A molecular weight of 70 kDa (Hsp70) plays a particularly important role in protecting cells and the whole body from various types of stress. These proteins are molecular chaperones that restore the native conformation of partially denatured proteins after exposure to proteotoxic forms of stress and are critical for the folding and intracellular trafficking of de novo synthesized proteins under normal conditions. Hsp70s are expressed at high levels in the central nervous system (CNS) of various animals and protect neurons from various types of stress, including heat shock, hypoxia, and toxins. Numerous molecular and behavioral studies have indicated that Hsp70s expressed in the CNS are important for memory formation. These proteins contribute to the folding and transport of synaptic proteins, modulate signaling cascades associated with synaptic activation, and participate in mechanisms of neurotransmitter release.
1. Introduction
The mechanism of action of Hsps is based on their ability to interact with the hydrophobic regions of partially denatured proteins, thereby preventing their aggregation and promoting the recovery of their native conformation. Hsp70 also mediates the correct folding and transport of newly synthesized proteins under normal conditions (
Figure 1)
[1]. Proteins with similar activities are called molecular chaperones. The most versatile molecular chaperones are proteins belonging to the Hsp70 family (each member with a molecular weight of approximately 70 kDa). Hsp70 is one of the most conserved cellular proteins and is found in the cells of all studied organisms at all stages of phylogeny
[2][3]. The Hsp70 family, as well as other families of molecular chaperones, includes a group of proteins whose synthesis is induced by stress (inducible Hsp70) and several proteins that are synthesized mainly under normal physiological conditions (constitutive Hsc70)
[4]. Hsc70 primarily facilitates the folding and intracellular transport of proteins, while Hsp70 is primarily involved in refolding or degradation of proteins that have been partially damaged or denatured under stress conditions (
Figure 1). Notably, Hsp70 is a strong inhibitor of apoptosis
[5].
