The conventional methodologies for the design of flexible pavements are not adequate in providing solutions that meet the diverse sustainability challenges. Therefore, developing new methodologies and frameworks for the design of flexible pavement has become a priority for most highway agencies. On the other hand, there is no sound sustainable flexible pavement framework at the design phase that considers the key engineering performance, environmental impact, and economic benefits of sustainability metrics. Hence, premature failure of flexible pavements has become a common problem leading to a growing demand for sustainable pavement. Pavement engineers need to have access to tools that permit them to design flexible pavements capable of providing sustainable solutions under various complex scenarios and uncertainties.
1. Introduction
Flexible pavements are one of the vital gears of transportation systems [
1] that sustain socio-economic growth for a nation [
2]. However, their construction and preservation require large capital investment [
3]. Thus, ensuring sustainable flexible pavements [
4] has become a primary focus of flexible pavement management systems and highway agencies [
5]. Fundamentally, pavement sustainability is a complex and broad concept [
6] that can be evaluated by analyzing three pillars: the environmental, performance, and economic sustainability of the system [
2]. Environmental sustainability evaluation tools were developed to quantify the environmental impacts of pavement [
7]; economic sustainability tools were designed to evaluate the monetary influence of possible modifications in design, construction, and materials; and resilience evaluation approaches were developed to evaluate the sustainability levels of engineering performance of the system holistically [
8]. Application of green technology [
9], recycling and reuse of materials, and utilization of locally available materials contribute to sustainable pavement [
8]. Generally, pavement sustainability is a non-tradeoff balance between engineering performances, the least possible environmental impacts, and economic benefits aspect to pavement assets [
10]. Sustainability-driven systems need to be environmentally helpful as well as economical [
11]. Sustainability of flexible pavements throughout the design lifetime, under the rare but damaging severe environmental events, needs to be addressed at the design stage based on resilience, LCA, and LCCA.
The resilience triangle [
12], a hybrid bottom-up/top-down adaptation [
13], and Bayesian network [
14] approaches were used to assess the infrastructure performance and quantify the resilience of the system during normal and extreme event conditions. LCA framework has been established to calculate energy consumption and greenhouse gases (GHG) emission during material production [
15]. LCA and Mechanistic-Empirical Pavement Design Guide (MEPDG) integrated framework has been developed to support an inventory analysis [
1]. The MEPDG helps in performing LCCA [
1]. Uncertainties associated with model parameters, measurement variabilities, traffic growth, and the changes in the pavement IRI with time were evaluated using the probabilistic LCCA approach [
16]. LCCA model was established to quantify the impact of pavement preservation on the cost of agency and vehicle operation [
17]. Fundamentally, LCCA is a decision-support tool applied by highway agencies to compare the overall cost of alternative projects [
18,
19,
20].
The available sustainability technology is very limited to satisfactorily assess the environmental and economic impacts and engineering performance of asphalt mixtures and waste, recycled, and reused materials [
10]. The resilience triangle approach is not properly structured and complex to quantify the resilience metrics of the system [
12]. Further, there is significant variation from the available resilience prediction models in terms of the magnitude of resilience metrics [
21]. The LCA framework uses limited information in the early design phase of roads to evaluate their environmental impacts [
22] because it is difficult to acquire the data necessary to carry out LCA leading to the final design. Lack of transparency and homogeneity of the data used by several researchers for the design and LCA features of pavement has also been the barrier for a harmonized application of LCA [
23]. In other words, the choice of LCA methodology varies with the type of study [
24]. Thus, by adjusting these inconsistencies, an environmentally friendly asphalt technology could be recognized [
23]. Frequently, experts get challenged by substantial uncertainties in estimating the short-term and long-term costs [
25]. A framework needs to be developed to quantify the sustainability of flexible pavements and their design alternatives by analyzing the environmental and economic impacts of the pavements [
7].
The term sustainability started to get due attention around 1972 [
26] and applies to every aspect of life. Sustainability is defined in terms of the system’s capacity to maintain natural laws and human values in the long-term future. Sustainable criteria need to be integrated into policies and strategies at every stage of the development process with the entire life cycle of a system. Thus, it has become the main focus of modern infrastructure design. Similarly, sustainable pavement can be defined as a pavement that pleased long-life engineering reliability, with a minimum acceptable life cycle economics and environmental impacts. The need to minimize the use of scarce primary resources is becoming more urgent in the pavement industry [
27]. Thus, sustainable pavement design is based on some criteria, such as minimizing reliance on natural resources, reducing energy consumption and GHG emissions, limiting air, water, and noise pollution, and improving health, safety, risk prevention, and user comfort [
28]. Applying sustainability definition to the construction field could result in a set of processes by which a profitable and competitive industry delivers buildings, structures, and roads that improve the quality of life while maximizing the efficient use of natural resources and energy [
29]. Several studies have been conducted to evaluate the sustainability of pavements in terms of life cycle costs and/or environmental impacts [
30]. However, sustainable practices in civil infrastructure, especially in pavement engineering, are emerging concepts but slow to be applied, likely due to (a) policy and decision-makers have not been provided with the key pieces of the broad knowledge that need to make expert decisions, and (b) there is a deficit of definite sustainability assessment metrics [
31]. Sustainable pavement design procedure starts by defining the goals, benefits, requirements, and methods and identifying the key sustainability performance indicators and standard index of the pavement [
32]. Though the practice of sustainable design in pavement projects started before 2015, study reports indicate that the topic started to get more attention in the years closer to 2015. Based on these observations, the authors believe that, in addition to current developments in sustainable flexible pavement design, most of the research findings reported before 2015 would likely be referenced in the recent publications. Hence, this paper has relied on the reports of peer-reviewed research papers on various aspects of pavement sustainability published between 2015 and 2020.
