Compared with organic analogs, onium derivatives of nido-carborane have increased stability due to the stabilizing electron-donor action of the boron cage. Charge-compensated derivatives are considered according to the type of heteroatom bonded to a boron atom.
1. Introduction
The synthesis of the first polyhedral boranes, carboranes, and metallacarboranes in the early 1960s was one of the major highlights in the development of inorganic chemistry over the last century
[1]. The first reports on the synthesis of icosahedral carboranes appeared almost sixty years ago, at the end of 1963 when both the United States and the Soviet Union almost simultaneously declassified documents about their boron fuel projects
[2][3][4][5][6]. A few months later, the nucleophile-promoted removal of one boron atom from the icosahedral ortho-carborane cage to form the 11-vertex nido-carborane cage species (
Figure 1) was reported
[7][8]. It was one of the most significant findings in the early years of the development of carborane chemistry, and now, more than five decades later, it remains indispensable for the synthesis of numerous metallacarboranes
[9][10][11][12][13][14][15][16][17] and hydrophilic functionalized carboranes for medical
[18][19][20][21][22][23][24][25][26][27] and other
[28][29][30][31][32][33][34][35][36][37][38][39][40] applications.
Figure 1. The idealized structure and atom numbering of nido-carborane [7,8-C2B9H12]−.
Metallacarboranes based on the dicarbollide ligand [7,8-C
2B
9H
11]
2−, which is formed upon the deprotonation of nido-carborane with strong bases, resemble the well-known transition metal cyclopentadienyl complexes. However, the dicarbollide ligand differs from the cyclopentadienyl ligand in a number of ways. In addition to its 3D character, the dicarbollide ligand is a significantly stronger donor than the cyclopentadienyl one and has a double charge. The donor nature of the dicarbollide ligand can be largely tuned via the introduction of substituents of various natures. At the same time, the charge of the ligand can be partially compensated by introducing into the dicarbollide ligand the so-called charge-compensating substituents of an onium nature (ammonium, phosphonium, sulfonium, etc.). This significantly brings the properties of the dicarbollide and cyclopentadienyl complexes closer together and causes a high interest in metallacarboranes based on charge-compensated dicarbollide ligands
[41][42][43][44][45][46][47][48][49][50].
2. Charge-Compensated Derivatives of Nido-Carborane with Boron–Nitrogen Bond
Due to the great diversity of nitrogen chemistry, the charge-compensated derivatives of nido-carborane with the B-N bond are characterized by the greatest variety of forms. The first example of the synthesis of charge-compensated derivatives of nido-carborane with a B-N bond was the reaction of the parent nido-carborane with pyridine in benzene in the presence of anhydrous FeCl
3, leading to the asymmetrically substituted pyridinium derivative 9-Py-7,8-C
2B
9H
11 (
Scheme 1)
[51], the structure of which was later supported via a single-crystal X-ray diffraction study (
Figure 2)
[52]. When FeCl
3·6H
2O was used instead of anhydrous FeCl
3, the by-product of the reaction was the disubstituted pyridinium derivative 9,11-Py
2-7,8-C
2B
9H
9 [52]. The reaction with 7,8-dimethyl-nido-carborane proceeds in a similar way, giving 9-Py-7,8-Me
2-7,8-C
2B
9H
9 [51]. In a similar way, the reaction of nido-carborane with methyl isonicotinate in the presence of FeCl
3 in refluxing benzene results in 9-(4′-MeO(O)CC
5H
3N)-7,8-C
2B
9H
11 [53].
Scheme 1. The synthetic process to obtain 9-Py-7,8-C2B9H11.
Figure 2. Crystal molecular structures of 9-Py-7,8-C2B9H11 (left) and 10-Py-7,8-C2B9H11 (right). Hydrogen atoms of organic substituents are omitted for clarity.