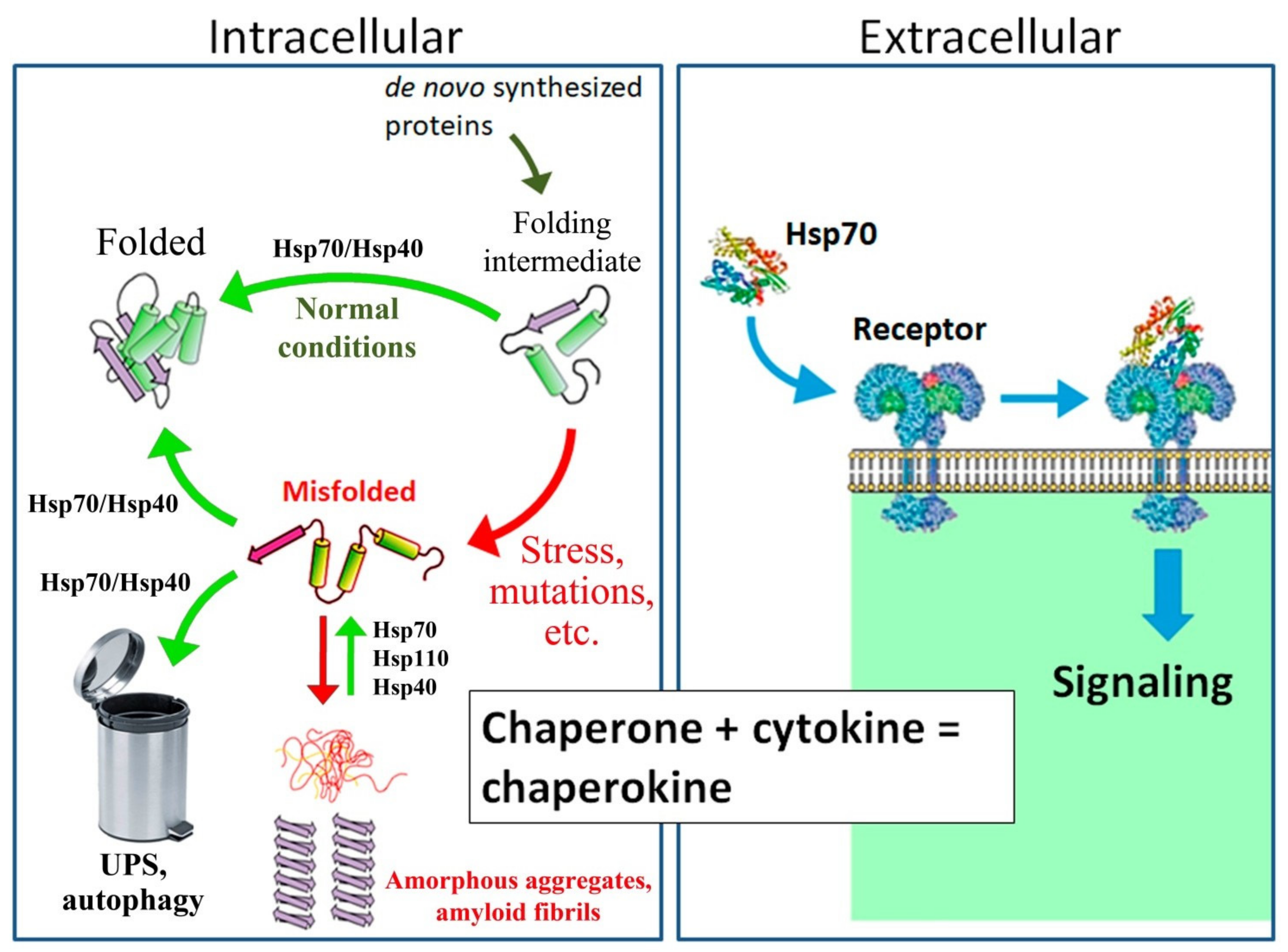
Figure 1. Mechanism of action of intracellular and secreted proteins of the Hsp70 family. As an intracellular housekeeping protein, Hsp70, in cooperation with Hsp40 and other co-chaperones, folds and sorts newly synthesized proteins in cells under normal conditions. Under proteotoxic stress and in the case of certain mutations, misfolded proteins accumulate in the cytosol. Hsp70, in cooperation with co-chaperone Hsp40, restores the native conformation of partially denatured proteins and directs irreversibly damaged proteins to the ubiquitin–proteasome system (UPS) or lysosomes by autophagy. In addition, Hsp70 in combination with Hsp110 and Hsp40 promotes the dissolution of protein aggregates, such as α-synuclein
[6][7][8][9][10]. As a secreted protein, Hsp70 is recognized by TLR2/4 and CD91 receptors and participates in the regulation of innate immunity, similar to classical cytokines; hence, Hsp70 is often called a “chaperokin”
[11][12][13][14][15][16][17][18].
Strengthening of both pre-existing and newly formed synaptic connections induced by brief high-frequency stimulation is referred to as long-term potentiation (LTP)
[19]. LTP formation is associated with enhanced neurotransmitter release and is one of the main mechanisms underlying learning and memory
[19][20]. In most synapses that support LTP, there is a postsynaptic increase in the concentration of calcium, mediated by the activation of NMDA (N-methyl-D-aspartate) receptors. An increase in calcium levels leads to the activation of CaMKII, which is expressed both in presynaptic and postsynaptic terminals. On the presynaptic side, the protein substrates for CaMKII phosphorylation include synapsin, synaptotagmin, and synaptophysin, which play key roles in the release of neurotransmitters. On the postsynaptic side, CaMKII substrates include several other proteins, such as α-actinin, PSD95, the synaptic adhesion protein densin-180, microtubule-associated protein 2 (MAP2) and neurofilament
L. Phosphorylation of these proteins leads to cytoskeletal rearrangement and structural changes in synapses, further strengthening the connection
[20]. The memorization sequence is given in
Figure 2.