The concept of sustainability is a multi-disciplinary, broad, and complex topic that touches almost every field of practice [
6]. In the field of transportation infrastructure, sustainability must be at the core of every activity from the initial planning to the final phase of the construction of the structural system. To ensure a sustainable infrastructure system, a well-designed and smart approach is imperative. The lack of a standard framework for evaluating sustainability, particularly in highway structures [
15], and globally acceptable sustainability metrics [
33] has been a hindrance. Thus, acceptable sustainability assessment tools are needed to balance the performance, economic, and environmental sustainability of pavement systems [
7].
Inconsistencies in the source and quality of data and issues [
34], lack of standardized procedure of conducting LCAs on pavements, and failure to consider the time effect of environmental impacts in pavement designs have delayed the adoption of LCA frameworks by the decision-makers [
35]. Lack of dynamic user traffic patterns, local and region-specific data resources, assumed data (based on relevant literature) or calculated data is a serious challenge in the application of LCA frameworks in both research and industry projects [
34]. Furthermore, inconsistent and inappropriate LCA in terms of the functional unit, reference service life, system boundary, lifecycle inventory, limited reporting of data sources, and lack of sensitivity and uncertainty analyses pose serious challenges in identifying the most environmentally friendly flexible pavement technology [
35,
36].
2. Framework for Design of Sustainable Flexible Pavement
2.1. Resilience Framework
The concept of resilience assessment of infrastructures was developed to understand the performance behavior of infrastructure during and after it has been subjected to extreme disturbance events [
39]. Resilience focuses on the dynamic nature of systems and their ability to function under a shock without undergoing an irreversible or unacceptable decline in their structural integrity and functionality [
40]. When a resilient system is subjected to a rarely occurring extreme damaging event, it will either withstand the event or recover from any damage it sustains from the less likely occurrence of extreme events. In the USA, for example, the National Infrastructure Advisory Council defines infrastructure resilience as the ability to reduce the magnitude and/or duration of disruptive events [
41]. Further, the Multi-disciplinary Center for Earthquake Engineering Research at Buffalo includes robustness, redundancy, resourcefulness, and rapidity as characteristics of resilience [
12]. The resilience of the infrastructure system is a crucial attribute that has gained much attention within the engineering discipline [
42]. Therefore, research on system resilience for critical infrastructure has significantly increased, and quantification of resilience has become the major factor to sustain infrastructure system functionality [
43].
Resilience metrics are used to measure the sustainability and recovery potential of a system [
44]. Several attempts have been made to define and explain resilience metrics used to judge the influence of investment and policy decision-makers in the selection of the best resilience system [
40]. Resilience can be characterized by the so-called four Rs of resilience metrics. These are:
-
Robustness: the capacity of a system and parts of the system to repel extreme event impacts without sustaining significant performance damage,
-
Redundancy: the degree of a system and parts of the system to sustain defined functional requirements in the event of a disturbance,
-
Resourcefulness: the facility to recognize and prioritize harms and mitigation reaction, and monitoring economic, technical, and performance-related problems,
-
Rapidity: the ability of a system and parts of the system to recover losses and avoid future disruptions [
12].
RA is an advanced technique of modeling that predicts the pavement structural conditions and acts proactively to maintain pavement infrastructure functionality [
45]. RA is a new, innovative, and flexible scientific-engineering procedure used to develop tailorable validated methods that can conceptualize, design, assess, and generate metrics for improved system resilience. This earned RA wide attention from academia as well as the industry [
19]. Theoretically, RA is held in a mindset of reducing failure probabilities, consequences, and recovery time during the occurrence of disturbances [
42]. Thus, infrastructure investments based on RA are used to reduce the impacts of current and future climate risks [
46] through situation assessment, rapid response, and effective recovery strategies [
47]. Extreme and prolonged moisture is the most common hydrological extreme phenomenon that causes inconvenience and widespread damage to the pavement. Continuous high-temperature variations can cause severe damage to the flexible pavement due to alternate softening and hardening of the asphalt surface, which can result in rutting and thermal cracking [
48]. Extreme weather events, such as heavy rainfall, flooding, and heatwaves can cause severe deterioration of flexible pavement [
49,
50]. To ensure the sustainability of investments over their entire lifetime, climate scientists and infrastructure engineers are engaged in the study of climate change impacts and adaptations in transportation infrastructures [
51]. Identifying and incorporating climate information into engineering design is the first step in reducing climate change impact vulnerability of pavement [
51,
52]. To address these effects at the design stage, resilience can be analyzed using different approaches such as graph theory, optimization techniques, and simulation [
14].
