Brucellosis is caused by the intracellular pathogens from genus
Brucella [34].
Brucella spp. can multiply within phagocytic cells with human beings as end hosts
[35]. Four species of
Brucella can infect humans:
B. abortus, B. canis, B. melitensis, and
B. suis. Of these species,
B. melitensis is the most commonly isolated from ruminants
[16]. In sheep and goats, which are the main hosts of
B. melitensis, the bacterium causes impaired fertility and abortions
[36]. Brucellosis in cattle is caused by
B. abortus, which can be easily transmitted to humans. Brucellosis may be transmitted to humans through contaminated food and dairy products, occupational contact, or inhalation of infected aerosols
[16]. Another important route of infection is the contamination of mucous membranes or open wounds with fetal fluids, making veterinarians, farmers, and abattoir workers the most vulnerable to infection. In other cases, transmission from animals to humans is mainly associated with drinking contaminated milk
[37]. Human-to-human transmission takes place through lactation, sexual intercourse, and tissues such as blood transfusion and bone marrow transplantation
[38]. Brucellosis in humans has several, often non-specific, presentations, including a systemic syndrome (fever, sweat, chills, and fatigue), but also some located presentations (epididymoorchitis and spondylodiscitis). Severe forms of this disease are neurobrucellosis and endocarditis
[38]. Brucellosis is one of the most frequent zoonosis in many parts of the world. However, this disease mainly affects humans in developing countries, as it is effectively controlled in developed countries
[16]. Brucellosis is an endemic zoonosis for the Middle East, the Mediterranean rim, Asia, Africa, and South and Central America. These are regions with a very high consumption of dairy products and insufficient animal health care
[37]. Around 500,000 cases of brucellosis in humans are reported worldwide each year
[36]. However, true incidence is estimated to be 5,000,000 to 12,500,000 cases annually
[39]. Seroprevalence by country in sub-Saharan Africa is 24.1% and 31.82% in Nigeria, 17% in Uganda, 7.7% in Tanzania, 3.8% in Chad, and 3.3% in the Central African Republic
[40]. Brucellosis is a major economic problem in African countries such as Nigeria. In this country, high losses are generated for cattle producers due to stillbirths, reduced calving percentages, medical costs, births of weak calves, culls due to infertility, and the loss of man-hours in infected people. Furthermore, wastes in meat and dairy production are estimated at USD 224 million per year. To compare, in the Republic of South Africa, the losses due to brucellosis are USD 37.5 million and in the USA, they equal to USD 800 million per year
[41]. According to the EU One Health Zoonoses Report, 310 human cases of brucellosis have been reported in the EU in 2019
[5]. As reported by the CDC, areas currently listed as high risk of brucellosis are the Mediterranean Basin (Portugal, Spain, Southern France, Italy, Greece, Turkey, and North Africa), Mexico, South and Central America, Eastern Europe, Asia, Africa, the Caribbean, and the Middle East
[6]. In the United States, brucellosis is a rare disease, with 80–120 cases reported annually
[6]. Syria has the highest number of human brucellosis with 1603.4 cases per 1,000,000 individuals. This is followed by Mongolia (3910), Iraq (268.8), Tajikistan (211.9), Saudi Arabia (149.5), and Iran (141.6)
[39]. In China, brucellosis is also an important public health threat. In 2014, 4.2 cases/100,000 people were reported
[42].
4. Tuberculosis Caused by Mycobacterium bovis and Mycobacterium caprae
Mycobacterium caprae and
Mycobacterium bovis are members of the
Mycobacterium tuberculosis complex and cause tuberculosis (TB) in animals and humans.
M. caprae is isolated not only from goats but also from sheep, red deer, cattle, wild boar, the Siberian tiger, camel, bison, and humans
[17].
M. caprae causes lesions and diseases like that of
M. bovis but occurs only in a low proportion of human TB cases. Moreover,
M. caprae is evolutionarily older than its epidemiological twin,
M. bovis. This bacterium is not globally distributed but primarily restricted to European countries
[43]. On the other hand, the most common host of
M. bovis is cattle, but other mammals, such as marsupials, carnivores, pinnipeds, lagomorphs, rodents, and some avian species, could be also infected
[44]. The main route of TB transmission in animals is via aerosol by the droplet nuclei generated during coughing and sneezing. Humans may be also infected through milk, dairy products, and by eating meat from infected animals
[45]. The disease is manifested in humans by fever, fatigue, arthralgia, and muscle pain, and variety of other symptoms depending on the part of the body affected by the disease. WHO reported that in 2016, there were 147,000 new cases and 12,500 people died due to TB, but with no information of the potential zoonotic origin
[18].
