The original TRP channel was found in
Drosophila photoreceptors. It contributes to sustained retinal depolarization when exposed to light. Deletion of the trp gene results in a transient calcium response, which lends its gene name. Unlike in
Drosophila, mammalian TRPs serve a wider range of physiological functions
[3][4]. In general, TRPs enable cells to detect subtle alterations in the local environment (e.g., fluid shear stress and mechanical forces), perceiving and responding to a variety of external cues through varied sensory perceptions, including vision, smell, taste, hearing, thermosensation, and most importantly, mechanosensation
[5]. They are a class of ion channels found in diverse tissues and cell types, widely distributed in human organs, such as the liver, lungs, skin, kidneys, nerves, and intestines. They are permeable to a broad range of cations such as Ca
2+, Mg
2+, K
+, Na
+, and others
[6]. TRP family members are categorized into two main groups, based on their sequence topology and phylogeny. Group 1 consists of TRPC (canonical), TRPV (vanilloid), TRPM (melastatin), TRPN (nompC), and TRPA (ankyrin), whereas group 2 includes TRPP (polycystin) and TRPML (mucolipin), totaling seven subfamilies
[7]. Group 1 TRPs share substantial homology across their transmembrane domain sequence. Most of the group 1 TRP proteins are characterized by a C-terminal TRP domain, composed of 23 to 25 amino acids and located near the sixth transmembrane domain. In contrast, group 2 TRPs feature low sequence similarity with group 1, and an extracellular loop that is interposed between the first two transmembrane domains
[2][5].
2. Function of TRPV4-Evoked Ca2+ Oscillations in Injury Repair and Fibrosis
TRPV4 mechanosensor is an integral factor in the progression of tissue repair and fibrotic disorders across different organs, including the skin, lungs, liver, kidneys, brain, blood vessels and heart
[13]. In this section, the researchers provide a comprehensive summary of the current understanding regarding the functions of TRPV4-induced Ca
2+ oscillation in each specific organ (
Figure 1). It is noteworthy that the mechanisms underlying cytosolic calcium oscillations in excitable and non-excitable cells significantly differ in the context of TRPV4 activation. Essential details, including whether the cells are excitable or non-excitable.
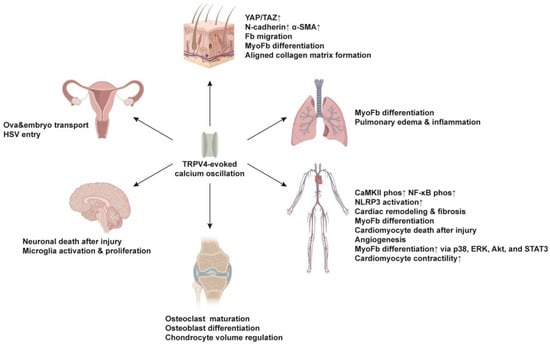
Figure 1. Functions of TRPV4-induced Ca2+ oscillations across different organs. TRPV4 is essential for physiological activities in the skin, lung, cardiovascular, skeletal, nervous and reproductive systems. Fb, fibroblast; MyoFb, myofibroblast; Phos, phosphorylation; HSV, Herpes simplex virus. Upward arrows indicate higher expression or activity.
2.1. Skin
In a bleomycin-induced skin fibrosis model, increasing matrix stiffness was found to activate TRPV4, with TRPV4 being essential for the nuclear translocation of YAP/TAZ in response to matrix stiffness. Genetic ablation of TRPV4 (
Trpv4-knockout) or pharmacological inhibition of TRPV4 could ameliorate this fibrotic response. The authors attributed the effect of TRPV4 to its role in regulating the process of epithelial-mesenchymal transition
[14]. In this study, it was also found that TRPV4-induced Ca
2+ oscillations led to higher expression of N-cadherin and α-SMA on skin fibroblasts
[14]. The researchers' group has recently revealed that N-cadherin
[15]—together with Connexin-43
[16] and p120-catenin
[17]—are critical intercellular adhesion molecules that coordinate the collective migration of fibroblasts at a supracellular level needed for wound repair and subsequent scar formation. Therefore, in the skin fibrosis model, TRPV4′s effect on fibrosis development could also be attributed to its induction of fibroblasts’ collective migration and cell state transition toward myofibroblasts. However, the detailed signaling components and routes between TRPV4-induced Ca
2+ oscillations and YAP/TAZ nuclear translocation, and the role of Ca
2+ oscillations in synchronizing collective fibroblast migrations are yet to be determined. Of note, TRPV4-induced Ca
2+ oscillations seem to be involved in adipogenic differentiation. Knocking down
TRPV4 using shRNA significantly reduced adipogenesis in human preadipocytes via reduced phosphorylation of Akt kinase
[18].