The resilience of flexible pavement under severe environmental events with a low probability of occurrence but the high damaging influence is a major global concern. It is difficult to accurately predict the impact of these extreme events on critical infrastructures. Further, there is no clear methodology to quantify resilience both in terms of operational and infrastructure integrity [
53]. As a result, most flexible pavements were designed based on historical climate data which does not reflect the anticipated future tendencies [
51]. Flexible pavement design and management practices must be adapted in response to future climate changes [
54]. Existing non-resilient-engineered systems may gradually downgrade toward a low-level performance and capacity due to extremely disruptive events. Resilience engineering quantities can be used to formulate an overall resilience framework [
19]. No precisely known threshold for the level of resilience of flexible pavement could be found in the literature, and it will remain a challenge to researchers unless resolved [
40]. This challenge reinforces the need for metrics to evaluate flexible pavement resilience. Thus, resilience requires thinking about the potential burden on the stability of a system and could develop countermeasures or safeguard the longstanding losses [
42]. Enhancing system resilience at a structure level could lead to massive savings through risk reduction and expeditious recovery. Therefore, a resilience pavement framework that can serve as a lens for sustainability has been developed to solve the existing gap. In the framework, the resilience concept and quantification techniques were considered in flexible pavement design to overcome resource insufficiency challenges for road authorities by satisfying the engineering constraints before and after extreme event occurrences. Infrastructure design engineers may apply status-quo, flexible, or robust design approaches to create a resilience system for low, gradual, and worst-case projected climate change scenarios, respectively [
48]. The proposed flexible pavement resilience framework is shown in
Figure 2.
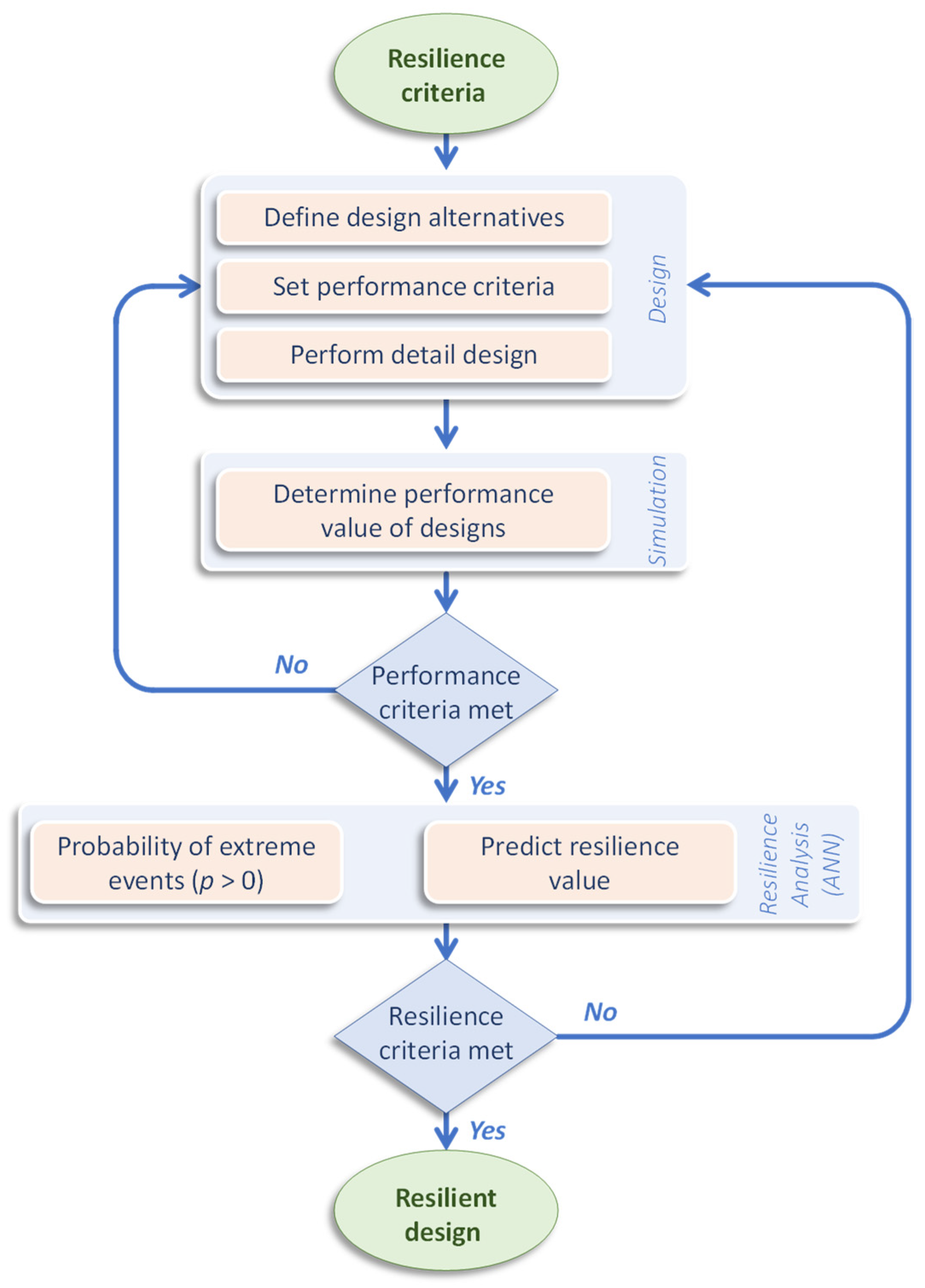
Figure 2. Flexible pavement resilience framework.
