Morden Navigation aids for the visually impaired is an applied science for people with special needs. They are a key sociotechnique that helps users to independently navigate and access needed resources indoors and outdoors.
1. Introduction
Visual impairment refers to the congenital or acquired impairment of visual function, resulting in decreased visual acuity or an impaired visual field. According to the World Health Organization, approximately 188.5 million people worldwide suffer from mild visual impairment, 217 million from moderate to severe visual impairment, and 36 million people are blind, with the number estimated to reach 114.6 million by 2050 [1]. In daily life, it is challenging for people with visual impairments (PVI) to travel, especially in places they are not familiar with. Although there have been remarkable efforts worldwide toward barrier-free infrastructure construction and ubiquitous services, people with visual impairments have to rely on their own relatives or personal travel aids to navigate, in most cases. In the post-epidemic era, independent living and independent travel elevate in importance since people have to maintain a social distance from each other. Thus, there has been consistent research conducted that concentrates on coupling technology and tools with a human-centric design to extend the guidance capabilities of navigation aids.
CiteSpace is a graphical user interface (GUI) bibliometric analysis tool developed by Chen [2], which has been widely adopted to analyze co-occurrence networks with rich elements, including authors, keywords, institutions, countries, and subject categories, as well as cited authors, cited literature, and a citation network of cited journals [3][4]. It has been widely applied to analyze the research features and trends in information science, regenerative medicine, lifecycle assessment, and other active research fields. Burst detection, betweenness centrality, and heterogeneous networks are the three core concepts of CiteSpace, which help to identify research frontiers, influential keywords, and emerging trends, along with sudden changes over time [3]. The visual knowledge graph derived by CiteSpace consists of nodes and relational links. In this graph, the size of a particular node indicates the co-occurrence frequency of an element, the thickness and color of the ring indicate the co-occurrence time slice of this element, and the thickness of a link between the two nodes shows the frequency of popularity in impacts [5]. Additionally, the purple circle represents the centrality of an element, and the thicker the purple circle, the stronger the centrality. Nodes with high centrality are usually regarded as turning points or pivotal points in the field [6]. In this work, we answer the following questions: What are the most influential publication sources? Who are the most active and influential authors? What are their research interests and primary contributions to society? What are the featured key studies in the field? What are the most popular topics and research trends, described by keywords? Moreover, we closely investigate milestone sample works that use different multisensor fusion methods that help to better illustrate the machine perception, intelligence, and human-machine interactions for renowned cases and reveals how frontier technologies influence the PVI navigation aids. By conducting narrative studies on representative works with unique multisensor combinations or representative multimodal interaction mechanisms, we aim to enlighten upcoming researchers by illustrating the state-of-art multimodal trial works conducted by predecessors.
2. Narrative Study of Navigation Aids for PVI
We listed 19 remarkable works in a chronological table (Table 1) for better illustration of auxiliary solutions in PVI navigation aids. In the later narrative study, we focused on the three pivotal aspects, e.g., hardware composition, scientific focus, and validation methods.
Table 1. The chronological table of navigation aid systems for PVI.
