1. Introduction
Solar radio bursts provide a unique diagnostic of the development of flare/CME events in the low corona (see for example,
[1][2][3][4]); their onset and evolution coincides with an extended opening of the magnetic field and/or solar flare reconnection
[5] accompanied by energetic-particle acceleration and injection into interplanetary space as well as shocks (e.g., reviews by
[6][7]). Their signatures in microwave, decimetric, and metric and longer waves trace disturbances propagating from the base of the corona to interplanetary space. The radio signatures associated with the onset of these energetic phenomena on the Sun constitute potential radio precursors of space weather disturbances (e.g.,
[8][9][10]), including Solar Energetic Particles (SEPs).
Often the radio bursts encase groups of smaller bursts with different time scales, referred to as fine structure (FS). Our understanding of metric radio burst fine structure continuously improves with the advent of radio data with high time and frequency resolution. As the radio bursts provide readily available diagnostics of the development of energetic phenomena of the Sun, their fine structure may provide the details. To this end, high resolution dynamic spectra are required. Furthermore, radio imaging data supplementing the corresponding dynamic spectra are equally necessary.
The complexity of the processes associated with the flare/CME event development is reflected in a diversity of forms in dynamic spectra, which exhibit a variety of fine structures in time and frequency; these are characterized by a wide range in period, bandwidth, amplitude, temporal, and spatial signatures (See review by Chernov
[11]). The fine structures may be used for the detailed study of the magnetic field restructuring and the corresponding energy release associated with solar flare/CME events (e.g., reviews by
[12][13]). A number of morphological taxonomy schemes, mostly in the microwave and the decimetric frequency range, have been presented
[14][15][16][17][18][19][20], of which the most recent are also the most comprehensive. In the metric wavelength range, Bouratzis et al.
[21] proposed a morphological classification consistent with the works mentioned in this paragraph; in their work, fine structures were divided in five basic classes with two or more subclasses each, thus providing a more structured approach.
2. Fine-Structure Classification in the Metric Wavelength Range
The criteria used in the development a fine-structure classification scheme were based on the morphology of the fine structure on dynamic spectra from the ARTEMIS-IV/JLS/SAO receiver
[22]. The basic data set was in the metric wavelength range, yet we have strived for a flexible scheme capable to accommodate different fine structure frequency bands, including the highly complex structures of the microwave range. The results are consistent with the Ondrejov catalogue
[19], which was based on data in the 0.8–2.0 GHz range. However, at variance with previous attempts, researchers opted for a more structured approach adopting two-level classification. The fine structures were thus allocated into five basic classes, corresponding to coarse-grained spectral features. Each basic class was further divided in two or more subclasses based on the finer details of the spectral features
[21]. This two-level hierarchical arrangement serves the diversity of the microwave–decimetric bursts which exceeds that of their counterparts in the meter range and below
[23]. Details of the two-level scheme are given in the following subsections. In the classification process, statistical results were also obtained regarding the association of the various types with the various phases of the flare/CME phenomenon, which could be useful in understanding details of the evolution of the solar energetic phenomena through their radio signatures.
2.1. Featureless Broadband or Diffuse Continuum
This class includes both diffuse segments of type-IV bursts and radio emissions of smaller bandwidth and duration, such as slowly drifting bursts and patches. Intensity variations within the smooth periods of the recorded type-IV bursts might qualify as patches too. In Bouratzis et al.
[21], long periods of broadband continuum were found to overlap in time with the SXR emission and with embedded intermittent periods of the fine structure mentioned in the subsections below. The smooth periods devoid of fine structure varied between 0% and 60% of the type-IV continuum duration; the relatively longer smooth periods were found to increase with the duration of the type-IV burst. Although periods of featureless diffuse continuum have been recorded in abundance in the past, data suggest that fine structure may still be detected at sufficiently high resolution and sensitivity.
2.2. Pulsating Structures (Pulsations)
The dynamic spectra pulsating structures appear either as drifting or stationary (see
[13] for a review); the shortest groups of these structures with durations ∼10 s are known as isolated broadband pulses in
[19]. They are considered as the result of quasi-periodic acceleration episodes in reconnecting current sheets
[5]. The ensuing kinetic plasma instabilities and the corresponding energetic electron populations are thought to produce the characteristic radio signature which are known as quasi-periodic pulsations (QPPs). In the microwave frequency range (0.8–2.0 GHz), drifting QPPs are thought to be among the possible flare radio precursors as they were found to precede the flare onset by ∼1–4 min .
2.3. Narrow-Band Bursts
The signatures of small-scale acceleration episodes (see review by Nindos et al.
