Glioblastoma is the most common and malignant primary brain tumor, defined by its highly aggressive nature. Despite the advances in diagnostic and surgical techniques, and the development of novel therapies in the last decade, the prognosis for glioblastoma is still extremely poor. One major factor for the failure of existing therapeutic approaches is the highly invasive nature of glioblastomas. The extreme infiltrating capacity of tumor cells into the brain parenchyma makes complete surgical removal difficult; glioblastomas almost inevitably recur in a more therapy-resistant state, sometimes at distant sites in the brain. Therefore, there are major efforts to understand the molecular mechanisms underpinning glioblastoma invasion; however, there is no approved therapy directed against the invasive phenotype as of now.
1. Introduction
Glioblastoma is the most common and malignant primary brain tumor [
1]. Despite the advances in diagnosis and treatment, life expectancy still remains at approximately 12–18 months [
2]. Invasion ability towards the surrounding tissue is a determinant for malignant tumor progression [
3]. The high mortality of glioblastoma patients is partly attributed to the tumors’ therapy-resistant nature, as well as to the extremely invasive behavior of tumor cells. Extensive tumor cell infiltration (dispersal) from the primary tumor site into healthy adjacent tissue results in rapid and almost inevitable recurrence. Nearly all patients with glioblastoma, within a 2-year period from the time of primary surgery, experience tumor regrowth. Maximal safe tumor resection is important in terms of decreasing the tumor burden before radiotherapy and chemotherapy, and in reducing the risk of recurrence. However, the fact that healthy brain tissue and the boundaries of the tumor cannot be separated, either by eye or by standard surgical microcopy, makes the maximum safe removal difficult. Taking healthy tissue near the tumor environment during the removal of tumors located in functional areas of the brain or adjacent to the brain poses a risk for neurological sequelae.
The current treatment for glioblastoma patients is the maximal safe tumor resection followed by the Stupp protocol, which is radiotherapy combined with concurrent daily temozolomide, followed by adjuvant temozolomide treatment [
4]. However, the invasive nature of glioblastoma cells limits the therapeutic efficacy. The distant invasion of tumor cells to the brain parenchyma, and their infiltration and cooperation with healthy tissue, are major obstacles limiting complete tumor resection. Indeed, in glioblastomas, tumor borders are diffuse, and individual cells that have infiltrated into the healthy parenchyma are not detectable [
5]. Therefore, the infiltrative cells that escape from surgery can recolonize and cause therapy-resistant recurrent tumor growth. Due to the diffuse nature of these tumors, radiotherapy applications can prove very challenging [
6,
7,
8].
Figure 1 depicts the MRI scans of a glioblastoma patient before surgery, right after the surgical removal of tissue, and 18 months following surgery. Despite quite successful tumor resection and combined radiotherapy and chemotherapy, the tumor recurred in the contralateral hemisphere because of invasive cells infiltrating into the brain parenchyma, serving as an example and a testament to a huge clinical problem.
