1. VO2max Decline in the Long Term: Effects of Training in Masters Athletes
Masters endurance athletes represent an excellent model of healthy aging that is relevant for the evaluation of consequences of changing in training habit on VO
2max. Although VO
2max seems to inexorably decrease with aging, even in life-long runners, the potential modulatory capacity of short- and long-term training variations remains a matter of debate. In healthy sedentary adults of both sexes, VO
2max declines by about 10% per decade after the age of 25–30 years and slightly more at older ages (e.g., older than 60–70 years). Overall, this rate seems not to differ much in endurance athletes
[1][6]. Longitudinal data from a healthy population indicate an exacerbated VO
2max reduction with aging; whereas the reduction was about 8% in the fourth decade (over 10 years), it amounted 23% in the seventh decade and was more pronounced in males than females
[2][13]. Whereas higher physical activity levels were associated with higher VO
2max values across all ages and in both sexes, physical activity (quartiles) did not change the slope of the VO
2max drop
[2][13]. However, this may not hold true for (at least male) masters endurance athletes, who are able to maintain high training volumes (and likely intensity) until old age (
Figure 1). The presented data derived from longitudinal studies are in line with cross-sectional studies
[3][39]. Cross-sectional data from 203 men (male endurance athletes and untrained subjects, 20–90 years) indicate that the weekly training volume constitutes a significant positive predictor of age-related changes in aerobic capacity
[4][40]. Other cross-sectional data of masters athletes (20 power and 19 endurance athletes, 37–90 years) recently reported a peak aerobic and anaerobic power decline of around 7–14% per decade, with no difference between athletic disciplines or sexes
[5][41]. Because exercise tests were stopped when heart rates exceeded the age-predicted maximal heart rate by 10 bpm and due to the lack of information on training characteristics, assessments of potential training effects on the observed VO
2max decline are not possible
[5][41]. Similarly, another cross-sectional study suggests that active older men (n = 146) lose maximal aerobic power at a rate of about 12%, and active older women (n = 82) lose maximal aerobic power at a rate of about 8% per decade between the ages of 40 and 80 years
[6][42]. However, the VO
2max decline becomes considerably steeper in masters athletes older than 80 years old, even in the absence of major illnesses or orthopedic issues
[7][43]. Again, aging and training effects cannot be readily disentangled in that study due to the large variations regarding years of training (15.2 ± 9.7) and weekly training milage (33.5 ±19.3)
[6][42].