The reaction of
nido-carborane with pyridine in the presence of HgCl
2 in refluxing benzene gives a mixture of the symmetrically and asymmetrically substituted pyridinium derivatives 10-Py-7,8-C
2B
9H
11 and 9-Py-7,8-C
2B
9H
11 in a ratio of 2:1 (
Scheme 2)
[50][54]. The reaction of 7,8-dimethyl-
nido-carborane [7,8-Me
2-7,8-C
2B
9H
10]
− with pyridine proceeds in a similar way
[54].
Scheme 2. Synthesis of 10-Py-7,8-C2B9H11 and 9-Py-7,8-C2B9H11 via the reaction of nido-carborane with pyridine in the presence of HgCl2.
The asymmetrically substituted 9-pyridinium derivative of
nido-carborane was also prepared via the reaction of the parent
ortho-carborane with pyridine in the presence of copper acetate and water. Similar reactions with
C-monosubstituted
ortho-carboranes give a mixture of the corresponding isomeric pyridinium derivatives 9-Py-7-R-7,8-C
2B
9H
10 and 11-Py-7-R-7,8-C
2B
9H
10 (R = Me, Ph). In the case of 1-XCH
2 derivatives of
ortho-carborane (X = Cl, Br, OH), in addition to a mixture of the corresponding 9- and 11-pyridinium derivatives of
nido-carborane, the reaction gives the pyridinium methyl derivative 7-PyCH
2-7,8-C
2B
9H
11 [55].
3. Charge-Compensated Derivatives of Nido-Carborane with Boron–Phosphorus Bond
Unlike derivatives with a boron–nitrogen bond, derivatives of
nido-carboranes with a boron–phosphorus bond can be prepared using electrophilic substitution reactions. Heating the potassium salt of
nido-carborane K[7,8-C
2B
9H
12] with Ph
2PCl in tetrahydrofuran at reflux leads to the
P-protonated diphenylphosphonium derivative 9-Ph
2PH-7,8-C
2B
9H
11, which can be alkylated with MeI under reflux in ethanol in the presence of EtONa as a base to give the methyldiphenylphosphonium derivative 9-MePh
2P-7,8-C
2B
9H
11 (
Scheme 3,
Figure 3)
[56][57].
Scheme 3. The synthesis of 9-Ph2PH-7,8-C2B9H11 and its alkylation.
Figure 3. Crystal molecular structures of 9-MePh
2P-7,8-C
2B
9H
11 (
left) and 10-MePh
2P-7,8-C
2B
9H
11 (right). Hydrogen atoms of phenyl and methyl groups are omitted for clarity.
The symmetrically substituted phosphonium derivatives 10-Ph
2PH-7,8-C
2B
9H
11 and 10-MePh
2P-7,8-C
2B
9H
11 (
Figure 4) were prepared in a similar way using the disodium dicarbollide salt Na
2[7,8-C
2B
9H
11] as a starting material (
Scheme 4)
[57].
Scheme 4. The synthesis of 10-Ph2PH-7,8-C2B9H11 and its alkylation.