[7]) manifest themselves as narrow-band bursts of the type-III family: spikes, dots, and subsecond patches , depending on their shape in the dynamic spectra. Part of the same family are the III(U) and III(J) narrow-band bursts
[20][24] and the supershort structures (SSSs) found embedded in the metric solar type-IV continua
[25]. The high time-resolution of the SAO receiver made the morphological distinction possible between a large number of different narrow-band structures of the ARTEMIS-IV/JLS data set, such as narrow-band J or U bursts, and the unusual inverted U burst. The duration and bandwidth distributions, however, did not show any clear separation in groups. The narrow-band bursts occasionally comprise the “superfine structure” (or “hypefine structure”) of various type of bursts. Examples of radio bursts formed from spike chains are type-III bursts in the 1–2 GHz range , pulsating structures in the 2.6–2.8 GHz range , etc. Furthermore, the narrow-band bursts are part of both the type-II and the type-IV-associated fine structure. Note that type-I bursts, superposed on type-I continua (noise storms) at metric frequencies
[26], also have small duration and bandwidth and spike-like dynamic spectra. Their duration and bandwidth is close to the type-II-associated spike-like bursts and the type-IV-associated spikes observed in the same frequency range
[27]; high-resolution observations by Iwai et al. corroborate this similarity.
2.4. Intermediate-Drift Bursts
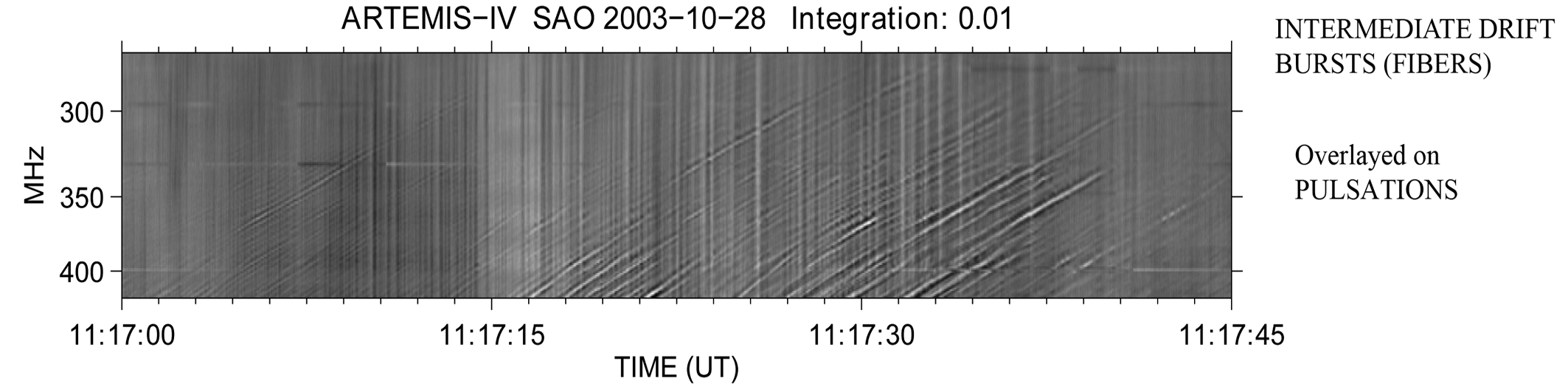
The intermediate-drift bursts are characterized by their drifting stripe-like form, whose drift rate exceeds that of the type-II bursts but does not reach the type-IIIs and their absorbtion ridge. They include the typical fibers
[28][29][30][31], the narrow-band rope-like fibers
[32][33][34][35], the narrow-band fibers, and the fast drift fibers (FDBs). The fiber bursts are mainly detected drifting along coronal magnetic structures and, in particular, postflare loops. In the metric wavelengths, they usually appear some minutes after the first energy release marked by the first HXR or microwave peak (see, for example
[21], Figure 9). The intermediate-drift burst radio emission has been interpreted as the result of whistler–Langmuir
[29][30] or Alfvén–Langmuir wave interaction , based on their characteristic drifting stripes in emission and absorption; they provide, thus, a valuable radio–diagnostic of Solar Coronal Magnetic Fields
[36][37]. The theory of whistler (or Alfvén) origin stipulates a three-wave coupling process (
𝑙+𝑤→𝑡 or
𝑙+𝐴→𝑡), where whistlers (w) or Alfvén (A) coalesce with the Langmuir waves (l) of the type-IV background, resulting in transverse wave emission (t). The three wave interaction (
𝑙+𝑤→𝑡 or
𝑙+𝐴→𝑡) depletes the background Langmuir wave energy, resulting in the absorption ridge at the the plasma frequency (low-frequency side of the fiber) and produces enhanced emission at the sum of the frequencies of the interacting waves. A different approach was presented recently by Ni et al.
[38], who considered the production of fundamental electromagnetic radiation (ordinary mode, O) at the plasma frequency induced by the resonant coupling of Z-mode and whistler (w) waves (
𝑍+𝑤→𝑂); this might offer an alternative IDB interpretation.
