Fissure ridges consist of elongated travertine masses with an apical fissure that follows the long axis of the body. Two symmetrical or asymmetrical walls made up of bedded travertine are typically dipping away from the central fissure. The internal part of the fissure is often cut by a network of sealed fractures, almost parallel to the long axis of the ridge, normally filled by banded Ca-carbonate (i.e., calcite and/or aragonite), almost parallel to the vein-walls (the so called “banded travertine” in). These veins developed within the fault zone and represent conduits along which geothermal fluids move towards the surface. Therefore, their analysis, in terms of geometry, age (using U/Th, U/Pb, and 14C geochronology), and geochemical properties, provides information about the structural features and timing of the fault system, and on the fluid path from depth to surface. Furthermore, travertine deposited in a fissure ridge is characterized by distinct petrographic and geochemical features preserving information on the parent fluids and ancient depositional conditions. The shape of the fissure ridge body depends on the flow rate, carbonate precipitation rate, and surface topography upon which deposition took place.
1. Introduction
In geothermal areas with carbonate reservoirs, thermal springs and travertine deposition have inseparably combined the effects of brittle deformation at shallow crustal levels (
[1][2], and references therein]). When the high pCO
2 hydrothermal fluids are flowing from a spring, CO
2 rapidly degases due to the lower atmospheric pCO
2. This leads to oversaturation near the surface zone with respect to the calcite that therefore precipitates close to the spring, according to the well-known reaction: 2HCO
3− + Ca
2+ = CaCO
3 + CO
2 + H
2O.
The high encrusting capacity of fluids at the surface and along the conduits at a relatively shallow depth implies that renewed permeability has to be guaranteed by tectonic activity in order to maintain the flowing of fluids. Curewitz and Karson
[3] described two different primary mechanisms controlling the permeability’s maintenance through time in fault zones permeated by hydrothermal fluids. These mechanisms guarantee the (i) dynamically or (ii) kinematically maintained permeability. The first case (i) implies faults that form and sustain the propagation of newly formed fractures in the rocks. In this case, the fracture propagation at both tip-zones and wall-zones, linked to the stress concentration, and related thermal springs leads away from the main fault. The second case (ii) can be characterized by faults with intermittent movement that progressively re-open pre-existing filled fractures (i.e., veins) along the fault. This implies that thermal springs are active during periods of tectonic activity, following the seismic cycle of faults (
[4], and references therein).
The neologism “travitonic” was introduced by Hancock, et al.
[1], after suggestion by Prof. Robert Folk following the idea proposed by Muir-Wood
[5], to fix the concept that travertine deposition and tectonic activity are inseparable processes. Among others
[6][7], the paper by Hancock, et al.
[1] opened a new perspective for neotectonic studies, shedding light on a new research approach based on decoding travertine deposits for tectonic analyses. Thus, since 1999 several researchers have applied the “travitonic” concept to seismotectonics and neotectonic investigation in geothermal areas around the world. Among the travertine bodies deposited from hydrothermal fluids discharged from thermal springs, fissure-ridge-type travertine deposits
[8] have attracted most researchers, being the most prominent spring-related travertine deposit formed along the traces of brittle structures and therefore consolidating the strict link between travertine deposition and faulting
[9][10]. It was later shown that the fissure ridge’s shape and internal architecture can reveal much about the geometry and kinematics of the main structure hidden by the travertine body
[11].
Fissure ridges consist of elongated travertine masses with an apical fissure that follows the long axis of the body. Two symmetrical or asymmetrical walls made up of bedded travertine are typically dipping away from the central fissure (Figure 1).
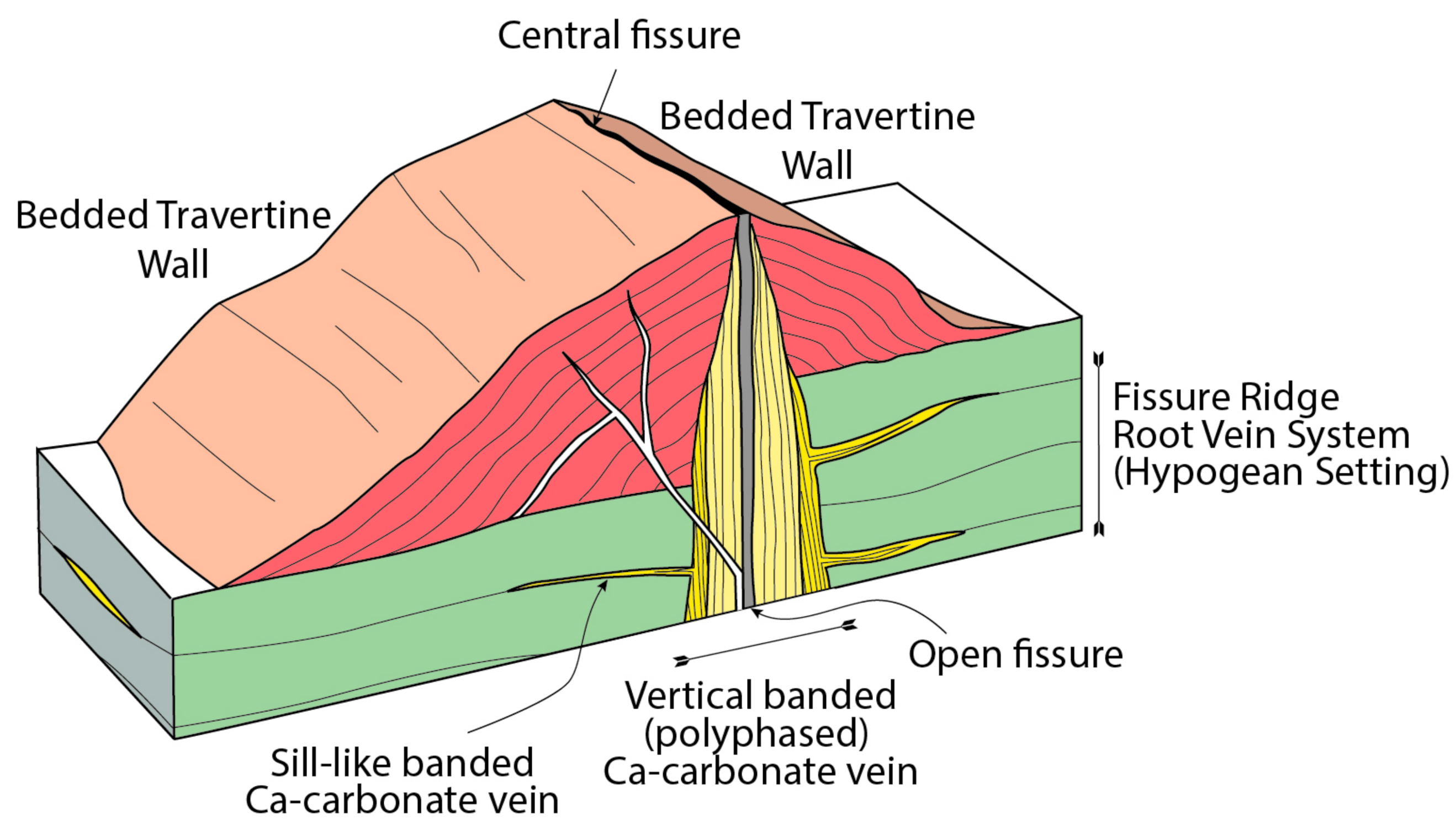
Figure 1. Diagram showing the anatomy of a fissure-ridge-type travertine deposit.