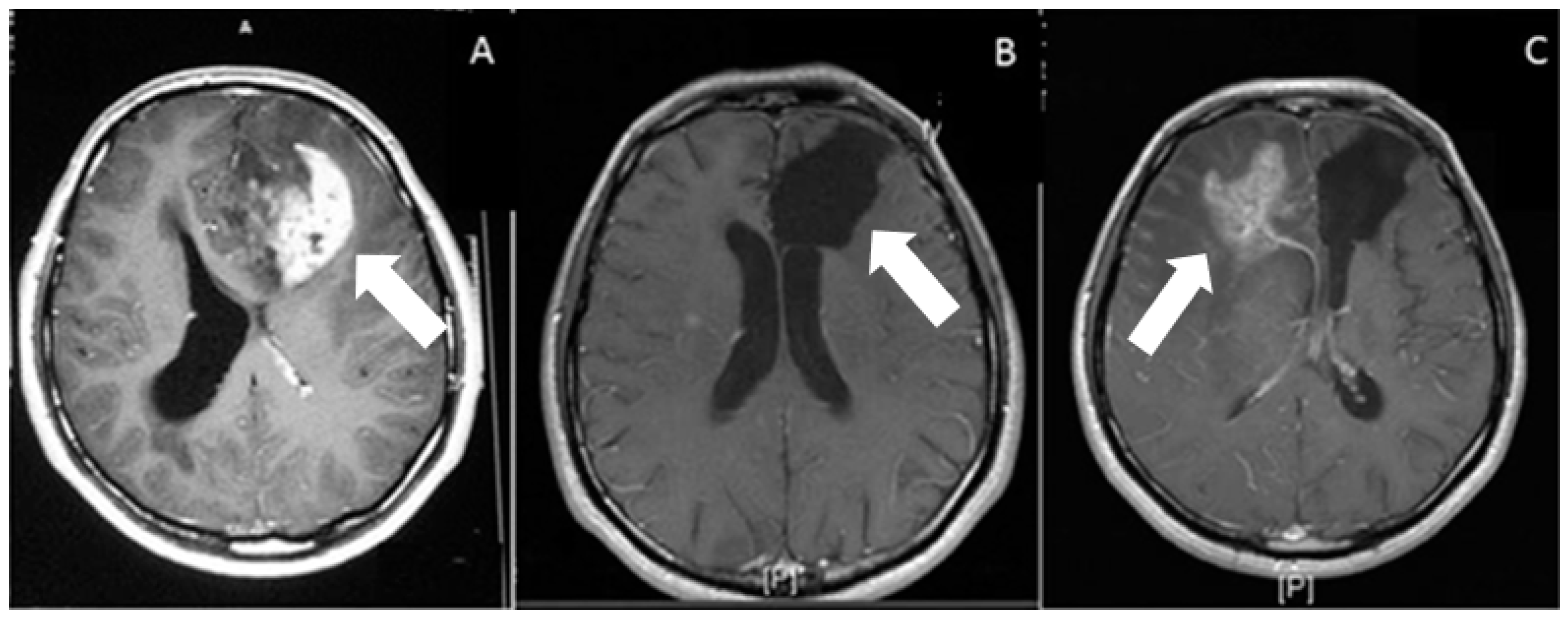
Figure 1. Post gadolinium contrast administration, T1-weighted axial images. (A) Preoperative, heterogeneous irregular enhancement, associated with the left frontal-lobe glioblastoma (arrow). (B) Postoperative (at 1 month) axial weighted image. On postoperative image, there is no residual enhancement. Arrow shows operation cavity. (C) Postoperative (at 18 months) axial weighted image shows recurrence of the tumor (white arrow) on contralateral hemisphere, associated with peripheral edema.
Despite the realization that the invasive nature of glioblastoma cells has drastic results for therapy resistance, high recurrence rates, and poor survival rates, there is no directed therapy to prevent this invasive behavior [
5]. Therefore, understanding the mechanisms of glioblastoma cell invasiveness is of the utmost priority to develop successful therapeutic approaches.
2. Routes of Glioblastoma Cell Invasion
Invasive glioblastoma cells diffusely infiltrate into normal brain tissue, which limits the therapeutic options and increases recurrence rates by inducing the formation of secondary tumors. To interpret the mechanisms facilitating the glioblastoma’s invasive behavior, molecular mechanisms of tumor cell migration, migration routes, and accumulation sites of tumors cells in the brain need to be better understood. The preferred or avoided anatomical structures may affect the growth and invasion pattern of glioma tumors, giving rise to defined anatomical subtypes, such as the optic pathway or limbic gliomas [
9,
10,
11].
The invading cells tend to follow roadmaps that already exist in the brain. They co-opt and move along certain anatomical structures, such as perivascular space and white-matter tracts, and they can reach to distant sites in the brain parenchyma or the leptomeningeal space [
12] (
Figure 2). More specifically, invasive glioblastoma cells have a preference to move along myelinated fiber tracts, such as the corpus callosum and the internal capsule, meninges, ventricular lining, and basement membrane of blood vessels, subependymal space–perivascular regions, and glia limitans externa [
13].
