Pesticides are pollutants found in wastewater due to increasing agricultural activities over the years. Inappropriate dosing of pesticides results in the dispersal of active ingredients in the environment. The complete removal of pesticides from wastewater is an immediate concern due to their high toxicity and mobility. At present, adsorption is one of the most widely used methods for pesticide removal, in which synthetic zeolites and mesoporous silica materials are extensively applied. This article presents a systematic and comparative review of the applications and comparison of these adsorbents, based on the data reported in the literature.
1. Introduction
Since the latter half of the nineteenth century, extensive agricultural use of plant protection products, which are referred to as “pesticides” (used henceforth in this paper), has been observed to pose a serious impact on soil, air, and water. As stated by the World Health Organization (WHO), the term “pesticide” is defined as any chemical compound that is used to kill pests (weeds, rodents, insects, fungi) [
1]. The global demand for increased food production has not only led to significant deterioration of food quality, resulting in severe consequences on the environment but also caused public health issues due to overuse or misuse of pesticides [
2,
3]. It is assessed that more than 20% of the pesticides reach their nontarget species, as well as air, water, and soil [
4]. Traces of these products are commonly detected in surface water, and more importantly, groundwater—a major source of drinking water on the world [
5]. The presence of many types of pesticides and their derivatives in water is of great concern to the public and authorities, due to increased undesirable health effects resulted in the exposition on pesticides even at very low concentrations (pg/L to ng/L) [
6].
Pesticides have greatly contributed to increasing agricultural yields by limiting pests and plant diseases and also by combating the insect-borne diseases in the human health sector [
3,
7]. For example, the production of food grains has increased dramatically in several countries since the implementation of pesticides. Although increases in productivity are attributed to different factors (e.g., use of fertilizers, better plants variations, and use of better machinery), pesticides have been an integral part of those processes by the reduction of losses from the weeds, diseases, and insect pests [
8,
9]. Furthermore, insecticides are the only way available to control the proliferation of deadly insect-borne diseases like malaria which results in an estimated 5000 deaths per day [
10].
However, overuse and misuse of pesticides may have a negative impact on human health. Pesticides are used for controlling living species—they are biologically active substances that intervene with organisms and are characterized by different levels of toxicity [
11]. These compounds are relatively stable and can bioaccumulate in living bodies. The toxicity of pesticides can be categorized as acute or chronic. Acute illness generally emerges after a short time of contact with the pesticide [
12,
13]. Suspected chronic effects resulting from regular exposure to small doses of certain pesticides may include birth defects, toxicity to fetus, genetic changes, blood, and nerve disorders. Furthermore, several studies have established a link between the exposure to pesticides and the frequency of chronic diseases that affect the nervous, reproductive, renal, cardiovascular, and respiratory systems in humans [
14,
15,
16,
17].
Based on their use and ability to kill organisms, pesticides can be classified as follows: insecticides, herbicides, rodenticides, fungicides, molluscicides, bactericides, avicides, virucides, algicides, acaricides, and miticides [
9,
18]. They can also be classified into four main groups according to the chemical nature of their active ingredients as follows: organochlorines, organophosphorus, carbamates, and pyrethrins and pyrethroids [
19]. A brief description of those groups is presented in
Table 1. In addition to the four main groups of pesticides mentioned above, there are few miscellaneous groups that are worth mentioning, such as phenoxyacetic acid (e.g., 2,4-D (2,4-dichlorophenoxyacetic acid) herbicide) or bipyridyls (e.g., paraquat and diquat herbicides). Inorganic pesticides are a minor category and include sulfur, copper, mercury, lead, and arsenic compounds. These pesticides are identified as extremely persistent and have caused serious problems of soil pollution in some areas; therefore, many of these are restricted [
18,
20,
21].
Table 1. Characteristics of the main groups of pesticides.
