Piperine (PIP) is an active alkaloid of black and long peppers. An increasing amount of evidence is suggesting that PIP and its metabolite’s could be a potential therapeutic to intervene different disease conditions including chronic inflammation, cardiac and hepatic diseases, neurodegenerative diseases, and cancer. In addition, the omnipresence of PIP in food and beverages made this compound an important investigational material. It has now become essential to understand PIP pharmacology and toxicology to determine its merits and demerits, especially its effect on the central nervous system (CNS). Although several earlier reports documented that PIP has poor pharmacokinetic properties, such as absorption, bioavailability, and blood–brain barrier permeability. However, its interaction with metabolic enzyme cytochrome P450 superfamily and competitive hydrophobic interaction at Monoamine oxide B (MAO-B) active site have made PIP both a xenobiotics bioenhancer and a potential MAO-B inhibitor. Moreover, recent advancements in pharmaceutical technology have overcome several of PIP’s limitations, including bioavailability and blood–brain barrier permeability, even at low doses. Contrarily, the structure activity relationship (SAR) study of PIP suggesting that its several metabolites are reactive and plausibly responsible for acute toxicity or have pharmacological potentiality.
1. Introduction
Piperine (PIP), an alkaloid, omnipresent in foods/beverages, is currently one of the compounds of interest for showing numerous pharmacological benefits. PIP is a yellow crystalline solid compound mainly isolated from black pepper (Piper nigrum), but also found in other Piper species (Table 1). This compound is bio-synthesized from L-lysine and cinnamoyl-CoA precursor as a secondary metabolite (Figure 1).
After a series of reactions, the bioactive PIP is yielded from piperonyl-CoA [
1]. Although earlier studies re-ported that PIP has poor bioavailability [
2] owing to its lipophilic nature, it can inhibit metabolic enzymes like CYP3A4 and drug transporter P-glycoprotein and modulate xenobiotics metabolism in human liver [
3]. In the process, PIP inhibits the metabolism and efflux of xenobiotics, making PIP a potential bioavailability enhancer of drugs and nutraceuticals.
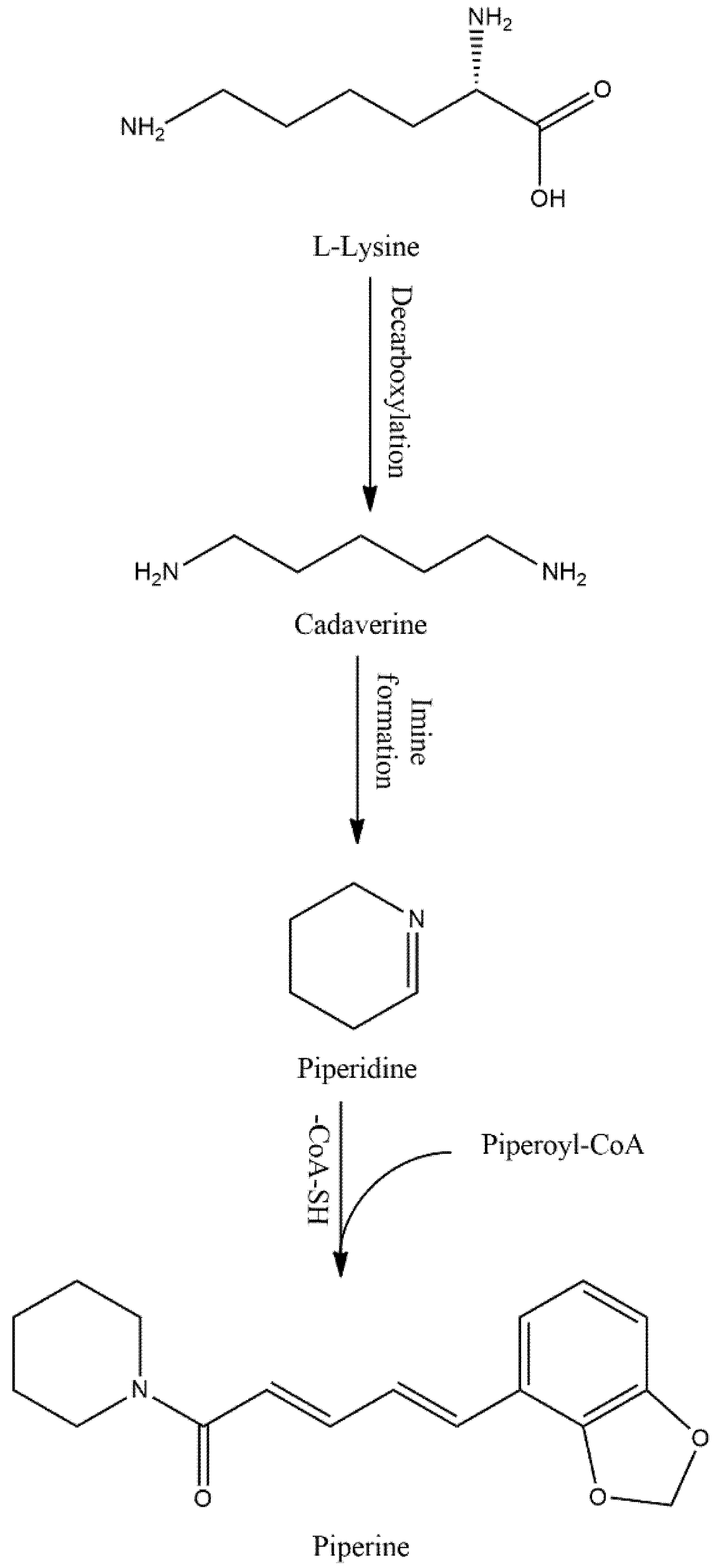
Figure 1. Possible biosynthesis pathway of PIP.
Table 1. Estimated amounts of PIP in different plant parts.
Sources |
Plant Parts |
Estimated Amount (%) |
Piper nigrum [2] |
Fruit |
1.7–7.4 |
Piper longum [3] |
Spike and root |
5.9 |
Fruit |
0.03 |
Piper chaba [3,4] |
Fruit |
0.95–1.32 |
Piper guineense [5] |
Fruit |
0.23–1.1 |
Piper sarmentosum [6] |
Root |
0.20 |
Stem |
1.59 |
Leaf |
0.104 |
Fruit |
2.75 |
2. PIP in Neurological and Psychiatric Diseases
2.1. AD
