Rosemary (Rosmarinus officinalis [family Lamiaceae]), an herb of economic and gustatory repute, is employed in traditional medicines in many countries. Rosemary contains carnosic acid (CA) and carnosol (CS), abietane-type phenolic diterpenes, which account for most of its biological and pharmacological actions, although claims have also been made for contributions of another constituent, rosmarinic acid.
1. Uses of Rosemary and Rosemary Extract
Rosemary is an economically important plant species that is widely distributed due to its culinary, medicinal, and cosmetic uses
[1][2][3]. Both fresh and dried leaves of rosemary have been used for their characteristic aroma and flavor in many food dishes. Additionally, rosemary extracts have been employed as lipophilic antioxidants to preserve foods against oxidation from environmental stress
[1][2][3]. Because of these characteristics, the European Union, United States, and Japan have all approved rosemary extracts as food additives for food preservation
[4][5][6]. Rosemary contains two groups of potentially active compounds. One group is comprised of small molecular weight aromatic compounds, called “essential oils”, which rapidly evaporate to produce the characteristic smell and taste of rosemary. The other group is represented by the polyphenolic compounds including carnosic acid (CA) and carnosol (CS), which have been shown to manifest direct and indirect antioxidant actions
[7][8][9]. While our group has focused on the effects of CA, we also report here the work of others on CS.
2. CA as a Potential COVID-19 Therapeutic via Inhibition of NLRP3 Inflammasome, Other Inflammatory Pathways, and SARS-CoV-2 Infection
2.1. ARDS Mediated by NLRP3 Inflammasome
As schematically highlighted in
Figure 1, acute respiratory distress syndrome (ARDS) is initiated by SARS-CoV-2 infection. Viral replication can lead to TLR signaling and NF-κB activation (Signal 1 in
Figure 2)
[10][11]. However, this signal itself is not the direct cause of ARDS
[10][11]. It is the dysregulated, hyperinflammatory response to viral infection, mediated at least in part by NLRP3 inflammasome activation, which contributes to ARDS
[10][11]. As reviewed above, the extreme inflammatory response is triggered by activation of the NLRP3 inflammasome and other inflammatory pathways that induce release of proinflammatory cytokines such as IL-1β, IL-18, IL-6, and TNF-α
[12][13][14][15]. Thus, a potential countermeasure against the development of severe COVID-19 would be to prevent activation of NLRP3 and other inflammatory pathways