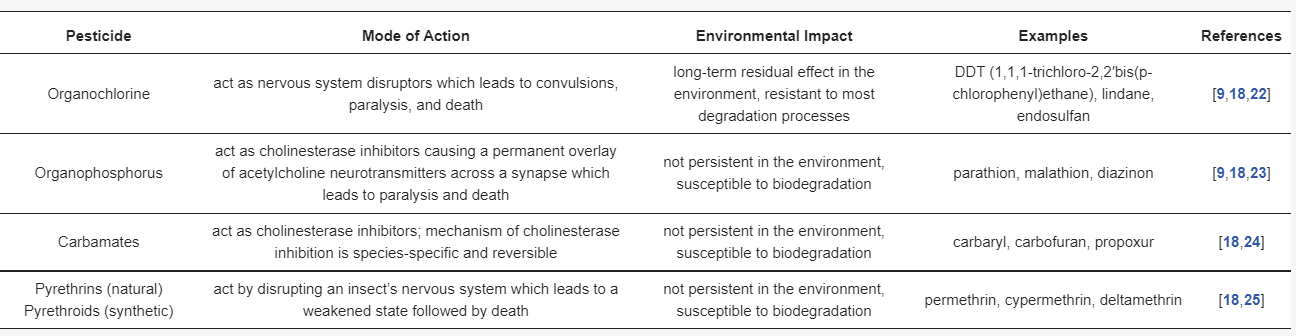
Currently, the removal of pesticides and their derivatives from the environment is one of the worldwide environmental alarms. Due to the wide use of different types of pesticides, it is extremely difficult to develop a single universal method for their removal. Basically, three groups of methods are used for pesticide remediation: biological, chemical, and physical (Figure 1). Biological remediation results in the transformation of organic compounds into harmless products such as CO2 and H2O [26,27,28,29,30,31,32]. These methods are of low cost and believed to be more environmental-friendly compared to the physical and chemical remediation methods. In chemical remediation, pesticides are converted into harmless compounds through certain agents by the way of chemical reactions [33,34,35,36,37,38,39,40,41]. Chemical treatment is usually combined with physical remediation processes; however, the costs of these combined treatments are very high and vary depending on the matrix [42]. Physical remediation is based mostly on the process of adsorption, which is one of the most commonly used methods for water purification because of its capacity, efficiency, and applicability on a large scale [3,22,43,44,45,46,47,48,49].
Figure 1. Methods used for pesticide remediation.
2. Characterization of Adsorbents
2.1. Mesoporous Silica Materials
Mesoporous silica materials are a new class of absorbents containing periodic arrays of channels and cavities [
55]. The International Union of Pure and Applied Chemistry (IUPAC) defines them as materials with pore size in the range of 2–50 nm and exhibit an ordered arrangement of pores, which is responsible for their ordered structure [
56,
57]. The formation of those inorganic materials is based on the utilization of ordered surfactants as a template for the condensation of sodium silicate or silicon alkoxides around it [
58]. Although the beginning of the synthesis of mesoscopic materials is dated back to the 1970s, mesoporous silica received attention only in the 1990s, when the Mobil Research and Development Corporation first synthesized mesoporous material from aluminosilicate gels with different morphological characteristics using the liquid-crystal template mechanism. Those materials were called Mobil Crystalline Materials or Mobil Composition of Matter (MCM) [
56,
57]. A few years later, silica nanoparticles with larger pores and thicker silica walls were produced at the University of California, Santa Barbara, which were named Santa Barbara Amorphous (SBA)-type materials [
59]. Nowadays, there are various types of mesoporous silica materials available with different structural characteristics and functional groups [
60].
The properties of mesoporous silica materials obtained depend strictly on the method used and the parameters of synthesis. These materials can be synthesized with different porosity, morphology, particle sizes, as well as with different functional groups, and hence, their physical and chemical properties can be tuned [
61,
62]. Usually, they have an enormous specific surface area (1000 m
2/g or more), homogeneous pore distribution, and large volume and size of pores [
63,
64]. Generally, MCM-41 has a hexagonal structure with a pore diameter of 2.5–6 nm. MCM-48 has is cubic, whereas MCM-50 has a lamella-like arrangement [
65]. SBA-type mesoporous silica materials differ from MCM type as they have larger pores with a size of 4.6–30 nm and thicker silica walls [
66]. Based on the template used, SBA-based silica may be designated as SBA-11 (cubic), SBA-12 (three dimensional hexagonal), SBA-15 (hexagonal), and SBA-16 (cubic cage-structured) [
56,
67,
68]. The structures of selected mesoporous silica materials are presented in the
Figure S1.
