Gestational diabetes mellitus (GDM) is a common metabolic disorder that often develops during pregnancy, characterized by glucose intolerance and insulin resistance (IR). To ensure the well-being of both the mother and the fetus, the body undergoes multiple metabolic and immunological changes that result in peripheral IR and, under certain hereditary or acquired abnormalities, GDM in predisposed women. The adverse short- and long-term effects of GDM impact both the mother and the fetus. Nutrition seems to play an important role to prevent GDM or improve its evolution. An emphasis has been given to the proportion of carbohydrates (CHO) relative to protein and lipids, as well as dietary patterns, in GDM. The effects of CHO on postprandial glucose concentrations are reflected in the glycemic index (GI) and glycemic load (GL). Diets rich in GI and GL may induce or exacerbate IR, whereas diets low in GI and GL appear to enhance insulin sensitivity and improve glycemic control. These positive outcomes may be attributed to direct interactions with insulin and glucose homeostasis or indirect effects through improved body composition and weight management.
1. Introduction
Gestational diabetes mellitus (GDM) is one of the most common pregnancy complications, with a steadily increasing prevalence, in parallel to the global rise in type 2 diabetes mellitus (T2DM) and obesity. Approximately 15% of pregnancies worldwide are complicated by GDM
[1].
Pregnancy undergoes various anatomical, biochemical, physiological, and hormonal changes to meet the increased metabolic demands for fetal development. During early gestation, insulin sensitivity increases in order to control glucose metabolism and promote the uptake of glucose into adipose stores in preparation for the energy demands of mid- and late pregnancy. In adapting to ensure an adequate supply of carbohydrates (CHO) for the fetus and consequently maintaining the necessary glucose levels to meet its energy demands, a state of peripheral insulin resistance (IR) is gradually established
[2]. As a result, blood glucose is slightly elevated across the placenta to fuel the growth of the fetus
[3]. Thus, as the pregnancy progresses, a surge of hormones, including estrogen, progesterone, leptin, cortisol, placental lactogen, and placental growth hormones together promote a state of IR
[3]. As part of the normal response, maternal tissues such as muscle and fat become relatively insulin-resistant. These maternal tissues increase the use of other fuel sources, such as fatty acids and ketone bodies
[4]. Increased estrogen, progesterone, and insulin favor lipid deposition and the accumulation of maternal fat stores in early and mid-pregnancy and enhance fat mobilization in late pregnancy
[5]. Protein catabolism is decreased as fat stores are used to provide energy for metabolism
[6][7][8]. Hyperinsulinemia ensues as a compensatory response to the increased insulin production by β-cells as a means of compensating for the IR
[1]. In addition to the hormonal imbalance, gestational IR is further exacerbated by genetic and epigenetic variables
[9], increased visceral fat, altered gut microbiota
[10], and obesity (
Figure 1).
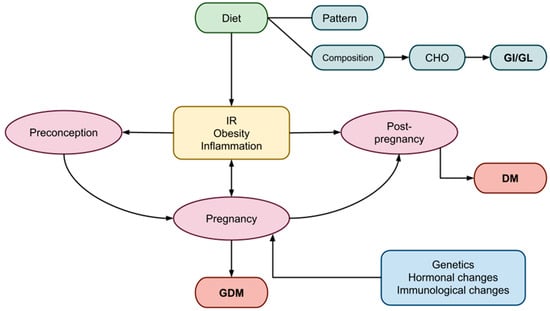
Figure 1. Factors influencing the periods of preconception, pregnancy, and post-pregnancy, leading to GDM. GDM, gestational diabetes mellitus; DM, diabetes mellitus; CHO, carbohydrates; GI, glycemic index; GL, glycemic load, IR; insulin resistance.
Also, a number of signaling pathways may play a role in the pathophysiology of GDM; NF-κB contributes to the development of GDM by promoting adipocyte inflammation and impairing insulin-related functions, such as glucose uptake, peroxisome proliferator-activated receptors (PPARs), sirtuins (SIRTs), 5′ AMP-activated protein kinase (AMPK), glycogen synthase kinase 3 (GSK3), PI3K/mTOR, inflammasomes, and the endoplasmic reticulum (ER)
[11]. These key pathways often interact with and alongside each other, as T2DM studies have shown. Unfortunately, there is no clear evidence to indicate that these pathways act and contribute to GDM development
[12].