[12][13][14][15].
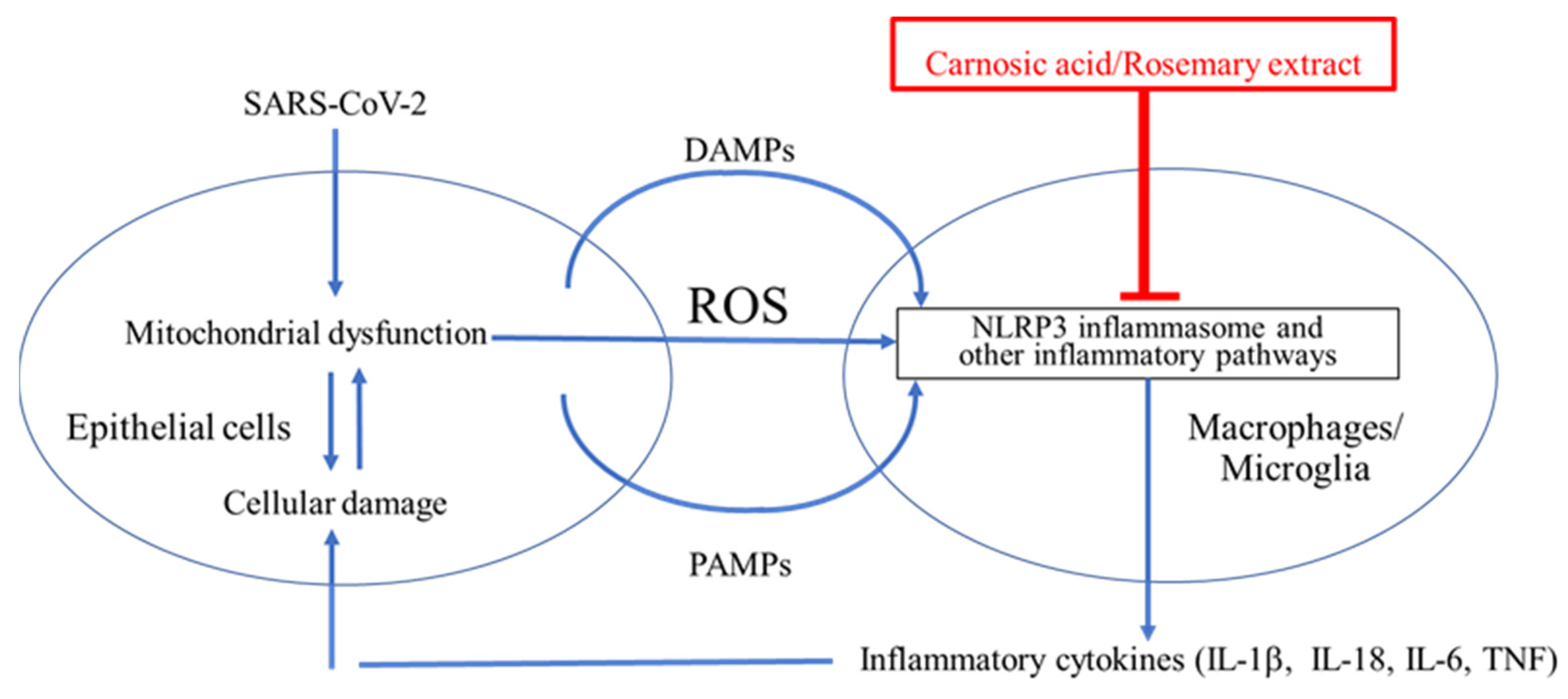
Figure 1. Cytokine storm contributes to ARDS in the lungs and possibly the brain of COVID-19 patients. SARS-CoV-2 binds to ACE2 and enters epithelial cells to induce endoplasmic reticulum (ER) stress due to overproduction of viral proteins
[16][17][18][19]. The damaged cells release damage-associated molecular patterns (DAMPs), pathogen-associated molecular patterns (PAMPs), and reactive oxygen species (ROS)
[20][21][22]. ROS, DAMPs, and PAMPs activate the NLRP3 inflammasome in surrounding macrophages in the lung and potentially in microglia in the brain
[23][24]. This activation is critical for the release of inflammatory cytokines such as IL-1β from macrophages and microglia
[23][24]. These cytokines enhance damage of epithelia and other cell types and may contribute to cytokine storm and the severe lung disease of ARDS
[10][11]. The NLRP3 inflammasome is thus a plausible drug target for treating ARDS
[23][24].
2.2. CA as a Potential Treatment for COVID-19 by Suppressing Infection and Cytokine Production
Recently, McCord et al. reported that PB125, a CA-based therapeutic agent, was potentially useful in the treatment of respiratory viral diseases, including COVID-19, in part by inhibiting cytokine storm
[25][26][27]. They observed a marked downregulation of genes encoding inflammatory cytokines including IL-1β, IL-6, TNF-α, cell adhesion molecules (ICAM-1, VCAM-1, and E-selectin), and a group of interferon-γ-induced genes, suggesting that cytokine storm might be suppressed by CA
[25][26][27]. McCord and colleagues further reported that PB125 downregulates ACE2 and TMPRSS2 mRNA expression in human liver-derived HepG2 cells, consistent with the notion that CA might inhibit viral entry, which ACE2 and TMPRSS2 proteins mediate
[25][26][27]. These results suggest that the use of rosemary extract or CA might represent a possible therapeutic against acute ARDS in the lung and more protracted cytokine production
[25][26][27]. Therefore, clinical testing of rosemary extract or CA for either acute COVID-19 lung disease or chronic (so-called long) COVID-19 might be considered. The rationale for such treatment is discussed further below. A caveat with these findings, however, is the fact that IL-1 or IL-6 inhibitors have been reported to suppress SARS-CoV-2 neutralizing antibodies in patients with COVID-19
[28]. Hence, the timing of any therapy aimed at suppressing these cytokines will have to be judiciously monitored in order not to interfere with anti-SARS-CoV-2 antibody therapies
[25].