In addition, TRPV4-mediated Ca
2+ oscillations are also implicated in matrix deposition. Gilchrist et al. found that the TRPV4 agonist GSK1016790A induced Ca
2+ oscillation in mesenchymal stem cells at low concentrations (1, 10 nM), and is required for collagen matrix assembly in an aligned manner
[19]. Interestingly, at a higher concentration (100 nM), the agonist initially activated TRPV4 and yielded a strong Ca
2+ influx, but failed to sustain a rhythmic Ca
2+ oscillation and regulate the formation of aligned collagen matrix
[19].
TRPV4 is expressed in the hair follicles during the growing anagen phase. More specifically, it can be detected mainly in the outer and inner root sheath layers of the hair follicle epithelium, particularly in keratinocytes. The activation of TRPV4 through its agonist GSK1016790A was found to cause a significant reduction in hair shaft elongation and induce morphological and structural changes in anagen hair follicles, pushing them toward the catagen phase
[20]. This study indicates that TRPV4-induced Ca
2+ influx promotes injury repair and fibrotic processes, yet it may also suppress hair follicle regeneration after wounding. Therefore, targeting TRPV4 could either facilitate skin wound healing by enhancing Ca
2+ influx, or stimulate hair follicle regeneration and reduce scarring by blocking its effects.
2.2. Lung
In human pulmonary fibroblasts, activation of TRPV4 assists cell membrane depolarization. When the membrane potential is in the voltage range of voltage-dependent Ca
2+ current, the activated Ca
2+ influx contributes to Ca
2+ oscillations
[21]. TRPV4 has also been reported to mediate TGF-β evoked repetitive Ca
2+ waves in human lung fibroblasts, leading to the upregulation of ECM genes encoding collagen and fibronectin, and increased phosphorylation of SMAD-2 protein
[22]. Via the P2Y receptor, ATP is able to release internal Ca
2+ through ryanodine-insensitive channels, thereby exerting its effect on Ca
2+ waves
[23].
TRPV4 activity has been found to be significantly higher in patients with idiopathic pulmonary fibrosis. Activation of TRPV4 in lung fibroblasts accelerates the differentiation of myofibroblasts and contributes to the progression of pulmonary fibrosis
[24]. In this context, it is worth mentioning that TRPV4 expression on murine lung fibroblasts is necessary for TGF-β-induced myofibroblast differentiation. Furthermore, TRPV4 mechanotransduction increases on stiffer matrices or in fibrotic lung tissue, while
Trpv4-deficient mice are protected from bleomycin-induced lung fibrosis
[24][25]. These findings imply that TRPV4 antagonists may represent potential targets for pulmonary fibrosis disease.