A growing body of research highlights that hyperglycemia during pregnancy influences epigenetic processes, inducing changes through histone modification, DNA methylation, and the disrupted function of non-coding ribonucleic acid (ncRNA), including microRNAs (miRNAs)
[13][14]. Mainly epigenetic changes lead to the dysregulation of gene transcription, modifying the phenotype of the developing child
[15][16]. Short-term effects on offspring include respiratory distress syndrome, hypoglycemia, hyperbilirubinemia, hypocalcemia, hypomagnesemia, polycythemia, and adverse fetal programming, contributing to long-term risks including childhood obesity, T2DM, hypertension, and CVD in adolescence and adulthood
[17][18][19]. Children of mothers with GDM or pregnant women with preexisting diabetes of any type (T1DM, T2DM, MODY, LADA, etc.) have an eight-times-higher risk of developing prediabetes or diabetes, compared to children of non-diabetic mothers
[18].
2. Pregnancy
2.1. Gestational IR
Insulin resistance is commonly observed during pregnancy, although the processes underlying its pathogenesis are complex and not yet fully understood
[20]. In a typical pregnancy, maternal tissues gradually become more insensitive to insulin, resulting in a 50–60% decrease in insulin sensitivity as gestation progresses
[21][22]. During the second half of pregnancy, when IR is at its peak, GDM is believed to develop when β-cells fail to adapt to the increasing demand for insulin
[21]. Both women with and without GDM experience similar increases in insulin production during pregnancy; however, women with GDM start at a lower level. Consequently, the β-cell abnormality in GDM is considered more of a chronic condition than a development during pregnancy. Overt T2DM may develop postpartum as a result of this β-cell malfunction in GDM
[23]. Prior to and independently of changes in insulin sensitivity, the insulin secretory response significantly increases in the early stages of pregnancy. This metabolic adaptation may be mediated by circulating hormones, with placental hormones and/or cytokines likely responsible for these changes in maternal physiology
[24].
Mostly women with preexisting IR and predisposing risk factors develop GDM. These risk factors include PCOS, low- or high-birth-weight fetuses, a family history of T2DM or GDM, multiparity, advanced maternal age, and a prior GDM diagnosis
[25]. However, women with no preexisting IR may also develop GDM because they have defective β-cell function due to genetic or idiopathic reasons. IR in early pregnancy and a positive glucose challenge test in later pregnancy, irrespective of BMI, were also found to be associated with visceral adipose tissue depth evaluated by ultrasound that exceeded the top quartile in early pregnancy
[26].
2.1.1. Gestational Weight Status
Maternal weight is a crucial factor that significantly influences pregnancy progression, in conjunction with maternal age. Extensive studies have examined weight, calculating BMI in early pregnancy and the percentage of weight gained during pregnancy. Being overweight and obese are established contributors to GDM. A high antenatal BMI and excessive weight gain during pregnancy are recognized risk factors for various complications, including postpartum weight retention, GDM in subsequent pregnancies, future obesity, T2DM, and long-term CVD
[27][28]. Additionally, these factors contribute to obstetrical complications such as pre-eclampsia, eclampsia, macrosomia, hemorrhage, and cesarean delivery
[29].
Regarding maternal body fat levels, a significant correlation with maternal leptin levels during pregnancy has been observed. Leptin, a hormone produced by adipose tissue, has various metabolic effects, including decreased insulin sensitivity in non-pregnant individuals
[30]. Mothers with elevated body fat levels are also more likely to give birth to newborns who have a higher likelihood of being obese in adulthood
[31]. Visceral adipose tissue (VAT) in the abdomen is associated with metabolic syndrome, IR, and an elevated risk of CVD in the future
[32][33].
The detection of IR and glucose dysregulation in mid-pregnancy is correlated with an increased depth of VAT in the first trimester of pregnancy
[34][35].