Year |
Title of Reference |
2012 |
NAVIG: augmented reality guidance system for the visually impaired [7] |
2012 |
An indoor navigation system for the visually impaired [8] |
2013 |
Multichannel ultrasonic range finder for blind people navigation [9] |
2013 |
New indoor navigation system for visually impaired people using visible light communication [10] |
2013 |
Blind navigation assistance for visually impaired based on local depth hypothesis from a single image [11] |
2013 |
A system-prototype representing 3D space via alternative-sensing for visually impaired navigation [12] |
2014 |
Navigation assistance for the visually impaired using RGB-D sensor with range expansion [13] |
2015 |
Design, implementation and evaluation of an indoor navigation system for visually impaired people [14] |
2015 |
An assistive navigation framework for the visually impaired [15] |
2016 |
NavCog: turn-by-turn smartphone navigation assistant for people with visual impairments or blindness [16] |
2016 |
ISANA: wearable context-aware indoor assistive navigation with obstacle avoidance for the blind [17] |
2018 |
PERCEPT navigation for visually impaired in large transportation hubs [18] |
2018 |
Safe local navigation for visually impaired users with a time-of-flight and haptic feedback device [19] |
2019 |
An astute assistive device for mobility and object recognition for visually impaired people [20] |
2019 |
Wearable travel aid for environment perception and navigation of visually impaired people [21] |
2019 |
An ARCore based user centric assistive navigation system for visually impaired people [22] |
2020 |
Integrating wearable haptics and obstacle avoidance for the visually impaired in indoor navigation: A user-centered approach [23] |
2020 |
ASSIST: Evaluating the usability and performance of an indoor navigation assistant for blind and visually impaired people [24] |
2020 |
V-eye: A vision-based navigation system for the visually impaired [25] |
2.1. Hardware Composition
Early electronic aid tools use plain perceptive sensors, such as ultrasonic, infrared, or laser sensors, as the key to detecting obstacles. Subsequently, with the recent deep integration of Bluetooth, GPS, and vision sensors into navigation systems, although the types of hardware equipment have become diversified, the demands on the processing kernel have not changed. Thus, we categorize these works into three families: (1) computer as core—a system that applies computers in the process of navigation; (2) phone as core—a system with smartphones as the main carrier of information collection or information processing; and (3) standalone—a system using specified or ubiquitous chips to process and control the multisensor collaboration. Each equipment type contains two parts, ’with Infrastructure‘ means that pre-built physical facilities, such as RFID or WIFI beacons exist at the site or in the smart city
[26], whereas ’Alone‘ denotes otherwise.
