1. Introduction
Forest trees provide valuable resources for economic, environmental, and industrial development. Indeed, these plants sustain human life directly or indirectly, which supply a wide range of goods and ecological services essential for survival and prosperity. The medicinal plants used in traditional medicine all over the world are a potentially rich source of therapeutic compounds. Population increase along with rapid technological advances are putting tremendous pressure on natural genetic resources, especially in developing countries, where such resources are rapidly declining, and more species face extinction [
1]. The more than 70 species in the pantropical genus
Pterocarpus (Fabaceae) are also faced with development pressure [
2]. The use of different plant parts in
Pterocarpus spp. to treat illnesses since ancient times has been well documented [
3,
4,
5,
6,
7,
8]. The species has received much attention in experimental studies because of the growing evidence of potential bioactivities. The increasing demand for wood has led to unsustainable harvesting from wild sources of three species of
Pterocarpus, namely
Pterocarpus marsupium,
P. santalinus, and
P. indicus, which are now recognized as threatened species [
9]. Besides the pharmaceutical value of
Pterocarpus species, the wood is also valuable for bridge and boat building, as well as small-scale construction materials, plywood, veneer, and specialty wood for musical instruments [
10].
The increase in public awareness of phytochemical-based drugs and the rapid growth of plant-based pharmacological industries have led to a greater demand for, and overexploitation of, natural flora. An increase in subsistence or non-commercial harvesting, as well as recent climatic changes have harmed many plant species, including
Pterocarpus spp. One possible biotechnical response to the decline of some species is micropropagation, which capitalizes on the totipotent nature of plant cells [
11]. In vitro propagation through tissue culture plays an important role in mass multiplication, plant improvement, plant breeding, regeneration of elite or superior clones, exchange of planting materials, secondary metabolites production, and germplasm conservation [
12,
13]. However, conventional propagation practice is time-consuming and labor-intensive and requires the availability of many plants for plantation or afforestation. Notwithstanding these challenges, plant cell, tissue, and organ culture techniques will become increasingly important in the cultivation of medicinal, as well as aromatic, plants by providing healthy and disease-free planting stock that can be widely utilized in commercial propagation and reforestation programs [
14,
15]. The information on actual genetic structure and the cryptic number of the differentiated genetic resources are valuable aids not only for developing in vitro regeneration protocols but also for the conservation of these medicinal plants. Many adulterants of
Pterocarpus plant materials are available in the market, and they affect the efficacy of the drug; in some cases, these adulterants might be toxic and can prove to be lethal. A DNA barcoding technique can be effectively utilized to characterize and authenticate
Pterocarpus and the detection of adulterants [
16]. This technique is an alternative for rapid and robust species identification of genuine plant materials for the herbal drug industry [
17].
P. marsupium propagates only by seed; the germination rate has been reported to be less than 30%, apparently because of the hard fruit coat coupled with poor viability and pod setting [
18]. The mature fruits are harvested from the trees in April and May or before they drop to the ground. Pathogenic infections of fallen fruit also affect the germination rate under natural conditions [
19]. Ahmad [
20] recommended freshly collected seeds as a good planting source for obtaining healthy plantlets. The oleo-resin exudates of this species contain several unique active constituents, including vijyayosin, pterosupin, marsupsin, and pterostilbene, all of which show a wide range of pharmacological activity [
21]. In addition, the National Medicinal Plant Board (NMPB) of India has estimated that the annual trade value of
P. marsupium is approximately 300–500 metric tons per year and that each mature tree (10–15 years) produces approximately 0.5–0.6 tons of dry heartwood, valued at US $ 1200–1500 for each mature tree in the international market. Due to the aforementioned increased interest in recent years in the pharmaceutical, as well as the economic value of
P. marsupium, the government of India has begun to encourage programs for large-scale cultivation and conservation of this species. The high demand for oleo-resin and wood, unsustainable harvesting practices, anthropogenic threats, and lack of regeneration, have together resulted in the rapid decline of natural populations. Furthermore, illegal harvesting of oleo-resin by damaging or wounding the wood can cause the trees to be susceptible to pests or diseases. Thus, uncontrolled extraction of oleo-resin could lead to adult mortality in combination with fragmentation and a low regeneration rate, threatening the persistence of the species.