While TRPV4 activation promotes skin wound repair, its activity in lung endothelial cells has been shown to be detrimental in the setting of lung ischemia-reperfusion (IR) injury. In fact, TRPV4 activation in lung endothelial cells increases vascular permeability, thus causing increased lung inflammation and edema after IR
[26]. Genetic depletion of TRPV4 using
Trpv4−/− mice or pharmacological inactivation of TRPV4 with the TRPV4-specific inhibitor GSK2193874a markedly reduced pulmonary edema and neutrophil infiltration after IR injury, when compared to untreated wild-type mice. To further investigate TRPV4′s role in endothelial cells, Ottolini et al. generated an inducible endothelial
Trpv4 knockout line by crossing
Trpv4 floxed mice (
Trpv4fl/fl) with tamoxifen-inducible VE-Cadherin (
Cdh5) Cre mice
[27]. The endothelial-specific
Trpv4 knockout exhibited the same phenotype as observed in the whole-body
Trpv4 knockout, suggesting that TRPV4 activity in endothelial cells contributes to pulmonary dysfunction and edema following IR injury. In line with the IR injury model, inhibition of TRPV4 had a protective effect in a mouse model of resistive breathing (RB)-induced lung injury. During RB, increased mechanical stress is imposed on the lung, thus leading to lung injury. The administration of the TRPV4 antagonist HC-067047 significantly mitigated symptoms by restoring static compliance and ameliorating the lung inflammation by reducing the number of macrophages and neutrophils, and the inflammatory chemokines keratinocyte-chemoattractant (KC) and interleukin (IL)-6 in bronchoalveolar lavage
[28].
2.3. Cardiovascular System
The role of TRPV4 in cardiac remodeling has been investigated in the context of myocardial infarction and subsequent fibrosis, with a focus on TRPV4 channels expressed in non-excitable cardiac fibroblasts. Experimental findings demonstrate that while hypotonicity induces significant Ca
2+ influx in cardiac fibroblasts from wild-type mice, this response is markedly diminished in cardiac fibroblasts derived from
Trpv4-knockout mice, thereby establishing TRPV4′s role as a mechanosensor in fibroblasts
[29]. In vitro studies using rat or human cardiac fibroblasts have revealed that TRPV4 activation by specific agonists such as 4αPDD or GSK1016790A leads to Ca
2+ influx, which subsequently promotes the differentiation of fibroblasts into myofibroblasts through the p38 and ERK MAPK pathways
[30][31]. Consistently, the blockade of TRPV4 by different antagonists or
Trpv4-siRNA inhibits TGFβ-induced myofibroblast transformation by inhibiting the p38, Akt, and STAT3 signaling pathways
[32][33]. In addition, it has been noticed that TRPV4 protein expression increases in cardiomyocytes of the aged heart. TRPV4-evoked calcium oscillations are directly correlated to the contractility of cardiomyocytes and contribute to tissue damage in the aged heart following ischemia-reperfusion (I/R), a pathological condition associated with cardiomyocyte osmotic stress. TRPV4 antagonist HC-067047 is effective in preventing Ca
2+ overload in cardiomyocytes after I/R and prevents hypoosmotic stress-induced cardiomyocyte death and I/R-induced cardiac damage in the elderly population
[34][35]. The same observation is documented in the aged
Trpv4 knockout mice
[36]. Furthermore, TRPV4-induced Ca
2+ oscillation has been reported in human cardiac c-kit
+ progenitor cells, regulating their migration but not proliferation
[37]. In endothelial colony-forming cells, TRPV4-mediated Ca
2+ oscillation results in the nuclear translocation of a Ca
2+-sensitive transcription factor p65 NF-κB and induces angiogenesis
[38].
In addition, TRPV4 channel expression is found to be significantly increased in failing human ventricles, and in murine ventricles using a mouse model of pressure overload-induced cardiac hypertrophy
[39]. Compared to wild-type mice,
Trpv4 knockout mice showed a remarkable reduction in cardiac hypertrophy, cardiac dysfunction, fibrosis, and inflammation. Those findings imply that TRPV4 is involved in cardiac remodeling and fibrosis after injury. Moreover, the absence of TRPV4 prevented an elevation of CaMKII phosphorylation in excitable cardiomyocytes of a pressure-overloaded left ventricle. It has been proposed that the TRPV4-mediated increase in CaMKII phosphorylation triggers NF-κB phosphorylation and NLRP3 activation, both of which contribute to the pro-inflammatory remodeling observed in these pressure-overloaded hearts
[39].