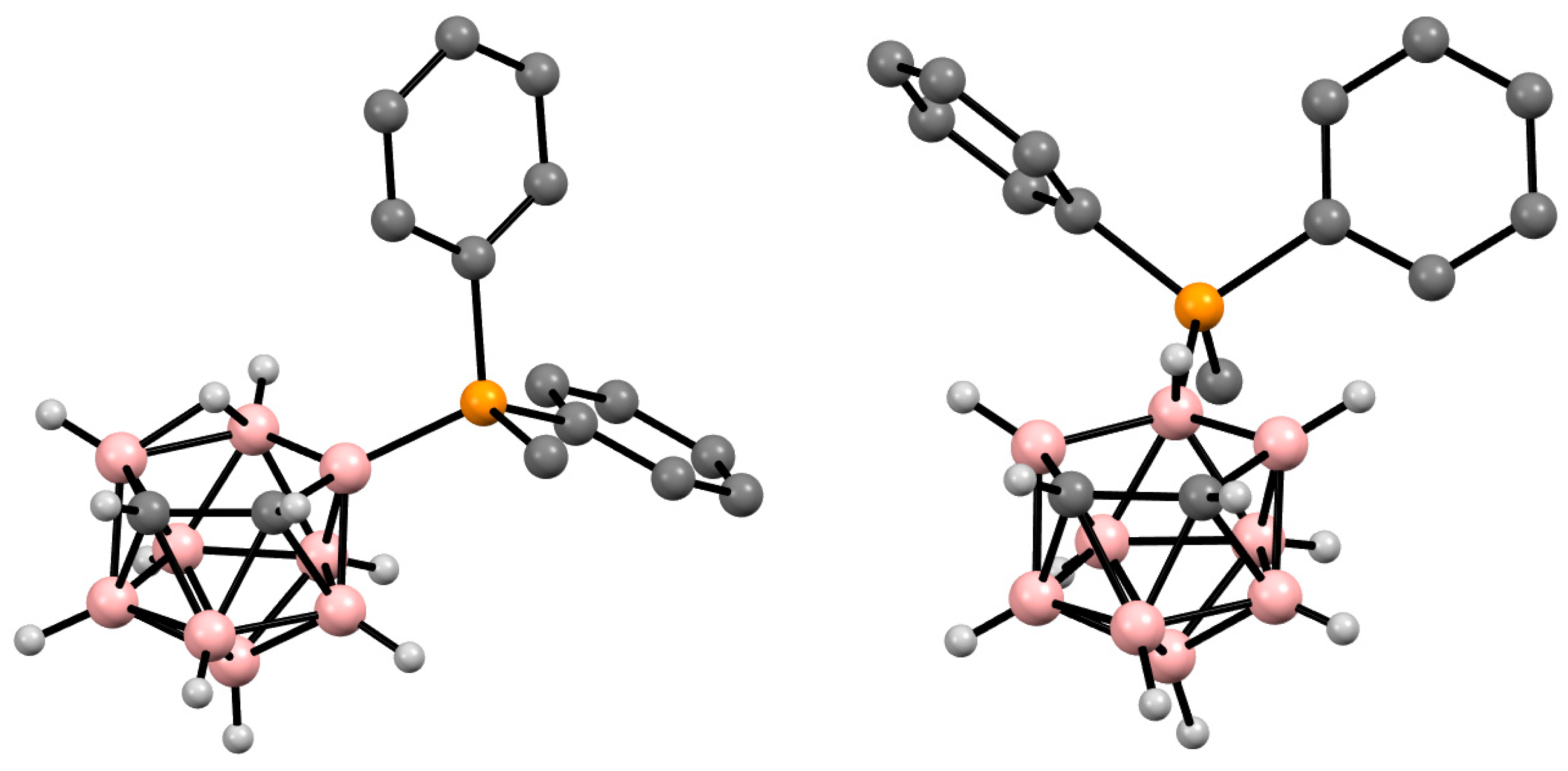
Figure 4. Crystal molecular structures of 9-MePh
2P-7,8-C
2B
9H
11 (
left) and 10-MePh
2P-7,8-C
2B
9H
11 (right). Hydrogen atoms of phenyl and methyl groups are omitted for clarity.
Phosphonium derivatives of
nido-carborane also can be prepared via Lewis-acid-mediated nucleophilic substitution reactions. The reaction of the potassium salt of
nido-carborane K[7,8-C
2B
9H
12] with PPh
3 in the presence FeCl
3 in benzene at 80 °C leads to a mixture of triphenylphosphonium 9-Ph
3P-7,8-C
2B
9H
11 and 10-Ph
3P-7,8-C
2B
9H
11, which were separated using column chromatography on silica (
Scheme 5)
[56][57].
Scheme 5. Reaction of nido-carborane with triphenylphosphine in the presence of FeCl3.