Despite the enormous ethnobotanical value of P. marsupium, there is limited information on its genetic structure. Data on intraspecific relatedness is vital for selecting the best genotypes for plant breeding, effective population management, and conservation of germplasm. Given that genetic diversity allows populations to adapt to changing environments, the investigation of the genetic diversity of P. marsupium is not only important for species conservation, but also for the development and utilization of germplasm for improvement of this valuable but threatened medicinal plant. P. marsupium is a highly valuable medicinal plant but is a threatened species in India. Efforts for the conservation and propagation of this tree species will ultimately lead to the development of policies to make the species available for general use at a low cost. In view of these facts, it is of paramount importance to develop biotechnological techniques that ensure rapid propagation, multiplication, and authentication of the species for optimal germplasm conservation.
2. Phytochemistry and Therapeutic Values of P. marsupium
2.1. Active Constituents
A large number of important phytochemicals, such as glucosides, sesquiterpene and vijayoside (
Table 1) have been isolated from aqueous extract of heartwood of
P. marsupium [
30]. The extract of heartwood contains pterostilbene (
Figure 2A, pterosupin) (
Figure 2O, marsupsin (
Figure 2M), and liquiritigenin (
Figure 2N), (−)-epicatechin) (
Figure 2H) [
31,
32]. Bark extract contains several reputed phytochemicals such as 3-
o-methyl-D-glucose (
Figure 3C), n-hexadecanoic acid (
Figure 3D), 1,2-benzenedicarboxylic acid (
Figure 3E), tetradecanoic acid, (
Figure 3F), 9,12-octadecadienoic acid (Z,Z) (
Figure 3G), D-friedoolean-14-en-3-one (
Figure 3H), and lupeol (
Figure 2J) [
33]. At least eleven bioactive compounds, namely pterocarposide (
Figure 2P), 2,6-dihydroxyphenyl glucopyranoside (
Figure 2Q), pteroside (
Figure 3I), vijayoside (
Figure 3J), pterosupol, marsuposide, epicatechin, quercetin (
Figure 3M), vanillic acid (
Figure 3N), formononetin (
Figure 3O), and naringenin have been extracted from the heartwood of
P. marsupium [
21]. The most important bioactive compounds extracted from
P. marsupium are presented in
Table 1. Structures of bioactive compounds of
P. marsupium are shown in
Figure 2 and
Figure 3.
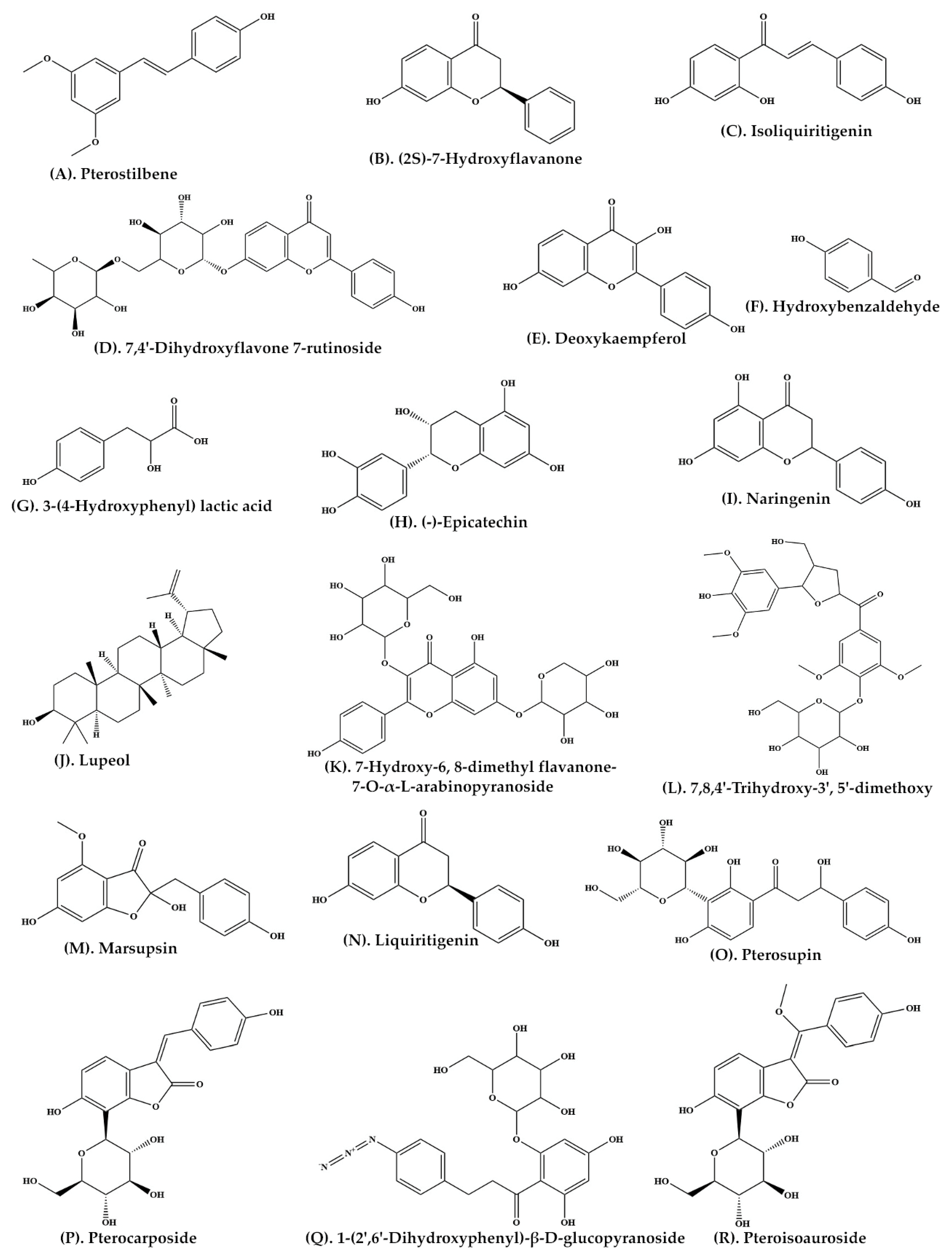
Figure 2. Molecular structure of bioactive compounds extracted from Pterocarpus marsupium Roxb.
Figure 3. Molecular structure of bioactive compounds extracted from Pterocarpus marsupium Roxb.
Table 1. Important bioactive compounds extracted from Pterocarpus marsupium (in chronological order).
Plant Parts |
Extract Preparation |
Technique * |
Bioactive Compound |
References |
Heartwood |
Ethyl acetate |
C-SG |
Pterostilbene (Figure 2A) (2S)-7-Hydroxyflavanone (Figure 2B) Isoliquiritigenin (Figure 2C) 7,4′-Dihydroxyflavone 7-rutinoside (Figure 2D) 5-Deoxykaempferol (Figure 2E) p-Hydroxybenzaldehyde (Figure 2F) 3-(4-Hydroxyphenyl) lactic acid (Figure 2G) |
[34] |
Bark |
Ethanolic extract |
C-SG |
(−)-Epicatechin (Figure 2H) |
[35] |
P. marsupium extract |
Ethyl acetate |
C-SG |
Naringenin (Figure 2I) Lupeol (Figure 2J) |