2.1.2. Effects of Diet on Gestational IR
Diet, encompassing total calorie consumption, dietary plans, food processing, and preparation, as well as exposure to endocrine-disrupting chemicals
[36][37], appears to exert a significant impact on the onset or exacerbation of IR, particularly in cases where IR already exists, such as in obesity or PCOS
[38].
A high-fat, Western-style diet characterized by a high caloric intake is a major risk factor for developing IR, prediabetes, T2DM, and obesity
[39]. A diet rich in fats or sugars may also significantly alter the diversity of intestinal microbial flora. However, most diet-related alterations to the gut microbiota appear to be reversible with appropriate dietary modifications
[40].
The diet recommended for expectant women with GDM should aim to promote healthy maternal weight gain while minimizing postprandial glucose spikes and fostering fetal development. Various dietary recommendations for GDM have been compared in numerous studies, including energy-restricted versus unrestricted diets; low-CHO, -GI, and -GL diets versus high-CHO, -GI, and -GL diets; diets rich in monounsaturated fats versus high-CHO diets
[41]; and conventional diets versus fiber-enriched diets.
2.1.3. Effects of CHO and GI/GL Estimates on Gestational IR
Women with gestational GDM should exercise extra caution regarding both the quantity and type of CHOs they consume. All pregnant women require a minimum of 175 g of dietary CHOs, 71 g of protein, and 28 g of fiber daily, as CHOs serve as a vital energy source for both the mother and the fetus
[42]. The nutrition plan should highlight monounsaturated and polyunsaturated fats while limiting saturated fats and avoiding trans fats
[19].
A low-CHO diet is typically recommended for women with GDM, despite inconsistent findings in several studies
[43]. Especially for women with T1DM, optimal metabolic regulation and more successful pregnancies seem to be achieved through CHO counting
[44]. A moderately low-CHO diet comprising 40% of the recommended daily calories improves glycemic management in women with T2DM but does not demonstrate beneficial effects on pregnancy outcomes
[45]. While a low-CHO diet improves short-term glycemic control in women with GDM, no impact on insulin requirements (in women receiving insulin treatment) or the success of pregnancies has been observed
[24][46]. However, caution is advised when combining a low-CHO dietary pattern with a high consumption of animal-based protein and fat, as it appears to be associated with a higher risk of GDM and T2DM later in life
[46][47].
A high GL diet has been associated with an increased risk of GDM in women, particularly when compared to those with the lowest tertile of dietary GL
[48]. Younger gestational ages, higher CHO proportions, and lower fiber intake were strongly linked to high-GL diets
[17].
Diets with a low GI and GL, such as the DASH diet and the Mediterranean diet, have demonstrated positive effects on various biochemical and health parameters
[49][50][51]. Regarding obstetric and fetal outcomes in GDM patients, lower-GI diets have demonstrated potential benefits, although the findings are still debatable
[48]. Notably, lower insulin utilization was observed in individuals adhering to a low-GI diet, characterized by the consumption of high-quality, complex CHOs
[16][52][53].
Examining the impact on offspring, low-GI diets were associated with a decreased incidence of large-for-gestational-age (LGA) babies
[53], as well as influences on birth length and early childhood arterial wall thickness
[54]. The Homeostatic Model Assessment for Insulin Resistance (HOMA-IR), insulin, and leptin levels in children were also significantly were also significantly and positively correlatedwith dietary GI during pregnancy
[55].
Contrary to these findings, several studies suggested that GI and GL indices were not significantly associated with GDM risk
[56][57]. In patients with T2DM, Ojo et al. reported no notable differences in total cholesterol, HDL cholesterol, or LDL cholesterol
[58]. Additionally, no distinctions were found in lipids
[16][34], fructosamine, glycosylated hemoglobin
[16][34], overall glycemic control, or pregnancy outcomes in women with GDM.
2.2. Inflammation in Gestation
Low-grade inflammation induced by cytokines is a typical feature of pregnancy, playing a vital role in the finely controlled inflammatory response crucial for the development of placentation from implantation to labor
[59]. Maternal obesity and GDM are strong risk factors for persistent low-grade inflammation.