In this flexible pavement design procedure, resilience principles should be considered in advance before the reality of risk issues. Thus, resilience criteria are significant and robust for the sustainability of flexible pavement. The collective influence of climate change, augmented traffic flow burden, economic force, and community demand have impelled pavement design to take into consideration resilience criteria at the initial stage of pavement design and appraisal process. This requires integrating resilience criteria into policies, strategies, and designs at every stage of pavement development practice with the all-inclusive life cycle of the system. The resilience criteria considered in the study include:
-
Identify existing and anticipated future situations to withstand the likely traffic load and environmental influence,
-
Recognize the local context of susceptibility and exposure to extreme events,
-
Reduce the probability of failure, consequence, and time to recover.
2.2. Life Cycle Assessment Framework
The awareness of forecasting the environmental impact of construction-related activities and the need to prevent it is growing [64]. Thus, a framework useful for quantifying flexible pavement-related life cycle impacts at the design stage has been presented. The developed life-cycle assessment framework encourages an environmentally friendly construction activity by determining, at the early design phase, the potential environmental impact of the activity. However, it is difficult to acquire the data necessary to carry out the LCA before completing the design. Thus, LCA is not typically used in the design phase [36]. To overcome this limitation, a probabilistic LCA framework has been developed. The framework will be embedded in the design phase of the pavement to quantify the environmental impacts and energy consumptions in the holistic life of the pavement system. A framework for flexible pavement considers design inputs to quantify the energy consumption, amount of raw materials required, and emissions from the construction, maintenance, and end of life of the asphalt pavement [15]. The LCA framework allows an all-inclusive approach for recognizing strategies to reduce environmental influence throughout the life cycle of the pavement [8,66]. The developed probabilistic LCA framework is shown in Figure 3.
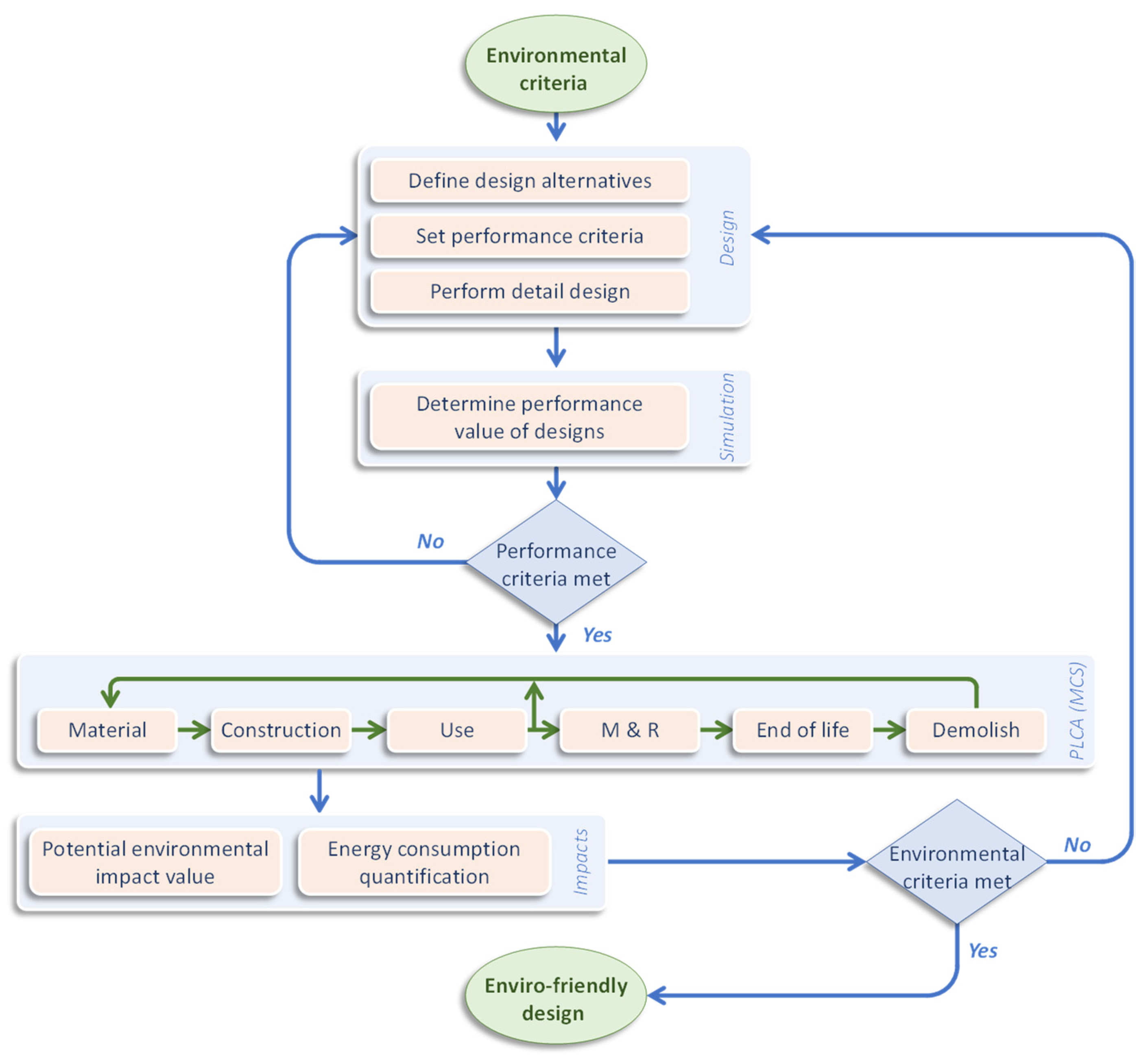
Figure 3. Probabilistic life cycle assessment framework.