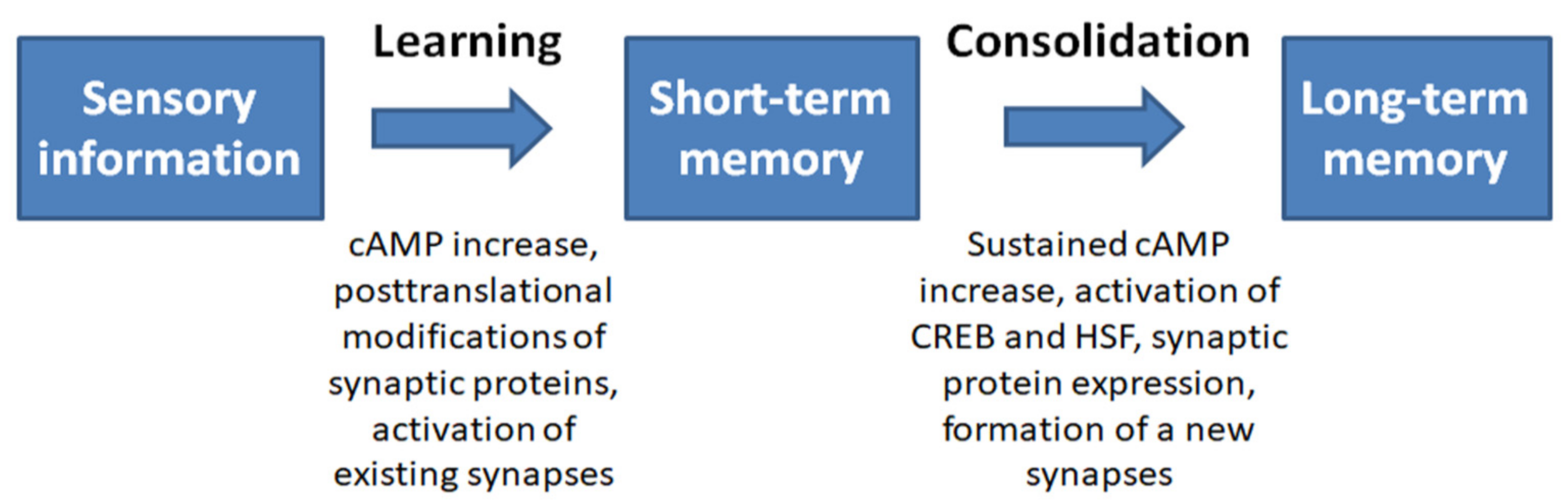
Figure 2. Summary of the sequence of events during memory formation
[21][22][23][19].
Stimuli that require memorization are often associated with various types of stress. Animals need to remember potentially dangerous environmental impacts (temperature changes, predator attacks, etc.) that require an adequate response (avoidance or defense), as well as needing to be aware of their own actions, remembering those that may lead to negative consequences. Most likely, through these connections during the course of evolution, the relationship between the stress response systems, in particular the mechanisms of Hsp70 induction, and memory was formed and consolidated. It has been shown that the stressful effects of moderate-intensity stressors contribute to the formation of LTP and memory
[24]. It has also been demonstrated that Hsp70 family members, which are universal molecular chaperones, are involved in the processes of protein synthesis and trafficking that are necessary for the maintenance of existing synapses and the formation of new synapses. Finally, Hsp70 may act as a neuroprotector, to some extent, reducing the impact of damaging factors and ageing of the nervous system, including memory deterioration. Therefore, it is evident that the relationship between Hsp70 functions and memory formation is of fundamental interest and carries promise for the treatment of age-related neurodegenerative diseases, such as Alzheimer’s disease.