Oxidative stress plays a vital role in the pathogenesis of AD and subsequent cognitive impairment. A recent study used intracerebroventricular (ICV) injection of streptozotocin (STZ) for AD modeling in rats by inducing severe oxidative stress and impairing hippocampal neurotransmission [
37]. In their model, a low dose of PIP (2.5 mg/kg/day for 4 weeks) improved the memory task and long-term potentiation in animals. Interestingly, chronic PIP administration resulted in more efficient synaptic plasticity than donepezil. Chronic treatment of PIP for 23 days also improved locomotor activity and neurotransmission by reducing oxidative-nitrosative stress in cerebrospinal fluid and hippocampus and enhanced cholinergic function in ICV-STZ infusion-induced sporadic AD mouse model [
38,
39]. Moreover, PIP treatment inhibits glutamate release from hippocampal nerve endings by reducing Ca
2+ current in rats [
40], which could increase paired-pulse facilitation and, subsequently, increase short-term synaptic plasticity. Acetylcholine (ACh) receptor is abundantly expressed in the hippocampus and regulates plasticity and cognitive function. PIP and its derivatives have shown potential inhibition of ACh esterase (AChE) [
41], an enzyme that catalyzes and breaks ACh into acetate and choline. Although the molecular basis of ICV-STZ infusion-induced memory deficits is unknown, STZ infusion causes a sharp increase in amyloid-β (Aβ), synapsin, and tau-phosphorylation [
42]. Therefore, it could be presumed that PIP treatment might modulate misfolded Aβ and tau hyperphosphorylations. A study showed PIP treatment not only benefits cholinergic functions, but also benefits from Aβ and tau pathogenesis by inhibiting β-secretase [
43,
44].
Further, as we discussed earlier, PIP is a potential bioenhancer of drugs and/or nutraceuticals. The combination of existing and/or emerging therapeutics with PIP has become popular practice. With such intentions, Head et al. [
45] tested a medical cocktail containing PIP (epigallocatechingallate 36.3% by wt., PIP 3.0% by wt., N-acetyl-l-cysteine 15.3% by wt., curcumin 36.3% by wt., and R-lipoic acid 9.1% by wt.) in aged dogs in an AD model. After 3 months of treatment, the dogs showed improved spatial attention and reduced cognitive impairment compared with non-treated age matching dogs, though brain and CSF Aβ remains unchanged. As that study observed neither Aβ plaque accumulation nor soluble and/or insoluble Aβ1-40 and Aβ1-42, it could draw few conclusions. For example, the presence of PIP in the cocktail not only improves other molecules’ pharmacokinetic property, but also its potential antioxidant property ameliorating cognitive impairment independently of Aβ or PIP could be inhibiting β-secretase [
43,
44] to prevent pathogenic Aβ fragmentation and accumulation.