The choice and use of sustainable pavement materials play an important role in environmentally-friendly pavement design. Environmentally sustainable pavement design considers drastic reduction and shift in the use of raw materials, the reduction of wastage, and replacing, renewing, and renovating of pavement materials and components. Sustainable criteria are related to material resources, energy efficiency in the manufacturing and processing of the materials, construction, and use phase of the pavement. The materials should have low environmental pollution and be less hazardous to human health. Generally, environmental sustainability criteria consider
-
Resource efficiency,
-
Energy efficiency,
-
Reducing, eliminating, or recycling wastes and ecologically unfriendly by-products,
-
Designing pavements are safe and ecologically sound throughout their life cycle.
2.3. Life Cycle Cost Analysis Framework
LCCA is an efficient method for determining the monetary influence of possible modifications in design, construction, and materials [85]. Pavement engineers and managers mainly use LCCA to make a decision [30]. LCCA is a framework to evaluate the most economically practical investment from established options over their respective lifetimes [25]. To evaluate long-term alternative pavement design investment options, the inputs are very robust [84]. Further, all the input factors in a highway project valuation modeling have uncertainties and use the technical aspects of pavement engineering in the calculations to achieve real value for the projects [86]. There are several approaches used in performing LCCA including (i) probabilistic approach which addresses the variability and uncertainty associated with the LCCA input parameters including activity cost and timing and discount rate [18], (ii) probabilistic simulation-optimization LCCA approach [82], (iii) deterministic and probabilistic approaches [87], and (iv) probabilistic and fuzzy approaches [88]. Since the result of LCCA depends on the deterioration procedure, a consistent performance forecasting model remains a key parameter in the effective execution of real-world LCCA [79]. However, experts are frequently challenged with substantial uncertainties in estimating the future costs, in both short and long terms. This uncertainty promotes a probabilistic perspective of LCCA [25]. Therefore, a probabilistic LCCA framework has been developed as shown in Figure 4.