2. Hsp70 Prevents Neurodegeneration and Promotes Memory Recovery in Alzheimer’s Disease Models
Exogenous Hsp70, as well as endogenous Hsp70, has a strong neuroprotective effect, as demonstrated in several AD models. The addition of exogenous Hsp70 induces microglial activation and promotes Aβ phagocytosis in vitro
[25]. Transgenic mice and
Drosophila flies secreting Hsp70 into intercellular medium have been constructed. In strains obtained by crossing extracellular Hsp70 producers with those carrying
APP transgenes expressing Aβ and prone to the development of neurodegenerative processes, a pronounced neuroprotective effect has been observed compared to the control cross or wild-type strain
[26]. Several investigations
[27][28][29][30] have shown that recombinant Hsp70 labeled with Alexa Fluor or radioactive iodine penetrates the brain when administered intranasally and accumulates mainly in the cortex and hippocampus, the structures most severely affected in AD. Sub-chronic intranasal administration of recombinant human Hsp70 has resulted in a decrease in Aβ concentration, activation of neurogenesis, and restoration of cognitive function (particularly spatial memory) in two mouse models of AD, i.e., bulbectomized mice and 5XFAD transgenic mice
[28][30].
Furthermore, analysis of the hippocampal transcriptome of 5XFAD transgenic mice intranasally treated with recombinant Hsp70 shows a significant decrease in the expression of genes critical for the development of neuroinflammation, which plays an important role in the development of AD
[31][30]. In addition, the expression of genes critical for antigen presentation, in particular, belonging to MHC classes I and II, has been shown to be significantly increased
[29]. Transcriptome studies have also shown an increase in the main neurorepair markers and increased activity of neurotransmitter synthesis systems after administration of exogenous Hsp70 (
Figure 5). In addition, intranasal administration of Hsp70 has resulted in the activation of genes involved in the regulation of the MAPK cascade
[29], which plays an important role in cell proliferation, synaptic plasticity, and memory consolidation
[32]. These data are in good agreement with the work presented in Reference
[33], which shows the modulating effect of exogenous Hsp70 on the activity of the MAPK cascade. Finally, administration of recombinant Hsp70 leads to reduced Aβ levels in the brain and restored neuron density in bulbectomized and transgenic mice