Proinflammatory cytokines have been consistently linked to inflammation induced by obesity, showing higher levels in affected individuals
[55]. In pregnancies affected by obesity, the placenta may undergo changes in shape and function as an adaptive response, acting as both a target and a source of inflammatory cytokines
[59]. Adipose tissue also produces several inflammatory factors that regulate hunger and fat synthesis. These pro-inflammatory mediators play a role in the development of IR, overt diabetes mellitus, and other complications related to obesity
[60][61][62][63].
Regardless of maternal BMI, specific inflammatory markers have been found elevated during pregnancy in women with a history of GDM and those who later develop GDM. Circulating tumor necrosis factor-alpha (TNF-α), as a biomarker of inflammation, decreases in early pregnancy, accompanied by an increase in insulin sensitivity
[64]. In a study by Challier et al., obese women exhibited a 2–3-fold increase in placental macrophages along with elevated mRNA expressions of interleukin-1 (IL-1), TNF-α, and interleukin-6 (IL-6)
[65]. While lean, overweight, and obese women show similar patterns of cytokine changes, those with a higher BMI tend to exhibit an increase in specific inflammatory markers (CRP and IL-6), although not consistently across all markers
[59]. Serum C-reactive protein (CRP) levels during the late second and early third trimesters are associated with GDM and weight gain, whereas elevated highly sensitive CRP levels in GDM patients may indicate an increased risk of later developing T2DM
[66]。
3. Postpartum Period and Long-Term Management
Following delivery, women with GDM who were not previously diagnosed as diabetic (with any type of DM, including T1DM, T2DM, MODY, and LADA) should discontinue any treatment if needed. The recommended timeframe for glycemic reassessment is six to thirteen weeks post-delivery
[67], to decide if initiation of drug treatment is indicated. This is particularly advised if future pregnancies are planned.
Healthcare providers should educate patients about the long-term risks of both T2DM and GDM during the postpartum period. Both the American Diabetes Association (ADA)
[68] and the National Institute for Health and Care Excellence (NICE)
[69] recommend lifelong, annual glucose level evaluations. Various risk factors, such as family history, a history of GDM in previous pregnancies, and the need for insulin or oral glucose-lowering medications during pregnancy, influence the frequency of T2DM occurrence
[67].
Lifestyle modifications play a crucial role, especially for morbidly obese women with IR, prior GDM, or prediabetes. A diet rich in protein and regular exercise are strongly recommended, as they have proven to be more successful in reducing IR and improving glycemic variability
[70]. Diets such as the Mediterranean and DASH diets, low in complex CHOs and GI/GL, particularly when combined with plant-based proteins and fats
[47][71], are employed to reduce the likelihood of GDM in future pregnancies and the risk of developing T2DM.
4. Conclusions
The use of GI and GL estimates in the management of GDM has gained attention, recognizing the substantial impact of diet on glycemic control and pregnancy outcomes for GDM patients. Research indicates that low-CHO diets, particularly in the short term, have proven beneficial for patients with T2DM. Considering that women with GDM typically have diabetes for short durations (less than six months), low-CHO/low-GI diets may also be advantageous for them. A low-GI diet, in contrast to one with a higher CHO content, has been associated with improved glycemic control, reduced insulin requirements, lower cholesterol levels, decreased inflammation markers, and enhanced obstetric outcomes.
However, the data remain contradictory, with several studies reporting no significant associations. The limited number of studies examining the impact of GI and GL on GDM, the absence of a consensus on what constitutes a preferred GL/GI diet, and ethical constraints preventing the study of effects on infants and newborns except through observational studies hinder our ability to fully assess these diets’ effects. Until larger-scale intervention trials are conducted, a low-GI diet should not replace the current pregnancy diets recommended by health organizations. Achieving a general consensus on what constitutes a low-GI/-GL diet is essential to enhance clinical practice with specific dietary recommendations for GDM patients.
This entry is adapted from the peer-reviewed paper 10.3390/nu16030399