The asymmetrical triphenylphosphonium derivative 9-Ph
3P-7,8-C
2B
9H
11 was also obtained via the reaction of triphenylphosphine with the dithallium dicarbollide salt Tl
2[7,8-C
2B
9H
11] in dichloromethane and in the presence of AgBr at ambient temperature (
Scheme 6)
[58].
Scheme 6. Synthesis of 9-Ph3P-7,8-C2B9H11.
Similar to the pyridinium derivatives, a series of asymmetrically substituted phosphonium derivatives 9-R’R
2P-7,8-Ph
2-7,8-C
2B
9H
9 was prepared via electrocatalyzed B-P oxidative couplings of 7,8-diphenyl-
nido-carborane with various phosphines and phosphites (
Scheme 7,
Figure 5)
[59].
Scheme 7. The electrocatalyzed B-P oxidative coupling of triarylphosphines, diarylalkylphosphines, and trialkylphosphines with 7,8-diphenyl-
nido-carborane.
Figure 5. Crystal molecular structure of 9-Ph3P-7,8-Ph2-7,8-C2B9H9. Hydrogen atoms of phenyl rings are omitted for clarity.
4. Charge-Compensated Derivatives of Nido-Carborane with Boron–Arsenic and Boron–Antimony Bonds
The charge-compensated derivatives of
nido-carborane with boron–arsenic and boron–antimony bonds are rare and are limited to a few examples. Similar to the triphenylphosphonium derivative, the asymmetrically substituted triphenylarsonium and tetraphenylstilbonium derivatives 9-Ph
3X-7,8-Ph
2-7,8-C
2B
9H
9 (X = As, Sb) were prepared via electrocatalyzed oxidative couplings of 7,8-diphenyl-
nido-carborane with Ph
3As and Ph
3Sb, respectively (
Scheme 8,
Figure 6)
[59].
Scheme 8. Synthesis of 9-Ph3As-7,8-Ph2-7,8-C2B9H9 and 9-Ph3As-7,8-Ph2-7,8-C2B9H9.
Figure 6. Crystal molecular structure of 9-Ph3As-7,8-Ph2-7,8-C2B9H9. Hydrogen atoms of phenyl groups are omitted for clarity.
The reaction of 2-pyridyl-substituted
nido-carborane [7-(2′-Py)-7,8-C
2B
9H
11]
− with triphenylarsine in the presence of catalytic amounts of PdCl
2 in a mixture of toluene, water, and acetonitrile at 120°C results in the corresponding triphenylarsonium derivative 11-Ph
3As-7-(2′-Py)-7,8-C
2B
9H
10 (
Scheme 9)
[60].
Scheme 9. Synthesis of 11-Ph3As-7-(2′-Py)-7,8-C2B9H10.
5. Charge-Compensated Derivatives of Nido-Carborane with Boron–Oxygen Bond
Alkyloxonium salts are much less stable than ammonium and phosphonium salts, and some of them are used in organic chemistry as strong alkylating agents. Nevertheless, strong electron-withdrawing of polyhedral boron hydride clusters substituted at boron atoms and, in particular,
nido-carborane
[61], is capable of stabilizing their oxonium derivatives
[62][63]. The first example of such a derivative was obtained very soon after the discovery of
nido-carborane via the reaction of the potassium salt of
nido-carborane with tetrahydrofuran in the presence of FeCl
3 in benzene. As a result, a mixture of two isomeric tetrahydrofuran derivatives of
nido-carborane was obtained. The reaction with the
C,
C′-dimethyl derivative of
nido-carborane proceeds in a similar way (
Scheme 10)
[51].
Scheme 10. Synthesis of 9-(CH
2)
4O-7,8-C
2B
9H
11 and 10-(CH
2)
4O-7,8-C
2B
9H
11 via the reaction of
nido-carborane with FeCl
3 in THF–benzene mixture.