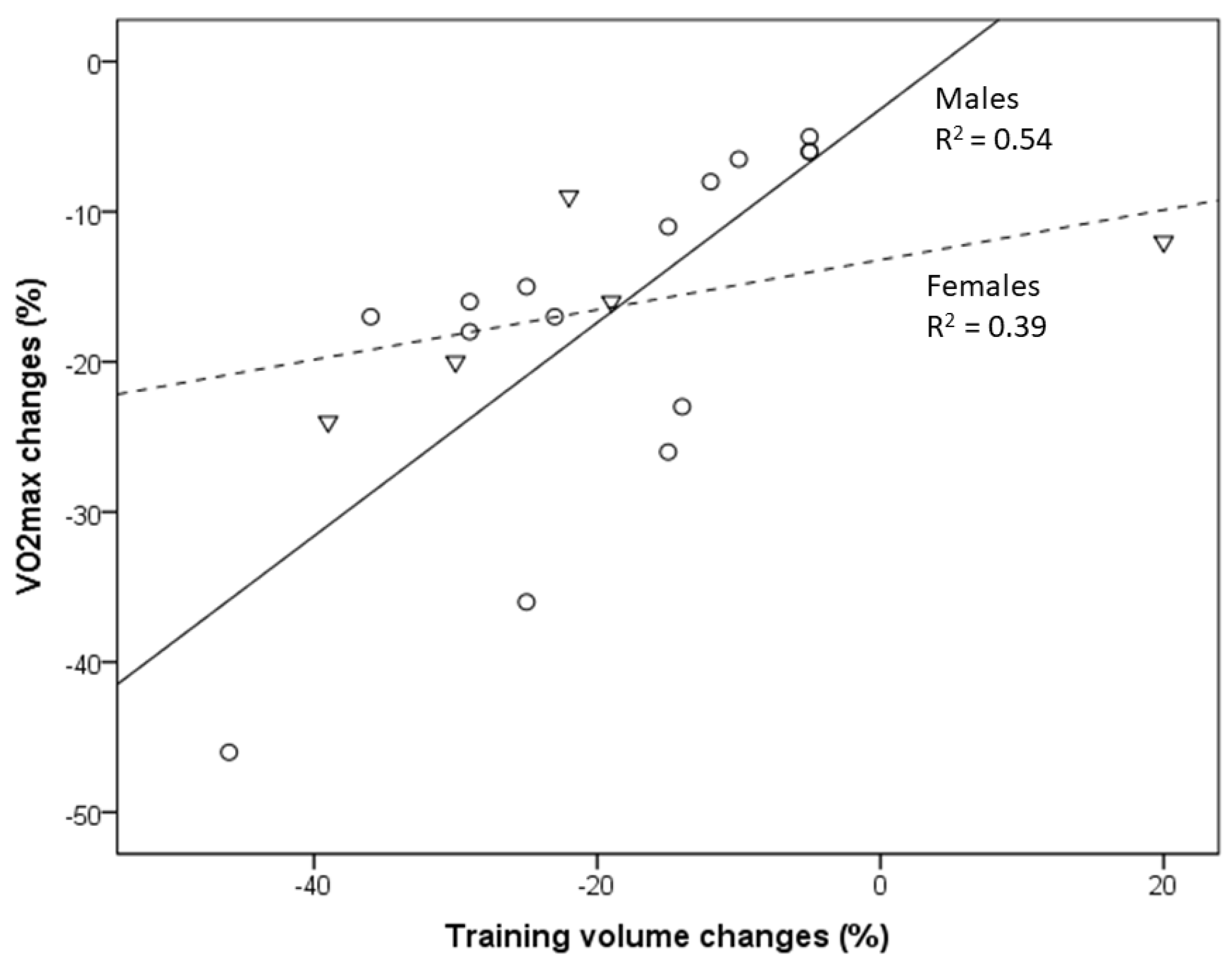
Figure 1. Relationship between VO
2max decline and the reduction in training volume with aging of masters athletes
[8][9][10][11][12][13][16,17,18,19,20,21]. Circles indicate males, and triangles indicate females. Changes in the training volume explain 54% and 39% of the variance in VO
2max changes in male and female masters athletes, respectively.
In a comparison of American road running events, it was recently shown that the sex gap in performance decreases with increased age
[14][44]. In the present study, the association between VO
2max decline and changes in training volume (and intensity) is less pronounced in female compared to male endurance athletes
[8][13][16,21]. This may partly be related to the lack of studies evaluating female athletes but also to the outlier of one female group studied by Eskurza et al.
[8][16]. Nevertheless, a somewhat lower trainability of aerobic capacity in women may contribute to those differences
[15][45].
Longitudinal changes in VO
2max in cross-country skiers (age 58.7 ± 2.3 years) who continuously competed and reported unchanged training patterns amounted to only −4.1 ± 3.7 % per decade
[16][46], which fits well with longitudinal data from male masters athletes (runners) presented in the present study, with a decrease in VO
2max of between −5% and −6.5% per decade shown in those who continued to engage in regular vigorous endurance exercise, training and competitions
[9][10][11][12][17,18,19,20]. The exaggerated VO
2max decline in masters athletes who reduced training volumes can be readily explained from both training scientific and practical perspectives
[17][18][19][47,48,49]. Training characteristics (e.g., training volume, intensity and frequency) are undisputedly important modifiers of VO
2max in both young and older athletes
[20][50]. Reductions in volume and/or intensity of exercise training and the resulting decline in VO
2max and performance in aging endurance athletes may be caused by various factors, such as decreasing motivation to train and/or compete, musculoskeletal injuries, pain and/or aging-related diseases
[1][21][22][23][24][2,6,51,52,53], sometimes even associated with excessive training and competition activities
[25][54]. Consequently, the aging-related CRF decrease, especially in male masters athletes, will remain small in those who are able to avoid factors provoking considerable training reductions. In summary, male masters athletes who can maintain their training volume (and intensity) can minimize their VO
2max decline to about −5% to −6.5% per decade
[9][10][11][12][17,18,19,20], but those values increase to up to −26% in those who reduce their training to a moderate level and can even be as high as −46% per decade in those who become sedentary
[9][10][11][12][13][17,18,19,20,21].