Figure 1 shows statistics relating to the hardware equipment types of navigation systems. Based on their number, standalones are the most popular, whereas distance sensors are widely used to achieve object detection and avoidance
[20] with relatively simple functionalities. For example, Wojciech Gelmuda and Andrzej Kos used a multichannel ultrasonic process for high-level safety and obstacle avoidance while achieving a minimum size and cost
[9]. Because safety is the primary concern for PVI, and the price is the fundamental barrier for societal acceptance, the work described in
[9] enlightens innovations regarding possible balances between the two factors. Some systems combine RFID tags to achieve environmental information collection and positioning
[27]. Because smartphones contain numerous sensors, environmental information can be provided via BLE beacons, NFC tags, etc.
[16][28][29], and scene data can also be acquired and processed through visual sensors
[21]. Smartphones are compact and popular among PVI. Navigation aids with smartphones as the core account for more than one-third of the total, and the classifications of ’with Infrastructure‘ and ’Alone‘ are similar. Computer-based systems mainly use external vision sensors to collect the required data
[13][23] and rarely use pre-set infrastructure. Due to the enhancement of the computing power, portability, durability, and affordability of smartphones, systems with smartphones as the core have engendered a trend to replace these tasks with computers. Thus, we hypothesize that smartphone-based navigation aids will gain more favor and interest from the research community, and, in the near future, will account for the greatest proportion of navigation systems.
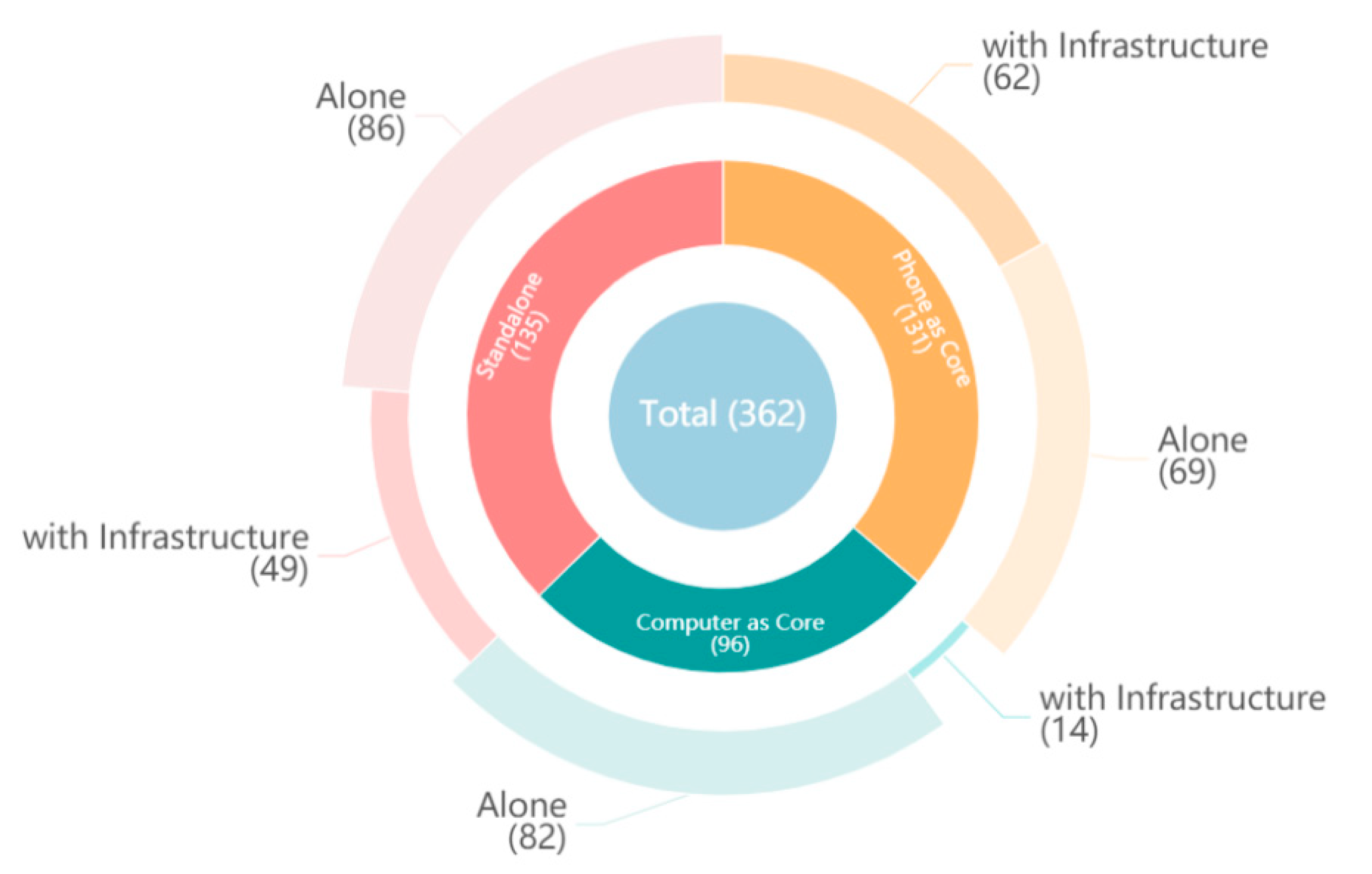
Figure 1. Hardware composition of navigation aids for PVI.
2.2. Validation Methods
Validation of the PVI navigation aids is helpful to objectively evaluate the effectiveness and value of a system. Simulations and experiments are the two primary approaches to confirm validity and performance. According to their validation method, the literature studied is categorized as shown in Figure 2.
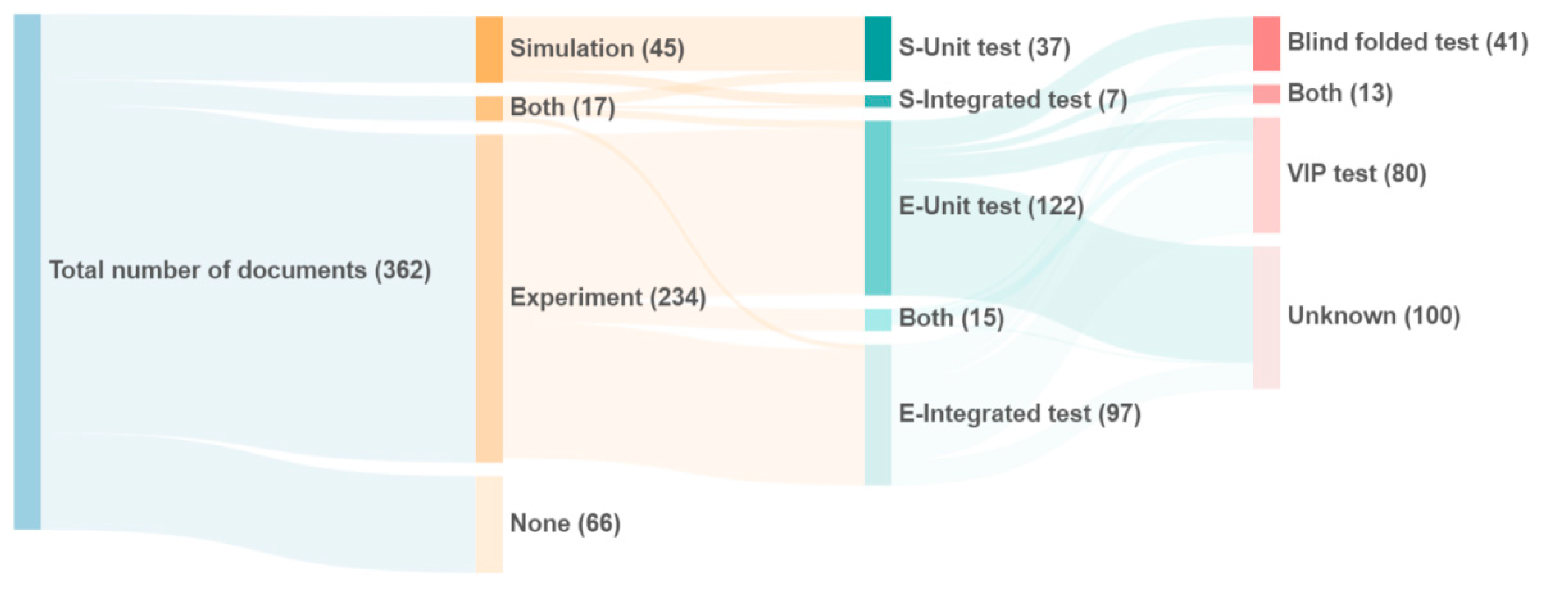
Figure 2. Statistics of validation method of the studied works.
The number of studies verified by experiments (251) is greater than the number of works using simulation (62). Among them, 17 works conducted both simulation and field tests. In simulations, unit tests are most popular to evaluate subsystems, such as mixed verification of camera calibration
[30], the capacity of proposed novel ultrasonic signals processing
[31], etc. In experiments, the results of the unit test (122) and the integrated test (97) are both numerous. Since the unit test mainly carries out verification for partial functions, such as accuracy in detecting obstacle distance
