van der Waals heterostructures are stacks of 2D sheets. The basal planes of each sheet are held strongly together by covalent bonding, while van der Waals forces keep them fixed in a sort of sandwich structure. It is not an underestimation that vdW structures can be projected as atomic-scale Lego blocks.
1. Introduction
2D sheets are less than 100 nm in a single coordinate direction. This specifies them as a thin paper or sheet-like material with a relatively expansive basal plane. Common examples are graphene (Gr), molybdenum disulfide (MoS
2), and hexagonal boron nitride (hBN)
[1]. Their unique structures induce anomalous chemical and electrochemical properties
[2][3]. The synthetic methods of vdW materials have been recently reviewed by Di Bartolomeo
[4], and therefore will not be detailed in this paper. In general, we can observe that despite the fact that many sophisticated methods have been developed for the synthesis of vdW materials, they can be fabricated in a rather easy way. One of the most frequently discussed is an exfoliation or “scotch tape” method categorized as a top-down approach. A 2D sheet may be exfoliated from its 3D crystal counterpart using this method. A bottom-up approach focuses on growing 2D sheets from small molecule precursors
[2]. van der Waals heterostructures are stacks of 2D sheets illustrated in
Figure 1. As perfect as Lego pieces match each other, vdW building blocks fit exceptionally well. Electronic coupling at the interface unlocks unique molecular characteristics, which are presumably tunable depending on the 2D sheet materials used, and other physical properties, such as layering and inter-layer distance
[5]. Stability and contaminant issues heighten the difficulty of synthesizing heterostructures and limit the amount of available 2D building blocks. Typically, high stability of the 3D bulk crystal is required before one can begin peeling off 2D sheets
[1].
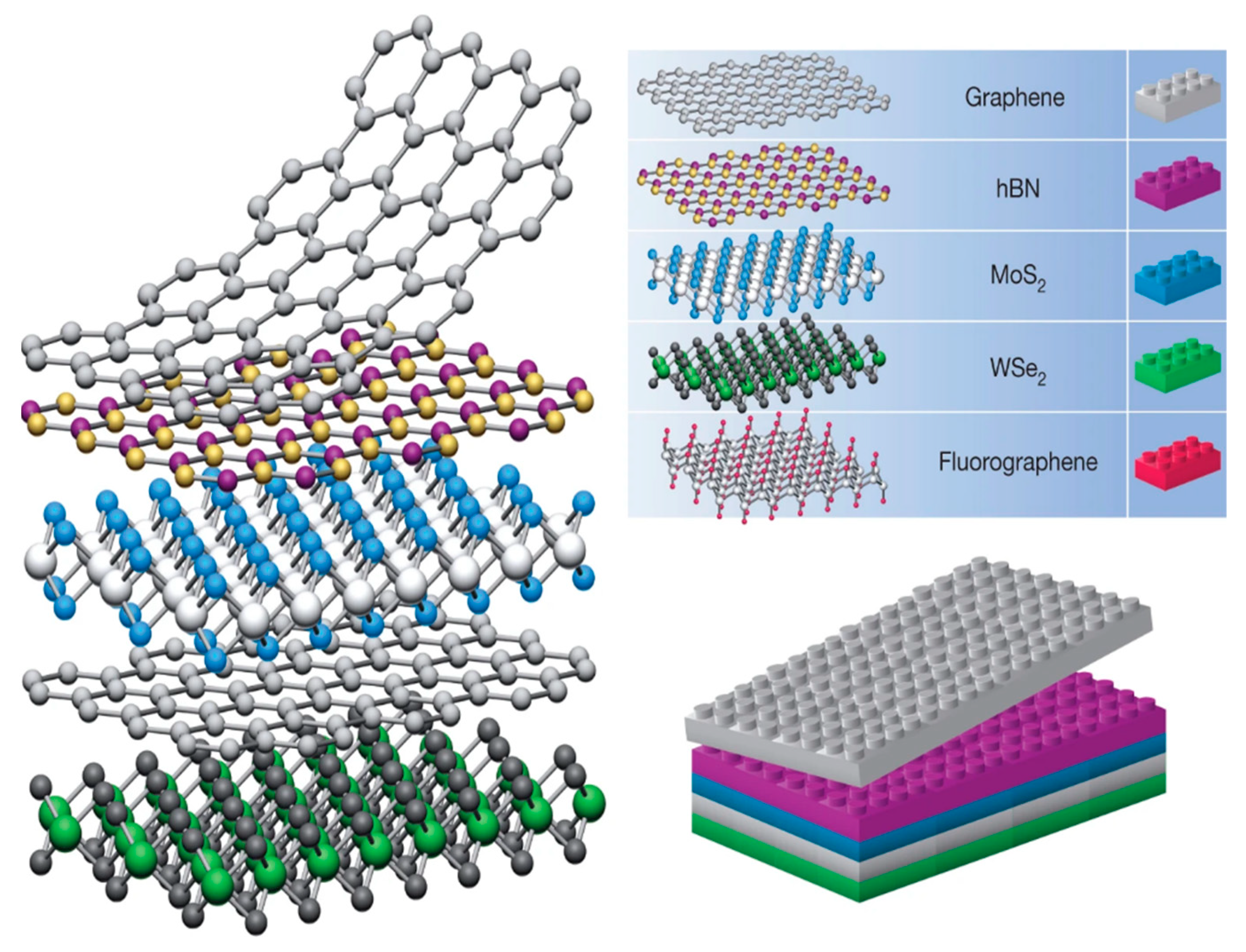
Figure 1. Structure of van der Waals heterostructures, similar to that of Legos. Reprinted (adapted) from reference
[1], copyright (2013) Springer Nature.
2. Prospective of van der Waals Heterostructures as the HER Electrocatalysts
The above discussed new vdW heterostructures offer a great foundation to develop water splitting systems to generate H2 fuel. Based on current progress in this area, several recommendations can be stated. First, stacking of the two types vdW materials (this accounts for pairing 0D/2D, 1D/2D, and 2D/2D) with one being graphene or its doped derivatives results in significantly improved HER catalysis. This can be attributed to more efficient charge transport facilitated by a more conductive component. More importantly, the subtle contact resistance