In the vascular system, TRPV4 expression is widely reported in vascular smooth muscle cells (VSMCs), and TRPV4 plays an important role in the regulation of blood pressure and the development of hypertension. In patients with hypertension and in mouse models of hypertension, VSMCs showed elevated TRPV4 activity, resulting in signal amplification for the simulation of α1 adrenergic receptor. Strikingly, VSMC-specific
Trpv4-knockout mice were protected from hypertension
[40]. TRPV4 expression in VSMCs also contributed to vascular remodeling after injury, whereby TRPV4 was activated by platelet-derived microvesicles and triggered Ca
2+ influx from the ECM and subsequent Ca
2+ oscillations, thus contributing to the modulation of the cell migration
[41].
2.4. Skeletal System
A mounting body of evidence indicates that TRPV4 is required for the normal development and maintenance of bone and cartilage. Osteoclasts are considered the main mediators of bone resorption. Their activity in removing damaged tissue and modulating the bone microenvironment is crucial for subsequent phases of bone healing and the restoration of bone structure and function. The maturation and function of osteoclasts are regulated by intracellular Ca
2+ oscillatory signals, which are stimulated by mechanical forces, such as fluid shear stress. TRPV4 was found to be highly expressed in mature osteoclasts and necessary for Ca
2+ oscillation induced by fluid shear stress
[42]. Ca
2+ oscillation affected the bone homeostasis-related gene expression, including sclerostin (
Sost), osterix (
Sp7), and osteoprotegerin (
Tnfrsf11b)
[43], and osteoblastic differentiation genes, such as alkaline phosphatase (
Alpl), osterix (
Sp7), dentin matrix protein 1 (
Dmp1), and osteocalcin (
Bglap)
[44]. Spontaneous Ca
2+ oscillation was generated by intracellular Ca
2+ release as well as Ca
2+ reuptake, and sustained by Ca
2+ influx. It was gradually shifted to Ca
2+ influx through TRPV4 during osteoclast differentiation, so that Ca
2+ oscillations and Ca
2+ influx via TRPV4 were sequentially required
[45]. Upon exposure to an electromagnetic field, TRPV4 expression was downregulated in osteoclasts, and Ca
2+ oscillations were impaired as a result of Ca
2+ influx decrease, culminating in reduced activity of the calcium/calmodulin-dependent protein kinase–cyclic AMP response element-binding protein (CaMK–CREB) pathway
[46].
In chondrocytes, intracellular Ca
2+ oscillations are one of the earliest responses elicited by physical stimuli, such as compressive loading and stretch
[47][48]. TRPV4 serves as a major mechanical sensor on chondrocytes by regulating Ca
2+ signaling, together with Piezo1 and Piezo2 channels
[49]. Evoked Ca
2+ oscillation regulates chondrocyte volume in response to the pericellular matrix
[50]. In stiff substrates, cytosolic Ca
2+ oscillations were enhanced in the cell recovering phase, whereas in soft substrates, the cytosolic Ca
2+ oscillations were increased in the cell swelling process. Of note, heterozygous mutations in
TRPV4 led to altered chondrocyte Ca
2+ oscillation and caused severe metatropic dysplasia
[48].
Intracellular Ca
2+ oscillation and a sustained Ca
2+ response have been documented in annulus fibrosus cells, which form one of the structural components of intervertebral discs. This suggests that TRPV4 is a crucial regulator of the perception of mechanical stimulation in the intervertebral discs of the spine
[51]. Furthermore, TRPV4 is also implicated in joint inflammation. Both TRPV4 and TRPV1 are activated in synovial cells by protein kinase A and protein kinase C, leading to increased cell sensitivity to noxious thermal changes and hypoosmotic stress
[52][53]. In contrast, in a mouse model of osteoarthritis, knockout
Trpv4 in articular chondrocytes (
Col2a1CreER;
Trpv4fl/fl) induced more severe age-related osteoarthritis
[54].