[36] |
Roots |
Ethanolic extract |
C-SG |
7-Hydroxy-6, 8-dimethyl flavanone-7-O-α-L-arabinopyranoside (Figure 2K) 7,8,4′-Trihydroxy-3′, 5′-dimethoxy flavanone-4′-O-β-D-glucopyranoside (Figure 2L) |
[37] |
Heartwood |
Ethyl acetate |
Thin Layer Chromatography |
Marsupsin (Figure 2M) Liquiritigenin (Figure 2N) |
[31] |
Heartwood |
Ethyl acetate |
C-SG |
Pterosupin (Figure 2O) |
[32] |
Heartwood |
Aqueous extract |
C-SG |
Pterocarposide (Figure 2P) |
[38] |
Heartwood |
Aqueous extract |
Coulman chromatography over Sephadex LH-20 |
1-(2′,6′-Dihydroxyphenyl)-β-D-glucopyranoside (Figure 2Q) |
[39] |
Heartwood |
Aqueous extract |
C-SG |
Pteroisoauroside (Figure 2R) Marsuposide (Figure 3A) Sesquiterpene (Figure 3B) |
[30] |
Leaves |
Methanolic extract |
UV-spectrophotometer |
Phenolics |
[40] |
Wood and bark |
Ethanolic extract |
GC-MS |
3-O-Methyl-d-glucose (Figure 3C) n-Hexadecanoic acid (Figure 3D) 1,2-Benzenedicarboxylic acid (Figure 3E) Tetradecanoic acid (Figure 3F) 9,12-Octadecadienoic acid (Z,Z) (Figure 3G) D-Friedoolean-14-en-3-one (Figure 3H) |
[33] |
Apical stem bark |
Methanolic extract |
Followed standard protocols |
Alkaloids Glycosides Flavonoids Terpenoids |
[41] |
Heartwood |
Ethanolic extract |
C-SG |
Pteroside (Figure 3I) Vijayoside (Figure 3J) C-β-D-Glucopyranosyl-2,6-dihydroxyl benzene (Figure 3K) |
[42] |
Heartwood |
Ethanolic extract |
C-SG and HPLC |
(+)-Dihydrorobinetin (Figure 3L) |
[43] |
Heartwood |
Methanolic extract |
LC-MS-MS |
Pterosupol Quercetin (Figure 3M) Vanillic acid (Figure 3N) Formononetin (Figure 3O) |
[21] |
Heartwood |
Methanolic extract |
HPLC and FTIR |
Liquiritigenin |
[44] |
2.2. Medicinal Properties
Ethno-medicine: Given the substantial evidence of its pharmacological properties,
P. marsupium has potential as an herbal drug yielding tree; indeed, it has been used to cure several diseases in the Indian traditional medicine system for many centuries [
30,
45]. The flowers of the tree are used in the treatment of fever, and the heartwood powder is useful in treating chest pain, body pain, and indigestion [
46]. Trivedi [
47] reported that a paste made from wood and seeds is useful in treating diabetic anemia. In addition, Yesodharan and Sujana [
48] have suggested that heartwood is useful in the treatment of body pain and diabetes. Interestingly, a cup made of the wood of
P. marsupium heartwood is used for drinking water to control blood sugar levels in “Ayurvedic” medicine [
49]. Aqueous infusions of the bark have also been used to treat diabetic patients since ancient times [
50]. The stem bark is used to treat urinary discharge and piles, and the resin-gum is applied externally in the treatment of leucorrhoea [
51]. The medicinal values of the active constituents or aqueous extracts of
P. marsupium are shown in
Table 2.
Table 2. Potential activities of some important bioactive compounds or aqueous extracts of Pterocarpus marsupium (in chronological order).
S.N. |
Extracts/Bioactive Compound |
Potential Activities |
References |
1 |
(−)-Epicatechin (Figure 2H) |
No effect on central nervous system Cardiac stimulant activity Anti-diabetic |
[35] |
2 |
Flavonoids |
Anti-hyperlipidemic |
[31] |
3 |
Phenolics |
Anti-hyperglycemic |
[32] |
4 |