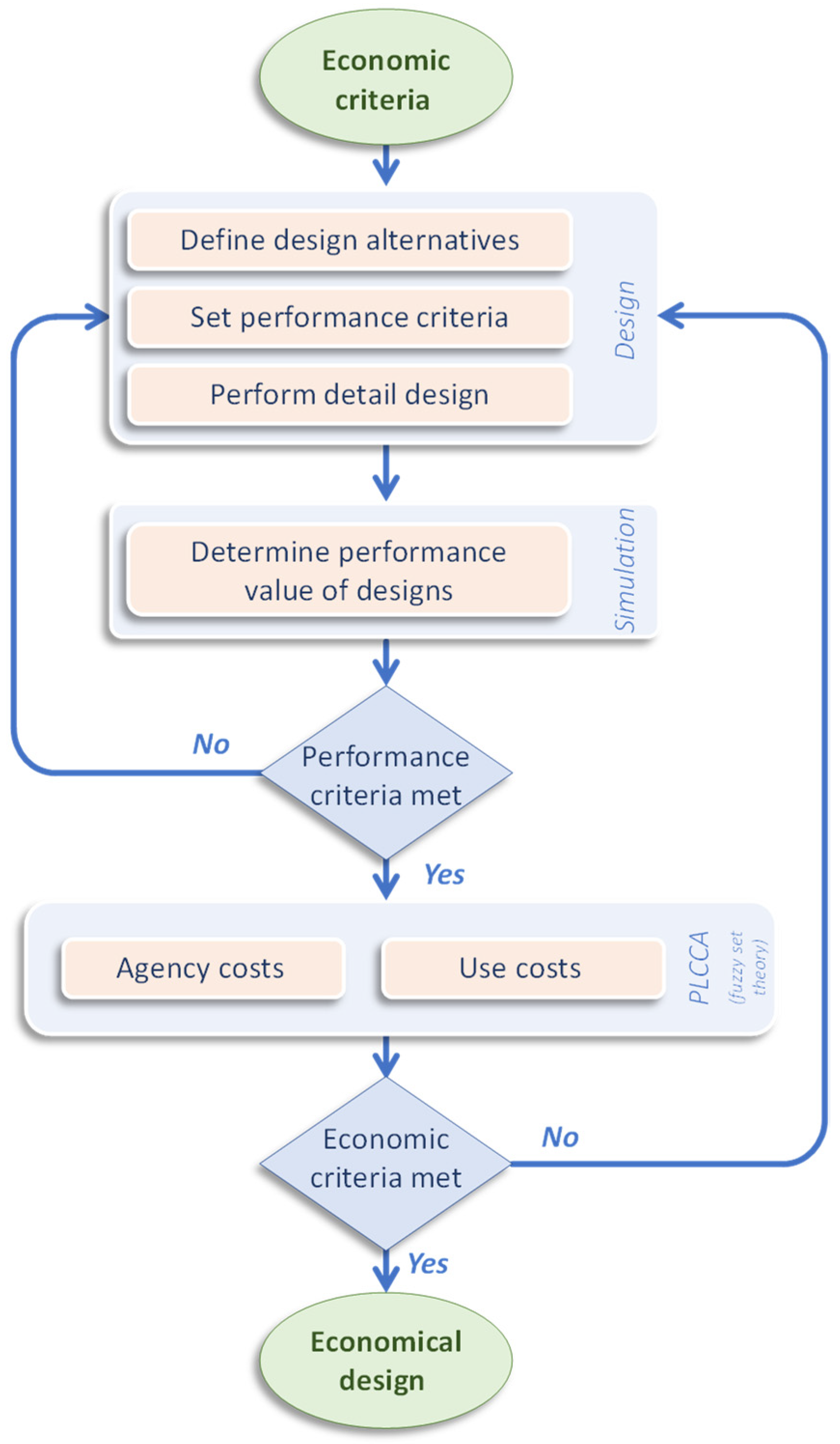
Figure 4. Probabilistic life cycle cost analysis framework.
The LCCA explicitly accounts for the alterations in material production costs, pavement life-cycle performance, maintenance triggers, maintenance effects, vehicle fuel consumption costs, and work zone user delay costs [
80,
81]. Probabilistic addresses the variability and uncertainty associated with the LCCA input parameters, including activity cost, activity timing, and discount rate [
18]. According to
Table 4, in LCCA both agency cost and user cost can be determined by probabilistic MCS or fuzzy set theory technique.
Table 4. Life cycle cost analysis techniques and cost categories.
Cost Considered |
Techniques |
References |
Agency and user cost |
Probabilistic Monte Carlo simulations |
[89] |
Agency and user cost |
Fuzzy set theory |
[90] |
Agency cost |
Probabilistic Monte Carlo simulations |
[82] |
2.4. Sustainability Framework
Sustainable pavement is durable and robust which can meet the technical requirements for a sound road, preserves and restores ecosystem related to the road, makes effective use of natural, financial, and human resources. Design can influence the sustainability of the pavement in terms of life cycle costs, performance, and the materials used. Thus, the need to minimize the use of scarce primary resources is becoming urgent in the pavement industry [
27]. Considering sustainability into pavement design provides various criteria for assessing pavement investment decisions, against environmental, social, and economic impacts. [
34].
Constraints in budgets, rapid world population growth, and an increase in travel demand have placed a significant burden on pavement systems. Hence, pavement engineers are being forced to use sustainable criteria [
31]. Several issues including augmented traffic flow, risky situation events, scarcity of quality pavement materials, and inadequate resources have triggered a declining trend in the quality of the pavement. Conventional pavement designs are not adequate in providing solutions that meet the diverse sustainability challenges. Furthermore, design variables and constraints are subject to uncertainties. The absence of a comprehensive sustainable flexible pavement design framework that considers the uncertainties of the design parameters, extreme events with high impacts, and premature failure of road infrastructures is the main challenge [
91].