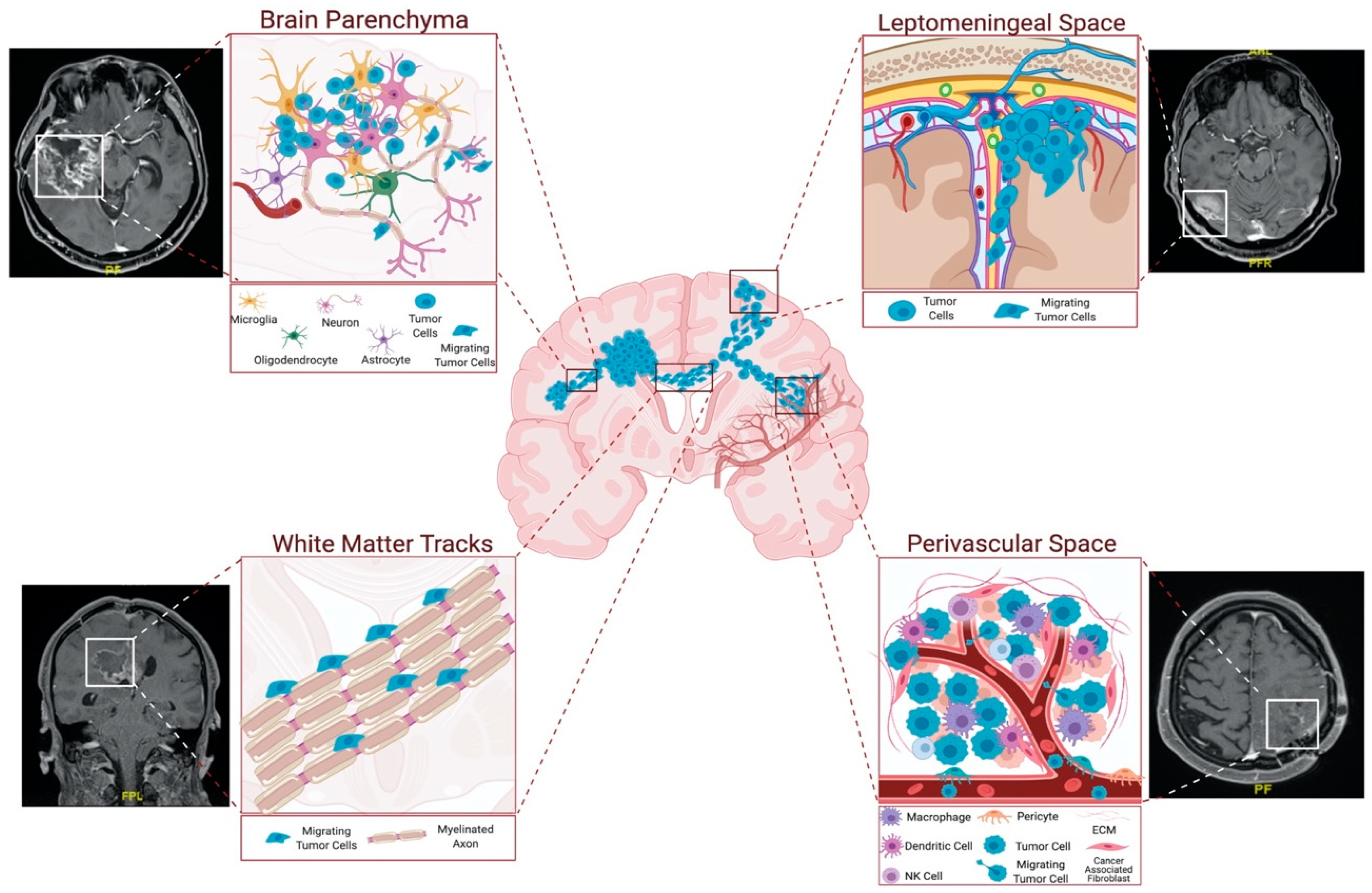
Figure 2. Routes of glioblastoma cell invasion. Glioblastoma cells generally invade using tracts in parenchyma, white-matter tracts, and leptomeningeal and perivascular spaces. Among these, perivascular space and white-matter tracts are the most preferred routes for glioblastoma invasion. Perivascular space attracts the tumor cells with the presence of blood vessels, which provide oxygen and nutrients. White-matter tracts are composed of myelinated axons, and tumor cells exploit these structures to reach distant locations in the brain. Parenchymal cells facilitate glioblastoma invasion by secreting several factors. Figure generated at Biorender.com, combined with representative MRI images from our clinic.