Pterostilbene (Figure 2A) |
Cyclooxygenase-2 (COX-2) inhibition |
[52] |
5 |
Pterostilbene and 3,5-hydroxypterostilbene |
Induce apoptosis in tumor cells |
[53] |
6 |
5,7,2-4 tetrahydroxy isoflavone 6-6 glucoside |
Cardiotonic |
[45] |
7 |
Pterostilbene |
Anti-cancerous Anti-inflammatory Analgesic |
[54] |
8 |
Phenolics |
Anti-oxidant |
[40] |
9 |
Pterostilbene |
Anti-cancerous Anti-proliferative |
[55] |
10 |
Bark extract |
Anti-oxidant Analgesic |
[56] |
11 |
Extract of bark and wood |
Anti-diabetic Anti-hyperlipidemic |
[57] |
12 |
Extract of apical stem bark |
Anti-microbicidal |
[41] |
13 |
Phenolic-C-glycosides |
Anti-diabetic |
[42] |
14 |
Pterostilbene |
Novel telomerase inhibitor |
[58] |
15 |
Heartwood extract |
Dipeptidyl peptidase-4 (DPP-4) inhibition activity |
[59] |
16 |
Heartwood extract |
Anti-glycation Sorbitol accumulation Inhibition of aldose reductase |
[60] |
17 |
Pterostilbene |
Inhibition of platelet aggregation |
[61] |
18 |
Heartwood extract |
Reduction in body weight Anti-diabetic Anti-hyperlipidemic |
[62] |
19 |
(+)-Dihydrorobinetin (Figure 3L) |
Radical scavenging activity |
[43] |
20 |
Heartwood extract |
In vitro lipid lowering activity |
[21] |
21 |
Liquiritigenin (Figure 2N) |
Hypoglycemic activity |
[44] |
22 |
Pterostilbene |
Sun (UV rays) protective capacity |
[63] |
2.3. Micropropagation through Various Methods
In vitro propagation basically depends on the choice of appropriate explants (pieces of tissue used to initiate cultures) to serve as the preliminary experimental planting material. For multiple shoot bud induction (or bud breaking), the most frequently used explants are those that contain meristematic cells, such as cotyledonary nodes (CN), nodal segments (NS), immature zygotic embryo (IZE), hypocotyl segment (HS), shoot tips and root tip explants. The cell division potential is highest in these tissues, which apparently yield the much-needed growth-regulating substances, such as cytokinins and auxins [127]. In vitro propagation highlights the potential of morphogenic responses on various explants of P. marsupium under the regime of different plant hormone combinations. However, the morphogenic potential of explants of various organs varies and some do not grow at all. Explants derived from juvenile seedlings are frequently used for organogenesis under the regime of different plant growth regulators, as they are easily established in axenic culture and have a greater morphogenic potential than do mature explants obtained from donor mother plants [128–131]. The axenic seedlings of P. marsupium are a suitable source for obtaining axenic planting material (or explants) as they are aseptically grown from sterilized seeds. Multiple shoot bud induction during plant cell, tissue, and organ culture greatly depends on the type of plant growth regulators (PGR) applied, and their concentration, uptake, transport, and metabolism, and the endogenous hormone levels of explants [132,133]. Endogenous levels of cytokinins in explants are available in various forms, such as free bases, nucleotides, ribosides, O-glucosides, and N-glucosides [134]. Exogenously supplemented PGRs can modulate the action of enzymes that control the level of endogenous hormones and enzymes [135].