It was later found that the replacement of FeCl
3 with HgCl
2 in this reaction leads to the selective formation of the symmetrically substituted tetrahydrofuran derivative 10-(CH
2)
4O-7,8-C
2B
9H
11 [54][64]. The symmetrically substituted derivative was also obtained via the reaction of the tetramethylammonium salt of
nido-carborane with AlCl
3 in a mixture of tetrahydrofuran and acetone
[65] and by the treatment of the potassium salt of
nido-carborane with tetrahydrofuran in the presence of acetaldehyde or formaldehyde and hydrochloric acid in a mixture of water and toluene
[66] (
Scheme 11).
Scheme 11. Different pathways for synthesis of 10-(CH2)4O-7,8-C2B9H11.
Oxonium derivatives of
nido-carborane with other cyclic ethers were synthesized as well. The symmetrically substituted 1,4-dioxane derivative 10-O(CH
2CH
2)
2O-7,8-C
2B
9H
11 can be prepared via the reaction of the potassium salt of
nido-carborane with 1,4-dioxane in the presence of HgCl
2 in benzene
[64] or in the presence of acetaldehyde and hydrochloric acid in a water–toluene mixture
[66]. The 1,4-dioxane derivative can also be synthesized via the heating of the protonated form of
nido-carborane 7,8-C
2B
9H
13 with 1,4-dioxane
[67] (
Scheme 12). The molecular structure of the 1,4-dioxane derivative of
nido-carborane was determined using single-crystal X-ray diffraction (
Figure 7)
[68].
Scheme 12. Different pathways for synthesis of 10-O(CH2CH2)2O-7,8-C2B9H11.
Figure 7. Crystal molecular structure of 10-O(CH2CH2)2O-7,8-C2B9H11. Hydrogen atoms of organic substituent are omitted for clarity.
The reaction of the potassium salt of
nido-carborane with tetrahydropyran in the presence of mercury(II) chloride in benzene results in the tetrahydropyran derivative 10-(CH
2)
5O-7,8-C
2B
9H
11 (
Scheme 13)
[69][70].
Scheme 13. Different pathways for synthesis of 10-(CH2)5O-7,8-C2B9H11.
6. Charge-Compensated Derivatives of Nido-Carborane with Boron–Sulfur Bond
Compared with the oxonium derivatives, the sulfonium derivatives of
nido-carborane are represented by a wider variety of derivatives and synthetic methods for their preparation. However, the most studied of them are the dimethylsulfonium derivatives of
nido-carborane, which are widely used for the synthesis of metallacarboranes
[41][50][71].
It should be noted that symmetrically and asymmetrically substituted dimethylsulfonium derivatives of
nido-carborane are usually obtained in different ways, which excludes the formation of mixtures of their isomers. The asymmetrically substituted 9-dimethylsulfonium derivative of
nido-carborane 9-Me
2S-7,8-C
2B
9H
11 was prepared via the reaction of the parent
nido-carborane with dimethylsulfoxide in the presence of sulfuric acid at 80°C
[52][72][73]. The reactions of the
C,
C’-substituted derivatives of
nido-carborane proceed in a similar way, leading to the corresponding dimethylsulfonium derivatives 9-Me
2S-7,8-R
2-7,8-C
2B
9H
9 (R = Me, Ph)
[52][74] (
Scheme 54). These conditions are similar to those used for the synthesis of the dimethylsulfonium derivatives of the
closo-decaborate
[75] and
closo-dodecaborate
[76] anions. The
C,
C′-substituted derivatives 9-Me
2S-7,8-Me
2-7,8-C
2B
9H
9 and 9-Me
2S-7,8-μ-(1′,2′-C
6H
4(CH
2)
2)-7,8-C
2B
9H
9 were prepared via the reactions of the corresponding
nido-carboranes with dimethylsulfoxide in the presence of triflic acid in 1,2-dichloroethane
[77] (
Scheme 14). The structures of 9-Me
2S-7,8-R
2-7,8-C
2B
9H
9 (R = H, Ph) were determined using single-crystal X-ray diffraction (
Figure 8)
[78][79].