Since the vasculature and white-matter tracts are the primary structural components of the brain, these structures are the most preferred routes for glioblastoma cell invasion. The perivascular space surrounds blood vessels, including arteries, arterioles, and veins. Invasive tumor cells infiltrate the perivascular space to reach into the vessels, which provide oxygen and nutrients. Indeed, several studies have shown the significance of blood vessels as routes for invasive glioma cells [
14]. Most importantly, it has been shown that over 85% of glioma cells move into contact with blood vessels when injected into the brain [
15]. Furthermore, chemoattractants produced by endothelial cells can facilitate the recruitment of both invasive glioma cells and glioma stems cells to the perivascular space around blood vessels [
15,
16]. Invasion into the perivascular space disrupts the blood–brain barrier by separating the astrocyte end feet from the blood vessels [
17].
The other primary invasion route, white-matter tracts, is composed of myelinated axons. The corpus callosum is the largest source of tracts, through which the tumor cells successfully invade and reach several distant brain locations [
13]. Indeed, the invasive glioblastoma cells can exploit the connective utility of the corpus callosum or the anterior commissure, and can spread from one hemisphere to the other. Glioblastoma cells have been shown to enrich optic and pontine–white-matter structures, despite their distance from the primary site [
18,
19]. Indeed, these sites are well known to also harbor diffuse pediatric gliomas [
20]. Cell movement along white-matter tracts is partly mediated by several axonal guidance molecules, such as proteins belonging to the slit, semaphorin, and netrin families [
21].
Glioblastoma cells invade the brain parenchyma in small groups, and they interact with their environment in doing so [
22]. There are a variety of cell types in the brain microenvironment, such as astrocytes, reactive astrocytes, oligodendrocytes, glial progenitors, microglia, glial progenitors, neural progenitors, neurons, neural stem cells, and vascular endothelium. In addition to the autocrine signals expressed by the tumor cells, all of these cell types secrete diverse ligands and mitogens that induce invasiveness in glioblastomas. The interactions between these cell types and the resulting changes in the microenvironment have important roles in glioma progression and invasion [
23]. For example, higher invasion was observed when glioblastoma cells expressed EGFR and responded to EGF secretion from microglia [
24]. Besides the important tumor cell–stromal cell interactions, the physical environment that the tumor cells encounter constitutes a major component in their invasive behavior. For example, the tumor cells disseminate passively within the perivascular space by the flow of cerebrospinal fluid and actively move along the ventricular lining, using cell-surface receptors [
25,
26].
The invasion of tumor cells also depends on the crosstalk between the invading cells and cues from the microenvironment. Hypoxia is one of the microenvironmental features affecting the invasive properties of glioblastoma cells. While tumors are growing rapidly, the need for an oxygen supply increases. Lack of oxygen causes intravascular thrombosis, hemorrhage, and eventually necrosis of the tumor tissue. To evade the hypoxic environment and to reach oxygen, tumor cells migrate away from the hypoxic area, produce angiogenic factors to induce blood vessel formation, and adopt anaerobic glycolysis [
27].
3. Modes of Glioblastoma Invasion
Tumor cells may have different invasion mechanisms, depending on their association with other cells. They may migrate as individual cells or move together collectively. The extracellular matrix (ECM) composition, mechanical properties, such as stiffness or porosity, and topography are the factors that affect the migration and invasion properties of these cells [
28]. At a single-cell level, invasion may be categorized as mesenchymal or amoeboid. While the amoeboid mode of invasion is based on the propulsive movement without proteolytic ECM remodeling, the mesenchymal mode involves focal interactions with ECM and movement in a traction-dependent manner, due to cytoskeletal contractility [
29]. Due to the focal cell–ECM interactions, ECM-degrading proteolytic enzymes are recruited. These enzymes remodel ECM and generate a path for the invading cells [
30,
31].