2.3. CA as a Potential Anti-Infectious Agent against SARS-CoV-2 by Inhibiting Binding to ACE2
Recently, two of us (C-k.O. and S.A.L.) found that CA can inhibit infection with SARS-CoV-2, approaching 90% efficacy, as assessed in a pseudovirus entry assay (Figure 2A). Mechanistically, CA, after conversion to the quinone form, is likely to S-alkylate critical thiol residue(s) on ACE2, the cell surface receptor for SARS-CoV-2 (Figure 2B). This reaction would then block the interaction of ACE2 with the Spike protein of SARS-CoV-2, and thus abrogate viral entry. Further work on reactions of these critical thiol groups in preventing SARS-CoV-2 infection is forthcoming.
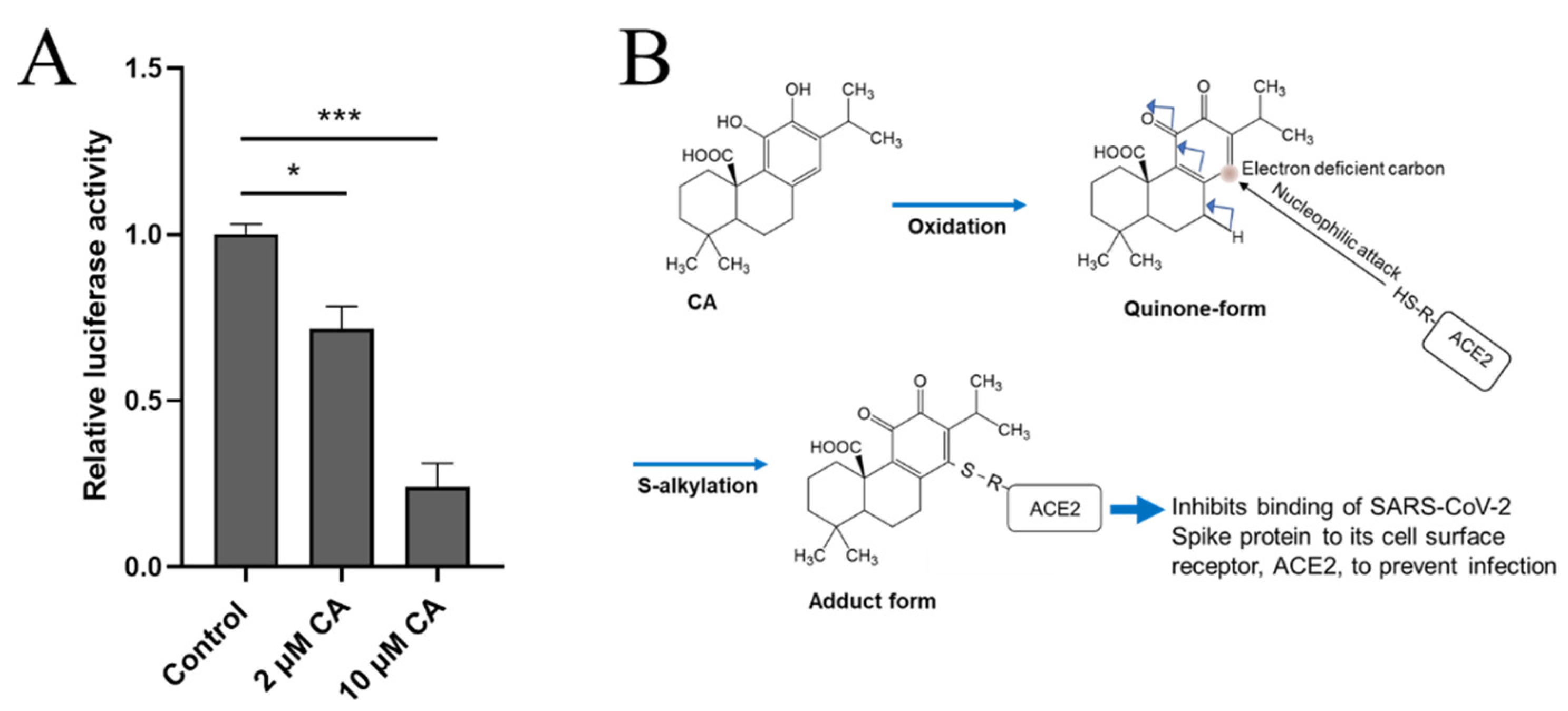
Figure 2. CA inhibits SARS-CoV-2 infection. (
A) Inhibition of SARS-CoV-2 infection by CA in pseudovirus entry assay. HeLa cells stably-expressing ACE2 (HeLa-ACE2) were incubated with SARS-CoV-2 Spike (D614) pseudovirus particles in the presence and absence of the indicated concentration of CA, as described previously
[29]. After 48 h, viral transduction efficiency was monitored by luciferase activity. Data are mean ± S.E.M., *
p < 0.05, ***
p < 0.001 by ANOVA with Tukey’s multiple comparisons post hoc test;
n = 3 biological replicates. (
B) Postulated mechanism of action of CA in preventing Spike protein of SARS-CoV-2 from binding to ACE2. The reaction mechanism involves nucleophilic attack of cysteine thiol of ACE2 on the electron deficient carbon of the quinone formed by oxidation of CA.