M. bovis is responsible only for 3.1% cases, with the exception for Tanzania, in which it reached 16% of TB in humans possibly due to poor zoohygienic conditions
[46]. In 2019, most of the zoonotic TB human cases occurred in Africa (50%) and South-East Asia (31%). Globally, there were 140,000 human cases of zoonotic TB. However, the uncertainty level is estimated to be 69,800 to 235,000
[47]. While in Europe, TB is a rare infection with 147 confirmed cases in humans reported in 2019 in the EU. Between 2015 and 2019, 918 cases of TB were confirmed in the EU, including 54 caused by
M. caprae [5]. The global distribution of zoonotic TB human cases in 2019 is presented in
Figure 2. The most effective way to eliminate TB in farm animals is through implementation of eradication programs. In developed countries, infection with
M. bovis is not common in cattle. This is related to compulsory tuberculin testing, the pasteurization of milk, and the removal of positive reactors
[48]. Before the routine application of milk pasteurization in the United Kingdom (UK),
M. bovis was isolated from 8% of churn milk samples from 3000-gallon tankers in 1945
[49]. In the 21st century, only 315 cases of human TB have been reported in the UK over a 10-year period
[50]. However, it is important to note that cattle can become infected from wild mammals. This can also have an impact on the eradication of the disease. To date, the following have been identified as reservoirs of the mycobacteria around the world: brushtail possum and badger, European bison, African buffalo, wild boar, and white-tailed deer, among many others
[51]. Research shows that Michigan deer may have infected surrounding cattle. Data indicate that while recording cases of the disease in wild deer between 1975 and 1994, infected animals were found in sixteen domestic cattle herds in four counties in the north-western part of the state
[52]. Transmission from humans to cattle is also possible.
M. bovis is usually transmitted directly by inhalation but also indirectly by hay and bedding contaminated with urine. In the Netherlands, humans were the source of transmission for 50 cattle herds
[53]. Zoonotic
M. bovis infections are mainly a problem in undeveloped countries. In the developing countries, due to the lack of control of zoonotic products, poor production hygiene, and outbreaks of other diseases (e.g., AIDS), the pathogen will continue to persist and remain a real challenge for public health in the future.
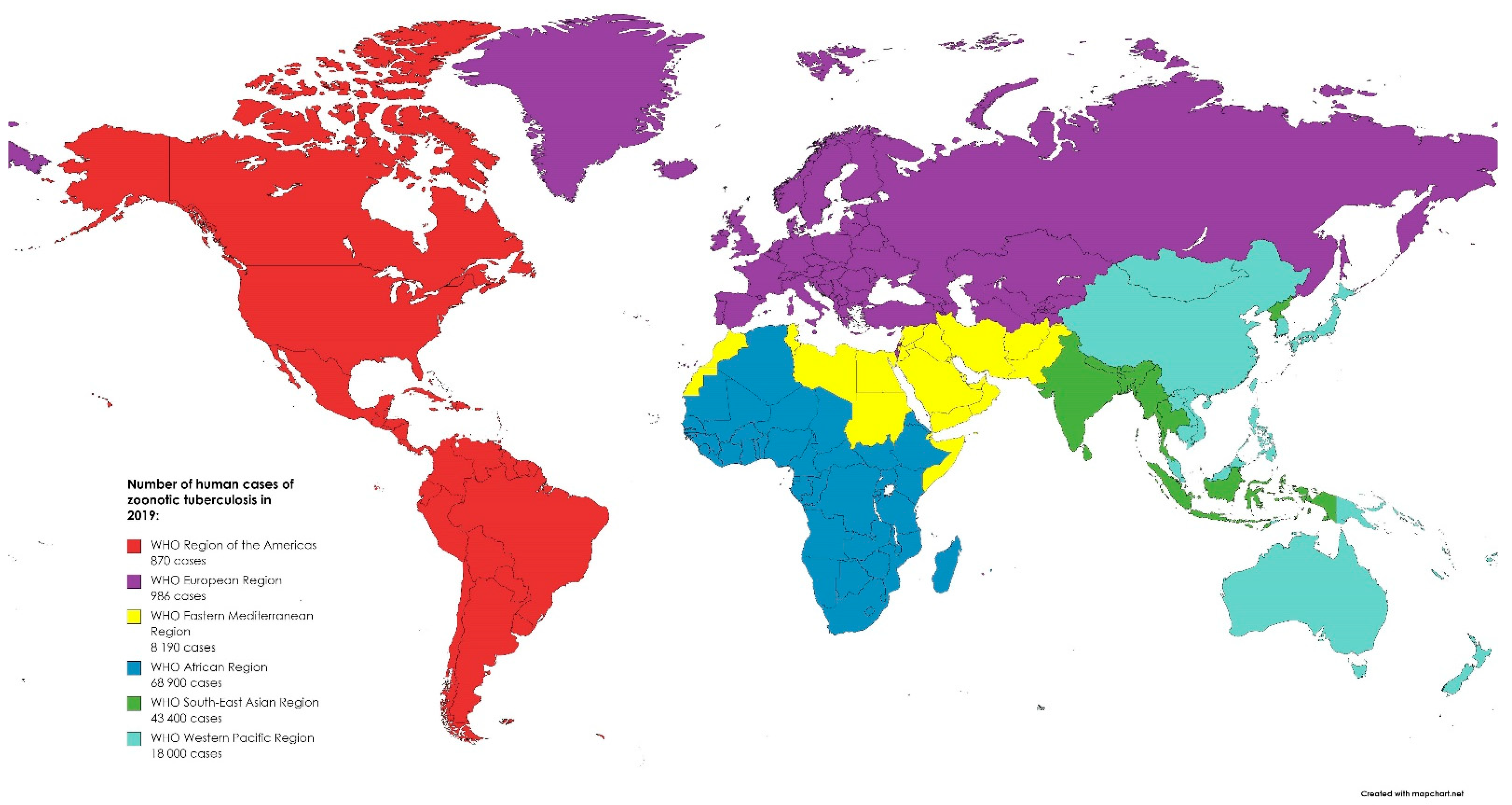
Figure 2. The global distribution of zoonotic tuberculosis human cases in 2019. Created based on data from
[47].
5. Salmonellosis
Salmonella is a large, ubiquitous genus of Gram-negative, rod-shaped, facultative anaerobic bacteria belonging to the family of
Enterobacteriaceae and is responsible for zoonotic infections of global significance. It can be persistent in dry environments as well as in water for months
[54]. There are two main species distinguished:
S. enterica (which includes more than 2600 known serovars) and
S. bongori. The majority of variants of