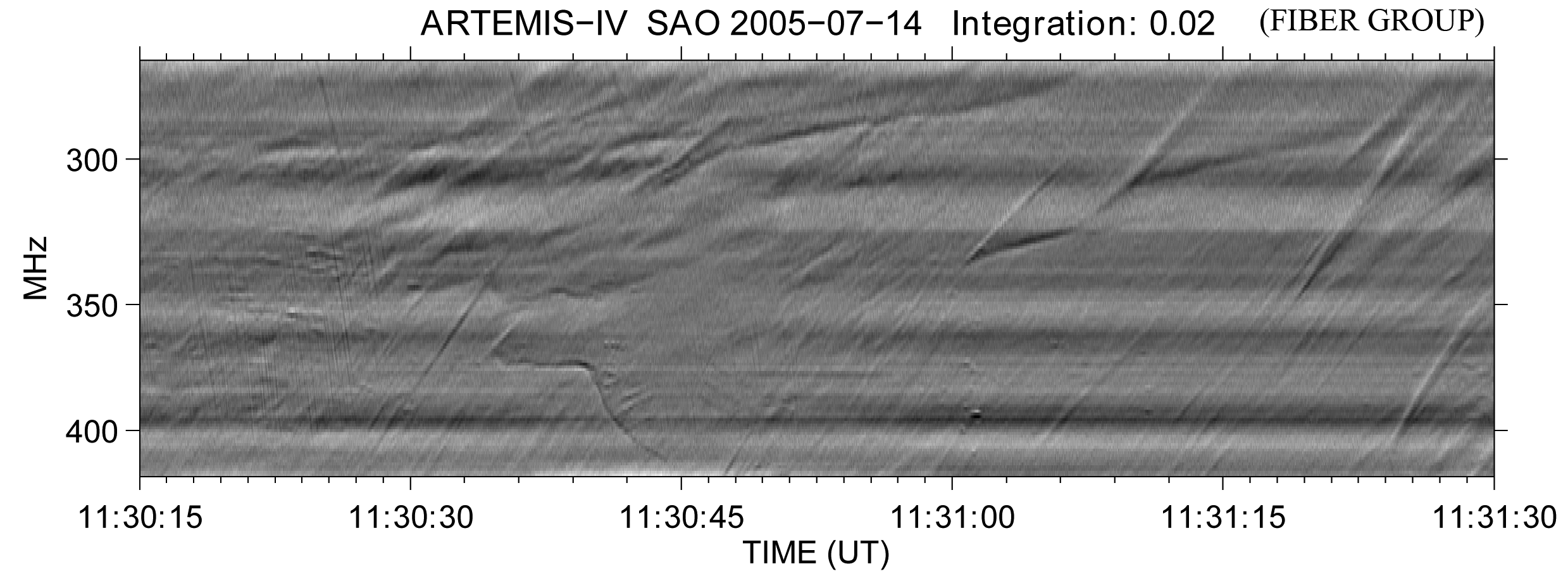

2.5. Emission Bands
The zebra family is the the emission bands and it shows significant diversity, including the classic or pulsation-superposed zebra patterns, the fiber-associated zebras
[34], and the zebras with a drifting emission envelope . The corresponding emission mechanism interpretations vary from double plasma resonance
[39][40], Bernstein modes, or plasma waves trapped in resonators, and more (see Nindos et al.
[7]). The rare lace-burst, first reported by Karlický et al.
[41], is also member of the zebra family; its emission has been attributed to the double plasma resonance
[42], and observational evidence in support of this interpretation has been provided by Chen et al.
[43]. The zebra bursts exhibit stripes in emission and absorption comparable to the fibers, and hence they provide an additional radio–diagnostic for Solar Coronal Magnetic Fields
[36][37].
Some uncommon band structures from the metric–decimetric frequency range have been also included in this class:
-
A single-emission band, designated as evolving emission line (EEL), first reported by Chernov et al.
[44] in the 350–450 MHz frequency range, at the low-frequency cutoff of a pulsating structure, and by Fu et al.
[20] and Ning et al.
[45] in the GHz range is included in this class, although the emission mechanism appears to be somewhat different from the zebras. After Chernov et al.
[44], the observed EEL was the result of electrostatic maser at double plasma resonance.
-
Some “serpentine” single-emission bands recorded in the ∼5 GHz frequency and designated as a “quasi-periodic drifting” structure (
[20], Figure 9), or “M-shaped structure” (, Figure 3) may be classified, either as emission bands or as narrow-band bursts of the type-III family (M-Bursts).
-
A structure detected at about 1416–1420 MHz by Oberoi et al.
[46] . It consists of a group of short 2–4 ms parallel stripes with a relative delay with decreasing frequency. The total duration of the group is about 16 ms. Due to the band structure, this burst may be considered as a group of emission bands; the short time scale and frequency bandwidth of these bands, however, show some similarity of this burst to narrow-band burst chains.
3. Summary & Discussion of the Classification Scheme
Most of the fine-structure classification efforts have been based on morphological criteria derived from dynamic spectra, with the scheme presented in this entry being no exception.
It was based on observations in metric wavelengths but introduced a novel two-level classification approach was adopted, which comprised a first level of five categories based on coarse spectral features and a second level with two or more subcategories based on the finer details of the features as already mentioned in Section 2. The five basic categories
were also, found to be statistically associated with the phases of the associated flare/CME evolution and the energy release process.
The basic limitation of this scheme, and all previous, is that ephasizes more the morphology that the underlying physical and radiation processes remains. Under the circumstaces certain subclasses, or even classes, may include bursts that are morphologically similar yet originate from different processes. Yet, regardless of these limitations, a classification scheme is still needed as a background for theoretical work
This entry is adapted from the peer-reviewed paper 10.3390/universe9100442