[28][30]. Chronic intranasal administration of Hsp70 to ageing mice results in a slight increase in longevity, as well as an increase in neuron density and synaptophysin levels in the cortex and hippocampus, which indicates the restoration of the synaptic network that degrades with age
[34]. Bulbectomized transgenic 5XFAD and ageing mice treated with recombinant Hsp70 have been shown to have greater memory recovery, as revealed in the Morris water maze test (
Figure 5)
[28][30].
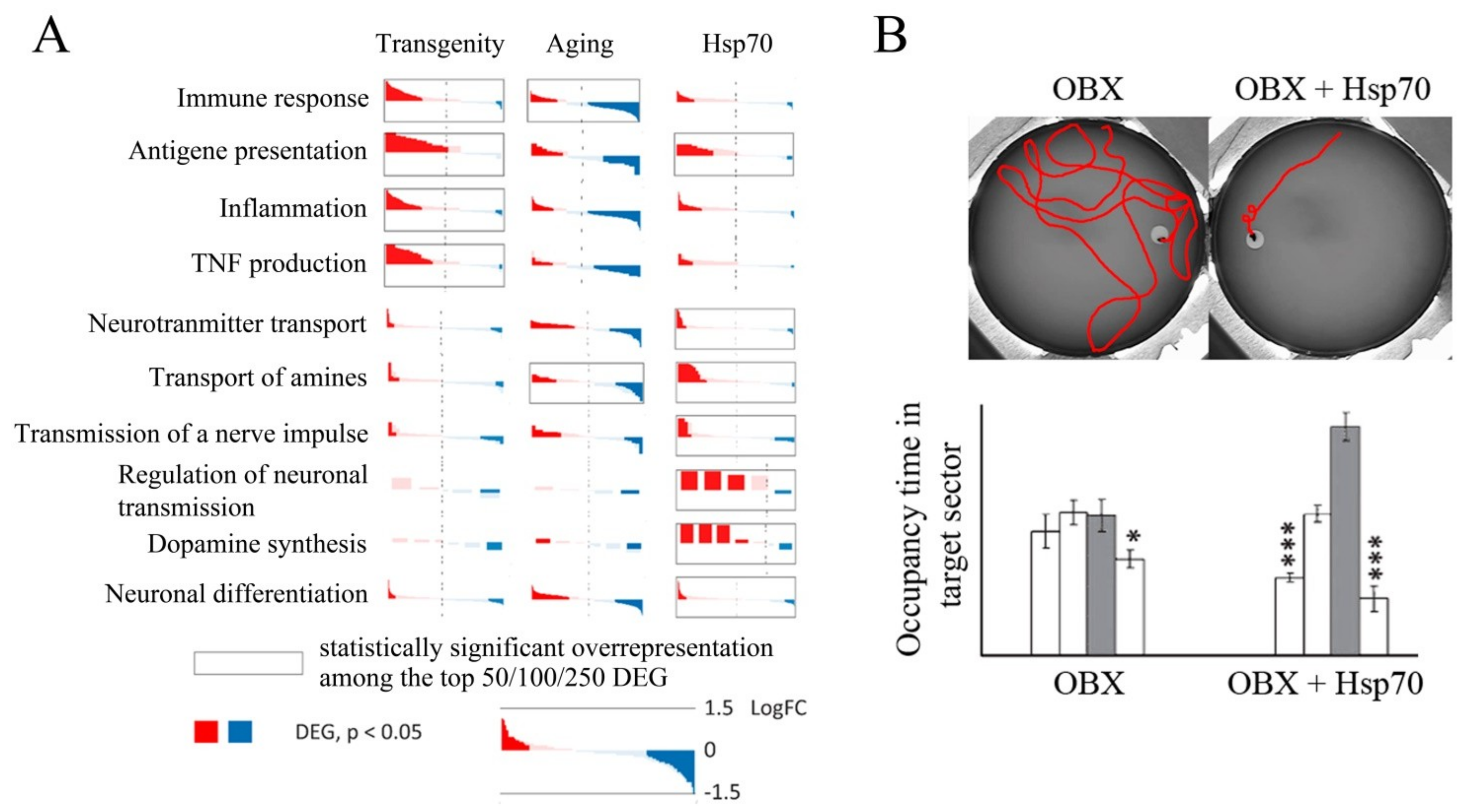
Figure 5. (A) Differentially expressed genes (DEGs) in transgenic 5XFAD mice, ageing mice, and transgenic mice after administration of exogenous Hsp70. (B) Restoration of spatial memory in bulbectomized (OBX) mice as indicated by performance in the Morris maze when exogenous Hsp70 is administered. *—p < 0.05; ***—p < 0.001.
There are several cases when human recombinant Hsp70 or Hsp70 inductors were successfully used in clinics
[35][36]. For example, the treatment with Hsp70 was applied to patients with lysosomal storage disease (LSD) which often leads to severe damage of CNS
[35]; however, application of Hsp70 in clinics is beyond the scope of this review.
Generally speaking, Hsp70 may play a dual role in memory formation and various types of neurodegeneration described above. First of all, Hsp70 may participate in different memory-related processes exploring its well-known chaperone properties. On the other hand, inducible Hsp70 sometimes found outside the cells at trace concentration, as well as exogenous recombinant Hsp70, may exercise its “chaperokin” properties and serve as a “danger signal” activating innate immunity and other protective cellular systems.
3. Conclusions
Hsp70 family members are involved in the formation and maintenance of memory in different ways. First, the stimuli that trigger the stress response and the induction of Hsp70 synthesis also activate the processes of memory and the formation of new synapses. The transcription factor HSF1 promotes not only Hsps production but also the rapid synthesis of synaptic proteins. Furthermore, Hsp70 functions as a molecular chaperone, facilitating the transport and folding of synaptic proteins and modulating the signaling cascades involved in the formation of synapses. Furthermore, Hsp70 (both endogenous and exogenous) protects neurons and synaptic structures from damage under stress (heat shock, hypoxia, etc.). Finally, Hsp70 interferes with the oligomerization and aggregation of proteins prone to amyloid formation, preventing neurodegeneration. Taken together, the accumulated data allow us to consider Hsp70 synthesis inducers and recombinant Hsp70 per se as promising neuroprotectors.
This entry is adapted from the peer-reviewed paper 10.3390/cells10071638