3. Conclusions and Future Prospects
The commercial productivity of tree plantations could be increased by reducing the genetic diversity of forest species and achieving greater homogeneity of tree phenotypes. Currently, many factors, such as increasing demand from pharmaceutical companies and timber-based industries and the overall decline of the global forest cover, as well as the impacts of climate change, have all motivated forestry decision-makers to raise the productivity of natural forests. There is also an increasing demand for aromatic and herbal drug yielding plants because natural products are seen to be non-toxic and have fewer side effects. Our comprehensive survey of the literature revealed that knowledge of the pharmacognosy, ethnobotany, and micropropagation of P. marsupium—a species of high value globally and in India—is rather limited and has only appeared in the past three decades. Plant biotechnology has opened new avenues for the generation of novel genetic variability, and techniques in this field now offer greater selection and are increasingly more precise and reproducible. Such techniques have broad applications in a number of important areas, for example, genetically modified food, feed, and fiber.
Micropropagation of P. marsupium can offer great advantages over traditional methods. Such advances can help researchers meet their goals in numerous specialties: plant breeding, plant biotechnology, germplasm conservation, rapid propagation of genetically modified plants, secondary metabolites biosynthesis, germplasm exchange, extensive collection within minimum space, supply of important planting material for wild population recovery, and molecular and ecological studies. Moreover, before developing any regeneration protocol, information on actual genetic variability and the cryptic number of the differentiated genetic resources are essential for both the genetic improvement of the species and its conservation. Before developing an effective method to maintain the genetic diversity of any targeted species, it must first be quantified. A promising method in regeneration programs is the use of DNA-based molecular markers.
Advances in DNA barcoding will help in the authentication of key forest species such as P. marsupium and may eventually lead to the formulation of legislation ensuring the public’s access to this plant at a reasonable cost. There are several substituted for P. marsupium wood on the market, and they can be less effective as medicines and, in some circumstances, fatal due to the toxicity of the substituted plant material as an open access, worldwide library of reference barcode sequences continues to be collected, DNA barcoding may be a viable answer to species authentication, allowing non-taxonomists to identify specimens. In vitro propagation and genetic diversity analysis of P. marsupium can be used effectively to select superior populations of the species for breeding programs aimed at improving productivity, wood quality, and chemical constituents, thereby helping to inform plans for conservation and sustainable use of this valuable plant species. More importantly, critical elements of an effective conservation strategies need to be discussed.