between different vdW structures can be accomplished for 2D materials that were synthesized separately via wet chemistry methods. This major breakthrough inspires research for using a template-assisted synthesis procedure, allowing the control of both the macroscopic features of HER electrodes (e.g., porosity and gas permeability) together with a molecular-level design (e.g., formation of a high number of active catalytic sites in 2D space). The second important recommendation is to take advantage of an electronic coupling when stacking 2D materials with the metallic surface. First, this significantly reduces the face-to-face contact resistance and thus improves the electron transfer from the metallic surface to the vdW catalytic plane. A dual advantage can be achieved by combining the vdW heterostructure with metals containing an excess of d electrons (e.g., gold). As a result, electrons injected from the metal to a basal plane of vdW catalyst can accelerate both the charge transport and, at the same time, improve the adsorption of hydrogen on the basal plane of vdW catalysts. Despite these recent and promising discoveries, more studies are needed to solve the complexity of the mechanism of HER reaction, in particular with respect to the electron coupling effects (metal/vdW combinations). Furthermore, more affordable synthetic pathways allowing for a well-controlled confined HER catalysis are emerging areas. These challenges should inspire research groups to create more combinations of vdW heterostructures with other 0D, 1D, and 2D counterparts while embracing the best catalytic features of each component.
3. Conclusions
van der Waals heterostructures have shown their capability as possible electrode materials and electrocatalysts. van der Waals heterostructures have been shown to catalyze HER, ORR, and OER much better than their 2D counterparts owing to their unique electrochemical properties, and in some cases, rivaling precious metal catalysts, such as platinum and iridium
[6][7][8][9][10][11][12]. Graphene and MoS
2 based van der Waals heterostructures have shown particular promise as a trifunctional catalyst of all three reactions
[8]. 2D sheets of transition metal dichalcogenides studied, such as MoS
2, MoSe
2, and WS
2, are relatively stable on their own, which helps ease the synthesis of their heterostructures
[1].
Moving forward, studies on van der Waals heterostructures should focus on expanding the current library of materials, engineering more sophisticated cells with heterostructure electrodes, and improving synthetic processes for highly controllable layer and heteroatom doping to achieve fine-tuning of catalytic activity.
This entry is adapted from the peer-reviewed paper 10.3390/ma14133754