2.5. Nervous System
TRPV4 is expressed in microglia, a type of non-excitable cells within the central nervous system (CNS), and plays a role in microglia activation and proliferation, promoting functional and structural plasticity in excitatory spinal cord neurons through the release of lipocalin-2
[55]. Genetic ablation of
Trpv4 in microglia using
Cx3cr1CreER;
Trpv4fl/fl mice or a pharmacological blockade of TRPV4 with TRPV4 antagonist GSK2193874 markedly attenuated neuropathic pain-like behavior in a mouse model of spared nerve injury
[55], highlighting TRPV4 as a potential target for chronic pain treatment.
The functional involvement of TRPV4 in excitable neurons in the spinal cord remains a subject of controversy. Previous studies have reported TRPV4 expression in spinal dorsal horn neurons, ventral horn neurons, and dorsal root ganglion in Sprague-Dawley rats and neonatal mice, indicating a direct influence on the induction of neuropathic pain
[56][57][58]. In contrast, recent work by Hu and colleagues challenges this perspective, asserting that TRPV4 is not expressed in NeuN
+ spinal cord neurons but rather in IBA1
+/Tmem119
+ microglia and CD31
+ endothelial cells, as revealed by genetic lineage tracing in mice. This suggests that TRPV4 might participate in pain signaling through sustained neurogenic inflammation
[55].
TRPV4 also plays a crucial role in neuronal damage during cerebral ischemia. In a rat model of hypoxia/ischemia-induced cerebral injury, the expression of TRPV4 is markedly enhanced in hippocampal astrocytes. The increased activity of TRPV4 channels triggers Ca
2+ oscillations in these cells, promoting active cellular proliferation that protects neurons, initiates repair, and correlates with astrogliosis—scar formation in the brain
[59]. TRPV4 expression undergoes upregulation in the ipsilateral hippocampus following middle cerebral artery occlusion, contributing to neuronal injury during cerebral ischemia. The neurotoxic effects associated with TRPV4 activation coincide with an elevation in the phosphorylation of the NR2B subunit of the N-methyl-D-aspartate receptor (NMDAR) and a concurrent downregulation of the Akt signaling pathway. Consequently, the TRPV4-specific antagonist HC-067047 has proven effective in reducing cerebral infarction. Conversely, intracerebroventricular injection of the TRPV4 agonist GSK1016790A0 has been shown to induce hippocampal neuronal death
[60]. In
Trpv4-knockout mice, impaired osmotic sensing and regulation in the CNS have been observed. In this context, TRPV4-induced Ca
2+ oscillations, which trigger c-FOS expression in osmotically responsive cells of the CNS, become dysfunctional
[61].
Moreover, rapid Ca
2+ oscillations are recorded in the subpial endfeet of astrocytes, with oscillatory activities relying on the IP
3R type 2 channel correlating with TRPV4 and dystrophin. Astrocytes leveraged the Ca
2+ response to effectively respond to hypoosmotic changes in the cerebrospinal fluid
[62]. However, this particular function is lost after subarachnoid hemorrhage
[63].
2.6. Reproductive System
TRPV4, in conjunction with γ-aminobutyric acid receptors A and B (GABA A/B), has been identified as a contributor to progesterone (P4)-induced ova and embryo transport. In the mouse oviduct, Ca
2+ oscillation and an increase in ciliary beat frequency are seen in response to P4 and GABA A/B activation. Importantly, TRPV4-mediated Ca
2+ entry is essential for the initiation and maintenance of this oscillatory signal
[64]. In addition, during infection in vaginal epithelial cells, Ca
2+ fluctuations seem to play a critical role in facilitating the entry of Herpes simplex virus (HSV). TRPV4 expressed in human epithelial cells mediates the influx of Ca
2+. Inhibition of TRPV4 by TRPV4 inhibitors, such as GSK2193874 and HC067047, not only reduces HSV-2 infection in human vaginal epithelial cells, but also attenuates the associated inflammatory responses, characterized by the expression of tumor necrosis factor (TNF)-α, IL-6, C-X-C motif chemokine ligand (CXCL)-9, and CXCL-10
[65]. These findings underscore the potential of TRPV4 as a target for therapeutic interventions related to reproductive health and HSV-2 infection.