Due to their diverse properties, mesoporous silica has a broad range of potential applications. In particular, they are used in medicine as, for example, delivery vehicles for pharmaceutical and biological molecules, host materials for bioimaging or biocatalytic agents, and platform materials for sensory or catalytic moieties [
61,
69,
70,
71]. They are also useful for the immobilization and separation of CO
2, heavy metals, organic pollutants, volatile organic compounds, bioactive molecules, pigments, and dyes and thus play an important role in environmental protection [
58,
69,
72,
73,
74]. Moreover, mesoporous silica materials attract a lot of academic interest because of their wide application in catalyst chemistry, electrochemistry, and energy storage [
75,
76,
77,
78].
2.2. Synthetic Zeolites
Zeolites include more than 50 aluminosilicate minerals with the general formula:
where M is any alkali or alkaline earth atom, n is the charge on that atom, x is a number varying from 2 to 10, and y is a number varying from 2 to 7 [
79]. Zeolites have a three-dimensional crystalline structure made of AlO
4 and SiO
4. The connection of the atoms forces the structure of the zeolite—four oxygen atoms are located at the corners of each tetrahedron and are shared with the adjoining crystal tetrahedral, and each tetrahedron in the framework contains silicon or alumina as its central atom [
79,
80,
81,
82]. Such orientation of atoms results in the development of the structure full of pores and empty voids formed as cages and channels [
83]. The crystalline lattice structure of zeolites is characterized by unique lattice stability and allows ion exchange as well as the accommodation of water molecules, new cations, and small organic molecules. Molecules and particles occurring in the voids and pores are loosely bound, and therefore, all the mentioned processes are reversible with no damage caused to the zeolitic framework [
79]. However, this feasibility is determined by the crystalline structures and chemical composition and of a particular zeolite. The type of zeolites formed depends on the temperature, pH pressure, concentration of the reagent solutions, process of activation and aging period, and the contents of SiO
2 and Al
2O
3 in the raw materials [
80].
The most important zeolites physical properties are their bulk density, specific surface area, specific gravity, radius and volume of pores, which can correlate with the porosity of the materials, and cation exchange capacity (CEC) [
84,
85,
86]. The CEC and adsorption properties, pH, and loss on acid immersion of zeolites are some of the chemical properties characteristic of zeolites and depend on the chemical composition of the zeolite. In general, for zeolites, a raise in the Si/Al ratio (from 0.5 to infinity) leads to changes in various parameters—acid resistivity, thermal stability, and increase in hydrophobicity, while hydrophilicity, acid-site density, and cation concentration may decrease [
85,
87]. The properties of synthetic zeolites are also influenced by the method used for synthesis and its related parameters. Both the surface area and CEC of zeolites exhibit substantial variations with a raise in alkali concentration and reaction time [
84]. For example, the surface area tends to raise with an increase in the concentration of alkaline solution and the reaction time, while the CEC decreases with increasing concentration of alkaline solution; however, CEC also fluctuates arbitrarily with a raise in reaction time, which is usually ascribed to the changes in the size and volume of the pores [
79]. The frameworks of selected zeolites are presented in the
Figure S2.
The potential applications of zeolitic materials in industrial field depends on their type and properties. Zeolites (especially those with high CEC) can be applied in water purification. In particular, the use of zeolites has been extensively tested in solutions containing heavy metals and ammonium [
88,
89,
90], which are commonly used as molecular sieves in gas purification technology [
89,
91]. Zeolites, as the most important solid catalysts, are used in traditional petrochemical industries, especially in cracking, isomerization, and hydrocarbon synthesis [
92]. These materials are also used as detergent builders, as in contrast to conventional detergent builders, they are more environmentally friendly due to their ability to lower the hardness of water and insolubility [
93]. Moreover, Canpolat et al. [
94] showed that the use of zeolite as a replacement material in the production of cement increased the compressive strength of the obtained cement products. Besides these applications, zeolites play a significant role in many sustainable processes, especially in the fields associated with renewable energy and environmental protection, such as biomass conversion, fuel cell production, thermal energy storage, agriculture, or biomedicine [
92,
95,
96].
This entry is adapted from the peer-reviewed paper 10.3390/ma14133532