3. Proposal for Testing Rosemary Extract or CA against SARS-CoV-2 Infection, Long- COVID, and Neurodegenerative Disorders
As we write this review, we are still in the throes of the COVID-19 pandemic with new variants frequently still appearing. While vaccinations and masking have abated the severity of infection in many cases, the unvaccinated are frequently infected and break-through infections of fully vaccinated and boosted individuals can also still occur. Thus, an effective pill to treat COVID-19 infection is still urgently needed. In addition to acute infection, long-COVID, presumably with direct or indirect effects on the brain, is also a persistent and prevalent problem. The neuropsychological symptoms of long-COVID may well stem from innate inflammatory pathways, at least in part attributable to activation of the NLRP3 inflammasome. Moreover, similar inflammatory pathways have been shown to contribute to the pathogenesis of neurodegenerative disorders including Alzheimer's disease (AD) and Parkinson's disease (PD)/Lewy body dementia (LBD). Here, we propose that CA from Rosmarinus officinalis, an innocuous pro-electrophilic compound that is converted to an active electrophile, can be used for the treatment of acute and chronic COVID-19 manifestations and for chronic neurodegenerative disorders based on the following:
-
CA is a pro-electrophilic drug (PED) and thus is much safer than electrophilic NRF2 activators because it is activated only at the site of oxidation and inflammation by ROS, which it then combats
[30][31][32][33][34].
-
CA can potently suppress cytokine storm
[25][26][27].
-
CA passes through the blood–brain barrier (BBB) with good bioavailability after oral administration
[35].
-
CA inhibits activated microglia, in part, via inhibition of the NLRP3 inflammasome
[36].
-
Rosemary (containing CA and CS) is recognized as a food additive in the U.S., EU, and Japan
[4][5][6].
-
Rosemary (containing CA and CS) is already used clinically as a therapeutic against rheumatoid arthritis in Germany and is on the FDA “generally regarded as safe” (GRAS) list in the U.S.
[37][38]. Moreover, CA manifests very few side effects at very high concentrations in two-species toxicity testing.
-
CA and CS have been shown to manifest neuroprotective effects in vivo in multiple models of AD and PD
[39][40][41][42][43].
4. Conclusions
Carnosic acid (CA), a diterpene found in the herb rosemary, is a safe pro-electrophilic drug (PED) that activates the KEAP1/NRF2 transcriptional pathway, leading to the sustained induction of phase 2 antioxidant and anti-inflammatory enzymes. CA exerts neuroprotective and anti-inflammatory effects and has been proposed to be a potential therapeutic against chronic neurodegenerative disorders, such as AD and PD, and acute and chronic effects of infections such as SARS-CoV-2. Here, we review the evidence and mechanistic considerations supporting the future testing of rosemary extract or CA against these maladies in human clinical trials. The use of rosemary extract in general and CA in particular as an NRF2 activator and anti-inflammatory has gained wide attention due to the virtual absence of side effects and its relatively low economic cost compared to other strategies. The health-promoting effects of CA have been demonstrated in multiple animal models against chronic neurodegenerative disorders. Moreover, inhibition of the NLRP3 inflammasome by CA represents an important molecular target in limiting IL-1β pro-inflammatory cytokine production. This review also highlights the role of the NLRP3 inflammasome in neurological diseases such as AD and PD/LBD, and in the pathogenesis of COVID-19 including so-called ‘long-COVID’ symptoms. CA appears to inhibit the manifestations of SARS-CoV-2 infection by two mechanisms, one at the point of viral entry, possibly through covalent binding to the ACE2 receptor protein, and the other by ameliorating cytokine storm through the inhibition of NLRP3 inflammasome activation. Thus, CA represents a potential therapeutic for COVID-19 as well as for neurodegenerative disorders like AD and PD/LBD.
This entry is adapted from the peer-reviewed paper 10.3390/antiox11010124