Glioblastoma cells display unique invasion features, as they invade locally inside the brain, instead of generating distant organ metastasis. Glioblastoma cells generally invade as single cells and they exhibit the mesenchymal mode of invasion, or so-called saltatory migration [
32]. During invasion, tumor cells generate a strong adhesion force at the focal contacts on ECM by concentrating integrins. At the same time, they produce proteolytic enzymes such as matrix metalloproteinases (MMPs) to degrade the local ECM components by pulling and contracting the actin cytoskeleton to propel themselves toward the newly generated invasion path [
5] (
Figure 3).
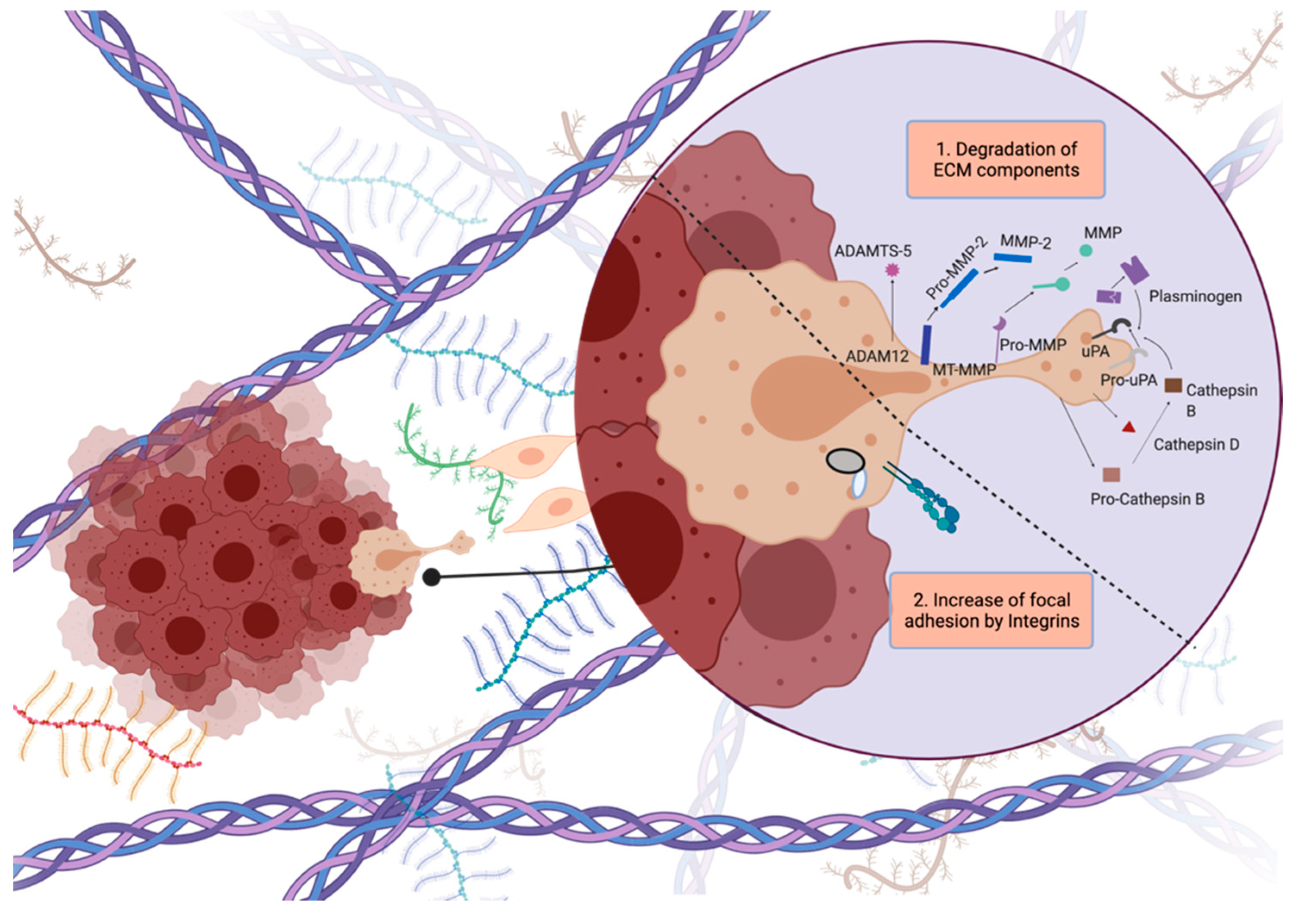
Figure 3. Steps of tumor cell invasion. Dynamic regulation of attachment–detachment cycles to break and generate contacts with ECM, and readjustment of cytoskeleton to generate protrusions are crucial for cell invasion. Figure generated at Biorender.com.