S. enterica are motile by the means of flagella but the most important virulence factors are invasion and intracellular replication
[54].
Salmonella was first isolated in 1884 by an American bacteriologist, D. E. Salmon, from porcine intestine
[24] and in the 1980s, the first pandemic of
S. enterica s. Enteritidis emerged due to contaminated poultry products
[55].
Salmonella sp. may cause clinical disease in livestock or subclinical infections in asymptomatic animals (carriers), such as dogs and cats, which transmit and contaminate the environment of food-producing animals. A very important role is played by vertical transmission, especially in the poultry and bovine reproduction sector, but pests are also a significant vector of the germ
[25][54]. An infection in humans can occur after drinking contaminated water or ingesting uncooked contaminated eggs, milk, and meat originating from poultry, cattle, or swine, although there have been reports about other foods, including vegetables contaminated by manure and ready-to-eat foods that caused infection. Human-to-human transmission through the fecal–oral route and infection after direct contact with infected animals, their feces, and the environment are less common, although still significant. What is a concern is that
Salmonella can pass through the entire food chain, starting from animal feed contaminated by manure and primary production to the table in households, food services, and institutions (farm-to-fork continuum)
[6][56]. EFSA reported that salmonellosis after campylobacteriosis was the second most often reported gastrointestinal infection in humans. In 2019, 87,923 cases were confirmed in the EU
[5]. According to OIE, salmonellosis qualifies as one of the most common foodborne bacterial diseases in the world. Human infections caused by
Salmonella species are most frequently caused by
S. Enteritidis and
S. Typhimurium, which are normally found in the intestines of humans and animals, as they are the main reservoir of these bacteria
[3][24]. A distinction is made between three major diseases caused by
Salmonella in humans, namely non-invasive non-typhoidal salmonellosis, invasive non-typhoidal salmonellosis, and typhoid fever, but in general, salmonellosis manifests with acute enterocolitis accompanied by inflammatory diarrhea, abdominal pain, fever, nausea, and vomiting in humans
[57]. Most cases of the disease are underdiagnosed, turning salmonellosis into a disease that contributes to the deaths of thousands of people worldwide, especially in economically underdeveloped countries
[54].
6. Conclusions
Human and animals’ health are intimately connected since they share a communal environment. Consequently, pathogen transmission is possible and it may occur via direct and/or indirect contact, including consuming products of animal origin. In the case of confirmed infection derived from animals or animal products, a comprehensive approach should be applied. Thus, in the suspicion of any zoonoses, the medical and veterinary doctors should closely cooperate to protect public health and work in accordance with the One Health conception.