[11], in most cases, the subject type is not required or described. Experiments usually demand the evaluation of the completion of a route by subjects in a real environment, and almost 40% of the solution invites PVI to participate while the rest were with blind-folded users. Compared with simulations, in which model parameters can be adjusted adaptively and the trial quantities are not restricted, experiments may produce an authentic experience and receive feedback regarding the navigation solution by practicing in real environments. Therefore, the experimental verification method is more popular, especially for validation and verification toward human-centric metrics such as usability, feasibility, and user experience.
3. Conclusions
The main findings follow. First, as applied science, navigation aids research and development periodically reflect the status of frontier science and the economical rules of electronic products that accompany the development of personal computation devices and services; the carriers of navigation aids gradually shift from specially manufactured equipment to ubiquitous personal devices, e.g., PDA, computer, and currently, smartphones. Second, the research and developmental works focus less on fundamental theories; the most influential journal of this field shares the scope of sensory technology, assistive technology, robotics, and human-machine interactions. Third, the most active authors are with institutions in the USA and Japan. Cooperation between leading authors results in high publication output and quality. Fourth, the key references reflect the fact that wearable and compact aids, two essential means of usability engineering, draw more attention and adoption by researchers. Fifth, hybrid functionalities supported by computer vision and multimodal sensors appear to gain more focus other than conventional obstacle avoidance. Moreover, the latest progress of science and technology, such as artificial intelligence, robotics simultaneous localization and mapping, and stereo multimodal feedbacks, all allow for navigation aids to be more powerful, compact, efficient, and user-friendly. Finally, there have been numerous attempts using different sensor combinations and distinct multimodal human-machine interactions to realize the navigation aids.
However, a clear conclusion is lacking, which establishes the best way to achieve navigation aids with comprehensive consideration of affordability, usability, and sufficient functionality. One of the reasons is that it lacks a recognized guideline or benchmark for these features. Thus, besides the scientific and engineering efforts, the next stage for research and development of PVI navigation aids may require additional efforts on the user-centric factors to make systems user-friendly and practical from aspects of affordability and usability, and on cooperatively working with leading research institutions and dominating organizations (WHO, World Health Organization or RESNA, Rehabilitation Engineering and Assistive Technology Society of North America) to jointly propose recommended standards and unified evaluation indicators for the research field.
This entry is adapted from the peer-reviewed paper 10.3390/su13168795