many advantages, which include their slow/controlled delivery of nutrients, and their abilities to reduce the loss of nutrients in soils by adsorption or fixation, increase the bioavailability of nutrients, extend the effective duration of the nutrient release in the soil, and increase the efficient use of nutrients. On the other hand, nanofertilizers have some problems because of their high reactivity and variability, and their phytotoxicity to plants. There are also human health risks, such as safety concerns for agricultural workers and consumers. Nanofertilizers should definitely be evaluated very carefully before marketing in order to examine not only their advantages for plant growth, but also their potential limitations in terms of the environment and human health. A genuine relationship between nanofertilizers and the plant stresses, in their individual or combined states, has been reported in the published literature; however, there are only a few studies that have been published on multiple stresses. The cited studies confirm the positive role of nanofertilizers on crop production under single and/or combined stress; however, their role under multiple stresses requires more effort and investigation.
This entry is adapted from the peer-reviewed paper 10.3390/su14063480