So, here below we describe the state of the art and updated knowledge on the fissure ridges, summarizing a large amount of information recently published on different fissure ridges described in various geothermal areas of the world. In different sections, we deal with: (i) the fissure ridge morphology; (ii) travertine facies developing on the wall-slopes; (iii) growth mechanisms, (iv) banded Ca-carbonate vein features crossing the fissure ridge and its substratum; and (v) tectonic environments in which fissure ridges have been described to develop. We also aim to emphasize how these results update the potentiality of the fissure-ridge-type travertine deposits for neotectonics and seismotectonic investigations.
2. Advantages of Using Fissure Ridges for Neotectonic and Seismotectonic Studies
A major advantage of investigating fissure-ridge-type deposits is that they are intimately related to a fault that produced (actively or passively, see the previous section) permeability at the time of its development. Permeability maintenance might be related to episodes of increased earthquake faulting following the suggestion of Brogi, et al. [2, and references therein] that, during earthquakes, co-seismic strain will re-open cracks previously sealed by Ca-carbonate precipitation, thus expelling geothermal fluids from the reservoir to the surface. A new cycle of carbonate deposition occurs along the wall slopes or within neoformational fractures (i.e., banded Ca-carbonate veins) and each Ca-carbonate band corresponds to a permeability enhancement period and possibly to a tectonic pulse
[1][5][12].
This background, coupled with the fact that Ca-carbonate can be dated by the U-series method and the
14C method and integrated by palaeomagnetic signatures
[13], allows us to consider fissure-ridge-type travertine deposits as favorable (almost unique) deposits for neotectonic and seismotectonic studies.
Information from paleoseismicity is also possible; in fact, our understanding of the recurrence of earthquakes at timescales longer than instrumental and historical records can provide considerable advantages through U-Th dating of Ca-banded calcite veins forming in fissure-ridge-type travertine deposits, which are able to record more than 400 ka of an earthquake’s history
[14].
In a fissure ridge along the North Anatolian Fault, this hypothesis was tested directly for the first time with robust historical earthquake records and paleoseismic data
[15]. The results suggest that the events recorded in Ca-carbonate veins indicate an epicenter with a distance of <200 km and high-intensity (I > VI) paleoearthquakes; thus, this tool has advantages over traditional paleoseismological methods for the understanding of long-term earthquake behavior. In this view, it should be possible to use age determination to reconstruct the seismicity and its recurrence
[16][15][14], the fault dilatation rate
[17], the age of a fault
[18][15][19][20], an assessment of the slip rate
[21], and relationships between faulting and fluid properties in geothermal fields
[2][22][23][24][25][26][27][28][29][30].
3. Concluding Remarks and Neotectonics/Seismotectonics Implications
Travertine is a useful proxy for the reconstruction of the fluid features in geothermal areas. The presence of travertine, as well as the associated Ca-carbonate vein network in the substratum, suggests a widespread hydrothermal circulation within a carbonate reservoir at depth.
Fissure ridge occurrences and aligned mounds indicate that fluid resurgence is (or was) tectonically controlled by faults and/or fractures.
Analyses of a fissure ridge can reveal much about the tectonic regime that favored (or is favoring) the maintenance of permeability and, therefore, the fluid flow. Isotopic analyses and determination of the age of the bedded travertine forming the ridge and each band forming the Ca-carbonate veins crossing the ridge and the substratum have a twofold fallout: they allow us to date the main tectonic pulses, helping us to reconstruct the paleoseismic history of the area, and the climate fluctuations/fluid–rock interactions generated by the geothermal fluids.
The dilatation of the central fissure and the age and thickness of the banded veins indicate the deformation rate.
Finally, the growth of fissure-ridge-type travertine deposits with active thermal springs implies the existence of active faults.
This entry is adapted from the peer-reviewed paper 10.3390/geosciences11070278