One major limitation of PIP treatment is its poor bioavailability, for which PIP requires a high dose to reach maximum efficiency. In addition to overcoming this limitation, nano-formulation or formulations containing PIP nanoparticle are being developed. Intranasal treatment of PIP-loaded chitosan nanoparticles showed 20-fold better pharmacokinetics than oral treatment in male AD rats [
46]. PIP solid lipid nano-formulation also improved blood–brain barrier permeability and ameliorated mobility in AD animals by reducing acetylcholinesterase activity and Aβ plaque and tangles [
47]. The PIP loading into nonionic surfactant-based ME formulations could increase its low-density lipoprotein receptor affection and might increase blood–brain barrier permeability [
48]. This might enhance its potentiality in AD treatment. However, the surfactants’ concentration must be carefully adjusted, as a high concentration might cause nephrotoxicity [
49].
2.2. PD
Dopamine depletion is a major biomarker of PD pathogenesis. MAO-B is an important catalyst of dopamine synthesis, and the inhibition of MAO-B might deplete dopamine synthesis. MAO-B is a flavin adenine dinucleotide (FAD) containing enzyme, located in the mitochondrial outer membrane [
50]. We discussed above that PIP and its metabolites are a potential inhibitor of both MAO-A and -B. The presence of the pentacyclic ring in the structure could reduce the MAO-B inhibition potential of PIP, while removal of nitrogen from the pyrrolidine ring might increase MAO-B inhibition selectively in the PD model [
51]. Moreover, PIP treatment has diminished lipid peroxidation and increased glutathione in 6-hydroxydopamine (6-OHDA) lesioned rat’s striatum [
52]. PIP treatment also induced atuophagolysosome via Akt/mTOR pathway activation and reduced neuronal degeneration in substantia nigra in rotenone-injected PD mice [
53]. A PIP-mediated increase in protein phosphatase 2A (PP2A) ameliorated mitochondrial injury, mitochondrial membrane permeability transition pore (mPTP) impairment, and oxidative stress [
53,
54]. In addition, SAR of PIP showed substitution of the aromatic group with a heterocycle and piperidine with diethylamine and ethylamine could enhance its pharmacokinetics and blood–brain barrier permeability. Meanwhile, such a modification also improved PIP derivatives’ efficiency in Nrf2/Keap1 pathway activation, thus providing neuroprotection in the PD model [
55]. PIP gavaging (25–100 mg/kg b.w.) could restore purinergic receptor P2X ligand-gated ion channel 4 (P2RX4) activity and promote autophagic flux in the α-synuclein overexpressed mouse PD model [
56]. Autophagy plays an important role in PD, while α-synuclein impairs autophagic flux by inhibiting syntaxin 17 (STX17), which promotes autophagolysosome membrane fusion by P2RX4 inactivation. PIP treatment re-activated P2RX4, promoted autophagic flux, improved neuronal cell viability, and ameliorated motor impairment. PIP (10–40 mg/kg) treatment for 40 days in a carotid artery occlusion model of PD dementia showed reduced expression of mammalian target of rapamycin (mTOR) regulator miR-99a-5p and pro-inflammatory factors (IL-6 and TNF-α), as well as slowing PD dementia pathology [
57].
2.3. Huntington’s Disease
HD is a complex neurodegenerative disease characterized by progressive motor dysfunction and mental abnormality owing to progressive loss of striatal neurons. PIP activity in an HD model has not been extensively researched. A recent study used 3-Nitropropionic acid (3-NP), a mycotoxin, to induce an HD-like model in rats and test PIP potentiality. PIP treatment restored ATP production and ameliorated striatal degeneration in basal ganglia and motor function in HD rats [
58].