Scheme 14. Synthesis of the asymmetrically substituted dimethylsulfonium derivatives of
nido-carborane 9-Me
2S-7,8-R
2-7,8-C
2B
9H
9 (R = H, Me, Ph; RR = μ-1′,2′-C
6H
4(CH
2)
2).
Figure 8. Crystal molecular structures of 9-Me
2S-7,8-C
2B
9H
11 (
top), 11-Me
2S-7-Ph-7,8-R
2-7,8-C
2B
9H
10 (
bottom left), and 9-Me
2S-7,8-Ph
2-7,8-C
2B
9H
9 (
bottom right). Hydrogen atoms of organic substituent are omitted for clarity.
The asymmetrically substituted dimethylsulfonium derivatives 9-Me
2S-7,8-Me
2-7,8-C
2B
9H
9 and 9-Me
2S-7,8-µ-(CH
2OCH
2)-7,8-C
2B
9H
9 were prepared via the reactions of the corresponding
nido-carboranes with dimethylsulfide in the presence of Fe(NO
3)
3 in aqueous ethanol
[49].
In the case of
C-substituted
nido-carboranes, such as K[7-Ph-7,8-C
2B
9H
11], the introduction of a Me
2S group results in a mixture of 9-Me
2S-7-Ph-7,8-C
2B
9H
10 and 11-Me
2S-7-Ph-7,8-C
2B
9H
10 isomers, which can be separated using column chromatography
[73]. The molecular structure of 11-Me
2S-7-Ph-7,8-C
2B
9H
10 was determined using single-crystal X-ray diffraction (
Figure 8)
[74].
7. Charge-Compensated Derivatives of Nido-Carborane with Boron–Selenium and Boron–Tellurium Bonds
The charge-compensated derivatives of
nido-carborane with boron–selenium and boron–tellurium bonds are rather rare. Similar to the dialkyl- and diarylsulfonium derivatives, a series of asymmetrically substituted triakyl(aryl)selenium and triaryltellurium derivatives 9-RR’X-7,8-Ph
2-7,8-C
2B
9H
9 (X = Se, Te) were prepared via electrocatalyzed oxidative couplings of 7,8-diphenyl-
nido-carborane with RR’Se and R
2Te, respectively (
Scheme 15,
Figure 9)
[59].
Scheme 15. Synthesis of 9-dialkyl(aryl)selenium and 9-diaryltellurium derivatives of nido-carborane 9-RR’X-7,8-Ph2-7,8-C2B9H9.
Figure 9. Crystal molecular structure of 9-Ph2Te-7,8-Ph2-7,8-C2B9H9. Hydrogen atoms of phenyl groups are omitted for clarity.
8. Some Other Charge-Compensated Derivatives of Nido-Carborane
The asymmetrically substituted 9-carbonyl derivative of
nido-carborane 9-O≡C-7,8-C
2B
9H
11 and the 3,3,8-(CO)
3-3,1,2-CoC
2B
9H
10 cobaltacarborane based on its symmetrically substituted analog as a ligand were isolated as minor products of the reaction of the parent
nido-carborane with [Co
2(CO)
8]
[80].
The symmetrically substituted cobaltacenium derivative of
nido-carborane 10-{CpCo(C
5H
4)}-7,8-Me
2-7,8-C
2B
9H
9 (
Figure 10) was prepared along with the 3-Cp-1,2-Me
2-3,1,2-CoC
2B
9H
9 cobaltacarborane in the reaction of the dithallium dicarbollide salt Tl
2[7,8-Me
2-7,8-C
2B
9H
9] with CpCo(CO)I
2 in acetonitrile
[81].
Figure 10. Crystal molecular structure of 10-{CpCo(C5H4)}-7,8-Me2-7,8-C2B9H9. Hydrogen atoms of methyl groups are omitted for clarity.