In 3D collagen gels, a “leader” glioblastoma cell reorganizes the collagen and creates a track for invasive glioblastoma cells to follow [
33]. These cells generate leading pseudopodia, which are short-lived, actin-rich membrane protrusions, and filopodia, which are long-lived, finger-like protrusions [
34]. Generation of these extensions that explore the environment is thought to be the mechanism in vivo to move along the white-matter tracts and blood vessels. Formation of these protrusions is followed by the formation of focal adhesions at the front edge and dissolution of these adhesions at the rear edge of the cells to detach from these adhesions [
35]. Dynamic regulation of attachment, protrusion, detachment, and readhesion is critical for controlling the cell movement. Strikingly, glioblastoma cells have been shown to generate an interconnected network in the brain and facilitate their infiltration using tumor microtubes, similar to their movement along other structures in the brain [
36].
Recent efforts have been directed towards understanding the heterogeneity within tumors, focusing on the differences between cores and edges of glioblastomas. For example, in a recent mouse model established with regionally derived glioblastoma cells, it was demonstrated that cells from the edge formed highly invasive tumors, and cells from the cores formed more confined tumors with therapy resistance. Additionally, it was uncovered that the core and edge cells exhibited a paracrine crosstalk through soluble factors to maintain their phenotypes [
37]. With the advanced imaging technologies, such as high resolution intravital microscopy of orthotopic mouse brain tumors, it has now been demonstrated that tumor cell dynamics vary at different locations [
38]. In the study by Alieva et al., various behaviors of tumor edges have been defined, such as slow but directed motility, or fast but less directed motility. Taken together, better understanding of the routes, patterns, and modes of glioblastoma invasion will provide clues for designing more effective surgical and therapeutic interventions.
4. Concluding Remarks
Given the aggressive characteristic of glioblastoma, the invasive glioblastoma cells are found already disseminated and embedded into the brain at the time of diagnosis. Despite tumor removal, these cells can recolonize and cause a recurrence of the tumor by resisting the conventional therapy approaches. Considering that conventional therapy approaches have a very limited effect on invasive glioma cells or cause adverse effects on healthy cells, understanding the mechanisms of glioblastoma cell invasiveness is important to develop effective therapeutic approaches. To this end, studies identifying druggable targets of glioblastoma invasion have a great potential to offer new approaches.
Therapeutics targeting invasion mechanisms would be useful to eradicate the population left behind after surgical removal and would make the tumors more manageable in the clinic, especially with complementary therapies that target other features of glioblastoma. However, considering that highly invasive cells have already disseminated to locations other than the primary tumor area and formed tumor seeds in secondary locations, preventing only the invasion may not eradicate these cells and may not change the patient’s survival considerably. To this end, anti-invasive therapies should be combined with other treatments. Offering such combinations would ultimately result in more manageable tumors and provide opportunities for better a prognosis for glioblastoma patients.
This entry is adapted from the peer-reviewed paper 10.3390/cancers14020443