2.4. Epilepsy
PIP has been studied by several research groups for the therapeutic potential in epilepsy, a neurological disease characterized by frequent seizures. PIP received special attention in anti-epileptic treatment for its potential MAO inhibitory and neuroprotective activity. In temporal lobe epilepsy or post status epilepticus (pSE) animal model, PIP treatment (25 mg/kg for 10 days) restored the serotonin level and modulated MAO and γ-amino butyric acid (GABA)ergic pathway [
59]. It is also reported that PIP treatment increased GABA, glycine, and taurine, and reduced nitrite in the sera and brain of a pilocarpine-induced epileptic mouse model [
60]. In a maximal electroshock (MES)-induced seizure model of epilepsy, PIP treatment (10 mg/kg) reduced the morbidity by negatively regulating the Na
+ channel, which delayed the onset of tonic clonic seizures [
61], indicating that PIP could modulate the Na
+ channel to reduce tonic clonic convulsion and related morbidity in epilepsy. For instance, blocking of transient receptor potential cation channel subfamily V member 1 (TRPV1) by a selective antagonist suppressed PIP-mediated anti-convulsant activity [
62], indicating that TRPV1 might also be involved in the PIP mechanism. Unlike capsaicin, which forms a hydrogen bond at T551 and E571 ligand-binding sites of TRPV1, PIP might directly interact with the pore-forming S6 segment to induce channel opening [
63]. Besides, the use of PIP as a sub-therapeutic agent or therapy enhancer revealed another chapter for PIP importance. Co-treatment of PIP and carbamazepine in epilepsy patients showed a significant increase in carbamazepine bioavailability, absorption, and AUC and reduced elimination half-life [
15,
64].
2.5. Other Neurological Diseases
PIP has also gained widespread attention in a few other neurological disorders. For example, a recent study showed that PIP interacts with r(CGG)
exp RNA motifs and that this interaction improves r(CGC)
exp associated splicing defects and RNA translation in fragile X-associated tremor/ataxia syndrome (FXTAS) [
65]. FXTAS is a neurological disease where the trinucleotide CGG repeats more than 200 times and results in clinical features like multisystemic atrophy, intention tremor, cerebellar and gait ataxia, and dementia. The interaction of PIP with r(CGG)
exp in an FXTAS model indicates its therapeutic importance in FXTAS treatment. Moreover, PIP could modulate the serotonin (5-HT) level in the brain and function as an anti-depressant and could synergize antidepressant activity of tetralin (5-HT
1A agonist) and anpirtoline (5-HT
1B agonist) [
66]. Interestingly, PIP-mediated anti-depressant activity involves BDNF/TrkB signaling cascade as well, and chronic treatment of PIP could increase BDNF protein concentration in the hippocampus and cortex of the mouse model of depression [
67]. Moreover, a PIP derivative, SCT-66, has been reported to modulate GABA
A and TRPV1 receptors more effectively than PIP [
68]. SCT-66 has also been reported to have much better anxiolytic than PIP, without changing body temperature.
Neuroinflammation is the most common pathophysiological feature of many neurodegenerative diseases including AD and PD. ROS production and glial cells’ activation is the key to the neuroinflammatory response. A series of pro-inflammatory cytokines including tumor necrosis factor α (TNF-α), interleukin 1β (IL-1β), and interleukin 6 (IL-6) are released and exaggerate the neurodegeneration. Among the other pathways, nuclear factor erythroid 2 related factor 2 (Nrf2) and heme oxygenase-1 (HO-1) are critical regulator of endogenous oxidative stress (
Figure 4). PIP could activate Nrf2 signaling and downregulate NF-κB activation [
69], a nuclear factor that could translate an inflammatory cytokine like IL-1β. PIP treatment (10 mg/kg, once daily) for 15 days has been shown to ameliorate behavioral deficits and pro-inflammatory cytokines (IL-1β, IL-6, and TNF-α) by reducing infarct volume and NF-κB activation in a middle cerebral artery occlusion (MCAO) rat model [
70].