Sustainable pavement design criteria is a step up aiming at maintaining a balance between the natural and built environments [
92]. It has become the focus of modern infrastructure design [
93] by promoting the main criteria for a sustainable pavement. The criteria are the use of rapidly renewable resources, recycled materials, resource assurance for the next generation, the efficient uses of resources over the life of the infrastructure, reducing greenhouse gas emissions, ensuring a high level of user comfort and safety, material conservation, and resource efficiency. By considering these criteria, the sustainability of road construction contributes to the environmental wellbeing and economic and social developments in a country [
29]. Pavement engineers need access to tools that enable them to design pavement systems that can produce reliable and sustainable solutions under a set of complex scenarios and uncertainties. Hence, this paper presents a comprehensive sustainable framework by integrating the calculus of a resilience framework, probabilistic LCA framework, and probabilistic LCCA framework into one single framework. Multi-objective optimization values of individual sustainability pillars are considered in the fuzzy composite program to determine the sustainability index. The overall framework is depicted in
Figure 5.
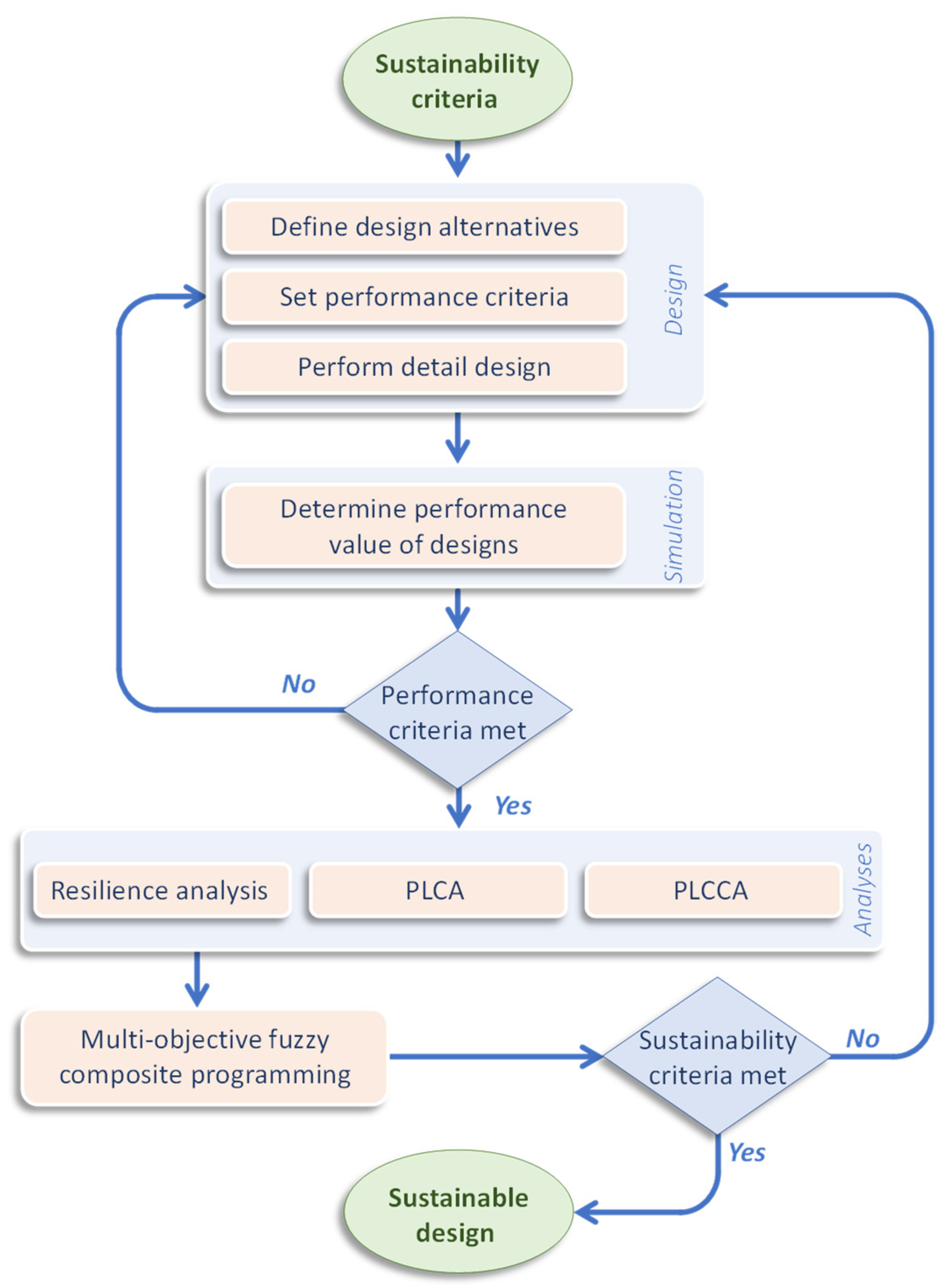
Figure 5. Framework for a design of sustainable flexible pavement.