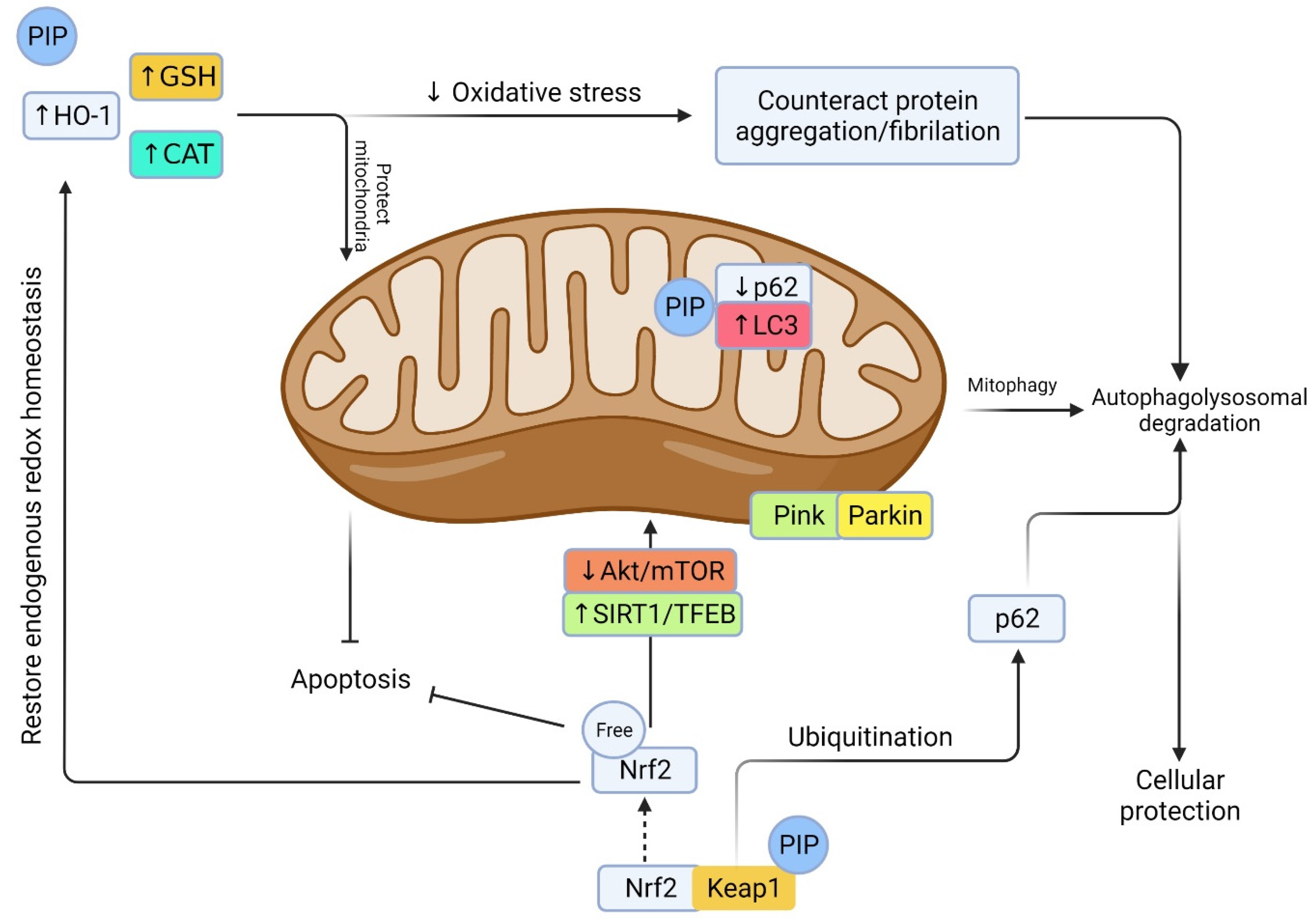
Figure 4. Prospective machinery of PIP in oxidative stress-induced neurodegenerative events. Oxidative stress arises from a decrease in endogenous antioxidant defense including HO-1, GSH, CAT, and Nrf2. This leads to a cascade of events including protein oxidation/glycation/mitochondrial damage, protein aggregation, and impaired autophagy/lysosome vacuoles. PIP is a potential antioxidant that could induce oxidative stress via promoting antioxidant defense, reducing ROS, and preserving mitochondrial integrity. On the other hand, Keap1 degradation and Nrf2 activation by PIP could counteract oxidative stress, reduce mitochondrial damage, promote autophagy by degrading/ubiquitination of p62/sequesterosome, promote autophagic flux by converting microtubule-associated protein 1A/1B-light chain 3 (LC3), promote mitophagy and mitobiogenesis via distinct pathway, and rescue from cellular apoptosis and degeneration [
74].
Depression is one of the major psychiatric disorders featured by fatigue, pain, cognitive dysfunction, depressed mood, and/sleep disturbances. PIP (5–20 mg/kg) treatment for 4 weeks showed anti-depressant activities in mildly stressed rats, plausibly by enhancing the serotonin level at the cerebral cortex and limbic area [
71]. Besides, chronic administration of PIP and its derivative antiepilepsirine (10–20 mg/kg) for 2 weeks significantly increased the dopamine and serotonin level in the hypothalamus and hippocampus, resulting in anti-depressant-like activities [
72]. Another study showed that pre-treatment of PIP (5–20 mg/kg, i.p.) in a stress-induced anxiety model significantly reduced the nitrite level and suppressed anxiety-like activities [
73].
3. Therapeutic Index and Future Perspective
Indeed, PIP is an important natural compound that has multiple health benefits. Yet, there are a few limitations halting its wide application. Firstly, the hydrophobicity, which caused its poor absorption and bioavailability. Another major concern of PIP is toxicity due to the presence of the MDP group in its structure [
11], which is common in carcinogenic compounds like safrole. Though the carcinogenicity of PIP has not been confirmed yet, treatment of 2 mg/kg on 3 days of a week for 3 months caused tumors in mice [
75], showed cytotoxicity to cultured neurons of rat brain [
76], and showed the formation of benzo[a]pyrene-DNA adducts that caused lung fibroblast cytotoxicity [
77]. Consistent oral administration of PIP at 1.12–4.5 mg/kg body weight for 5 days in mice reduced leukocytes and myogenic response of B lymphocytes and increased neutrophils [
78]; however, the lowest dose (1.12 mg/kg) had no immunotoxicity. Moreover, no adverse effects level (NOAEL) of PIP have been observed at 5 mg/kg/day consumption in humans [
79], which indicates that PIP consumption through different foods has no toxic effect other than irritation if a higher amount than normal is consumed. Besides, subacute toxicity testing of PIP oral and intravenous dosing showed that up to 100 mg/kg of oral dosing is nontoxic, whereas intravenous dosing has an LD
50 of 15.1 mg/kg body weight of adult mice [
10]. As PIP is mainly administered via the oral route, these data suggest that PIP is nontoxic and safe, and might cause irritation if overdosed.
Apart from a few adverse effects reported, so far, for overdosing of PIP, the main concern is high lipophilicity. Lipophilicity is an important physicochemical property of a compound that has parabolic relationship with in vivo brain penetration. High lipophilic compounds often experience low blood–brain barrier access owing to increased non-specific plasma protein bindings and vulnerability to P450 metabolism that led to rapid clearance of compounds. Because of this high lipophilic property, a low concentration of PIP is observed in the brain, limiting its neuroprotective effect at a low dose. Several recent studies have addressed this issue and resolved it on their own. For example, tween-modified monoolein cubosomes (T-cubs) loaded with PIP showed higher efficacy over free drug without affecting PIP’s cognitive function restoration effect in an AD model [
80]. Microemulsification of PIP also improved its bioavailability and blood–brain barrier permeability significantly [
48]. Similarly, intranasal delivery of PIP-loaded chitosan nanoparticles [
46] or PIP-SLNs’ formulation via emulsification coated with PS80 [
47] showed that a lower dose of PIP could be effective as well. However, as PIP is a potential bioenhancer, it could be administered with other drugs or herbs to potentiate their therapeutic efficacy in neurodegenerative diseases, like with curcumin, resveratrol, quercetin, carbamazepine, metronidazole, silybin, oxytetracycline, and/or phenytoin [
10,
81].
PIP is a potential antioxidant compound as well as an important therapeutic candidate for neurodegenerative diseases. In this current review, although we discussed beneficial sites of PIP, PIP is also reported for toxicity at a high dose. Extensive structural and functional chemistry-based research is needed to overcome this limitation. Besides, few studies until now have reported on PIP pharmacology on neurodegenerative diseases, especially of human trials. Thus, the answers to a few questions remain unclear, such as does PIP have role over misfolded protein clearance (Aβ, tau or αS)? Is PIP treatment protecting BBB integrity from environmental toxin-induced condition? A lack of studies on PIP in neurodegenerative disease-like models have limited our study from explaining more details about the therapeutic application of PIP. However, the strong point of this review is that we now know how to enhance PIP bioavailability, and that PIP co-treatment enhances other therapeutics’ bioavailability.
This entry is adapted from the peer-reviewed paper 10.3390/biomedicines10010154