According to Table 5, in LCA, LCCA, and PMS frameworks, decision-support tools, rating and certification tools, calculators, guidelines, and decision-support tools are used as sustainability tools. Concerning the pavement sustainability index framework, environmental, social, and economic impacts of a system are used to measure sustainability. Green Leadership In Transportation Environmental Sustainability (Green LITES), Green roads, Illinois livability and sustainable transportation (I-LAST), INVEST, and STARS are used as sustainability certification in a pavement.
Table 5. Sustainability framework, rating system, indicators, and uncertainty.
Framework |
Sustainability Tool Category/Indicators |
Uncertainty Consideration |
Rating Systems and Certification Tools |
References |
LCA, LCCA, PMS |
Decision-support tools, rating and certification tools, calculators, and guidelines |
Scenario uncertainty, variability in construction materials and methods, parameter uncertainty, and use Monte Carlo analysis |
Green LITES, Green roads, I-LAST, INVEST, and STARS |
[6] |
Pavement sustainability |
Environmental impact Social impact |
|
|
[26] |
index Framework |
Economic impact |
|
3. Conclusions
3.1. Resilience, Life Cycle Assessment, and Life Cycle Cost Analysis
There is inconsistency in the sustainability framework for the design and analysis of flexible pavement that involves a comprehensive assessment of sustainability in the design phase. To address this gap, the resilience analysis framework, probabilistic life cycle assessment framework, and probabilistic life cycle cost analysis framework were independently developed as pillars of sustainability. Then, these individual frameworks were integrated into a single framework to establish a comprehensive sustainable flexible pavement framework that can evaluate the sustainability of the pavement at its design phase. Therefore, the ultimate purpose of this research was to propose an integrated framework that can be used for a combined assessment of sustainability in the design phase of flexible pavement.
In the developed framework, the probabilities of extreme event occurrence and impact will be used in defining the resilience criteria to be used at the initial stage of pavement design. The design will be based on anticipated future traffic load situations and local context of susceptibility and exposure to extreme events; thereby, the resilience level of the design can be evaluated using machine learning techniques (artificial neural networks). The design that meets the resilience criteria will be considered as resilient design; otherwise, the preset resilience criteria will be redesigned. The resilient design, based on its functional performance, will be expected to exhibit high robustness, redundancy, rapid recovery, and resourcefulness. The final goal of this framework is to produce resilient designs that have a low probability of failure and short recovery time. Since climate change is inevitable, a resilience framework can be used as a lens for sustainability. Thus, resilience analysis is an indispensable part of pavement design.
In regard to pavement design parameters and LCA features, lack of uncertainty considerations, homogeneity, and transparency of parameters have been barriers for a harmonized application of LCA. So, in the developed PLCA framework, resource efficiency and energy efficiency will be considered as environmental criteria at the onset of the pavement design. The design will be evaluated based on the environmental criteria using Monte Carlo analysis. The design that meets the environmental criteria will be considered as environmentally friendly design; otherwise, the pavement will be redesigned until the criteria are met. Pavement material selection plays an important role in the design of environmentally friendly pavement. Thus, PLCA could be considered in the design phase to ensure the construction of environmentally friendly pavement. The proposed PLCA framework allows highway agencies to compare alternative pavement designs based on the environmental design criteria.
In the developed PLCCA framework, maximizing reliability and minimizing agency and user costs are used to define the economic criteria for the pavement design. Thus, in this framework the design will be evaluated based on the economic criteria, using fuzzy set theory. The design that meets the economic criteria will be considered as economical design; otherwise, a redesign will be required until the criteria are met. Thus, PLCCA could be considered in the design phase to ensure economically sound pavement construction. The proposed PLCCA framework allows highway agencies to compare alternative designs based on economic criteria.
3.2. Sustainable Framework
To quantify sustainability in flexible pavements, several tools were utilized. For instance, LCA is extensively applied to evaluate the environmental impacts of pavement options, resilience-based road network design, and analysis to have open accessibility of surface transportation under all-weather conditions. LCCA is an efficient method for determining the monetary influence of possible modifications in design, construction, and materials. Thus, a framework for use at the design phase of a sustainable flexible pavement was developed. The framework integrates resilience, economic and environmental sustainability frameworks. It can be used by pavement engineers as a guide to compare alternative design candidates by combining PLCCA, PLCA, and RA. The optimum design configuration will be selected based on sustainability key performance evaluation criteria. The fuzzy set theory for composite programming will be used to transform the concepts of comprehensive sustainability into practical design criteria and put them into practice. This is concluded by the selection of the optimum sustainable pavement alternatives.
This entry is adapted from the peer-reviewed paper 10.3390/infrastructures7010006