2. Body Composition Explain the VO2max Decline in Masters Athletes
The effects of regular exercise are closely related to body composition and are therefore difficult to disentangle. Aging-related training reductions or cessation are often associated with changes in body composition. Longitudinal and cross-sectional data
[26][55] indicate a significant contribution of lean body mass (LBM) alterations to VO
2max changes, an effect that seems to be more pronounced in males than in females. Accordingly, a recent study demonstrated that lifelong aerobic exercise attenuated the age-related loss of quadricep muscle volume by about 50% in men but not in women, whereas adipose tissue infiltration into muscle was attenuated by about 50% in both sexes
[27][56]. Higher training intensity throughout life provided increased protection against adipose tissue infiltration into muscle. The aerobic qualities of skeletal muscle among these lifelong exercisers likely contributed to the strong muscle mass-to-VO
2max relationship. In line with these finding, no association between appendicular muscle mass and age was found in highly active older adults of both sexes
[28][57]. The significant difference in muscle mass between exercising and sedentary individuals clearly illustrates that levels of physical activity play a considerable role in the phenotype of aging muscle. A recent study provided novel data on body composition in masters track and field athletes
[29][58]. The authors concluded that loss of skeletal muscle mass and changes in bioimpedance phase angle (a raw parameter of cellular function and an indicator of tissue hydration and nutritional status
[30][59]) are important contributors to the age-related reduction in anaerobic power, even in athletes who maintain high levels of physical activity in old age, indicating a deterioration of muscle quality in old age. However, it is also possible that a favorable body composition and nutritional conditions are prerequisites to participate in masters sport. Therefore, future research employing longitudinal study designs is needed, with the aim of determining which of these measures alone or in combination optimally predict individuals’ fitness and general health markers.
3. VO2max Decline in the Short Term: Effects of Training Cessation and Training (Re)Uptake
The VO
2max decline in endurance-trained individuals usually does not occur gradually over decades but rather consists of a “slow component” related to aging per se and a “rapid component” due to training reduction/cessation. As demonstrated in the presented results, an almost linear VO
2max decrease or increase was observed during a 12-week period of training cessation or training (re)uptake, respectively, in healthy young and older individuals
[31][32][33][34][12,24,28,31]. Compared to the −5% to −6.5% VO
2max decline within a decade in male masters athletes
[9][10][11][12][17,18,19,20], VO
2max steeply decreased as a result of training cessation, even in young endurance-trained (the majority of participants included in this study were male) athletes by −7% after only 12 days and dropped further to −18% after 12 weeks of training cessation
[35][22]. Similar observations have been published for young competitive female runners, for whom 5 days of training cessation did not yield significant changes, whereas 10 days resulted in a 7.6% drop, which was linked to reduced running performance after 15 days
[32][24].
However, VO
2max rapidly improves after a few weeks of training (re)uptake after cessation or when training starts from a sedentary status. VO
2max improvements occurred more rapidly during the first 3 weeks (12% vs. 8.4%) in young compared to older healthy men, but after 12 weeks, the increases were even higher in the older compared to younger men (15.4% vs. 29.3%)
[34][31], at least in the selected studies considered here (
Figure 2). A 31% VO
2max increase was reported in a female masters athlete after only 4 weeks of training (re)uptake following trauma-related training cessation
[31][12]. A meta-analysis demonstrated a 16.3% VO
2max improvement in previously sedentary older adults (>60 years) of both sexes after taking up an aerobic exercise training program; the effects increased with longer training duration (i.e., >16–20 weeks)
[36][60]. VO
2max increases of 9–13% were observed after 8 weeks of high-intensity interval training (HIIT) in men and women of a broad age range (20 to 70+), without significant differences between age groups
[37][61]. These findings should encourage masters athletes who become sedentary for any reason to take up training again as soon as possible. In summary, a VO
2max loss corresponding to aging-related decline of at least one aging decade can be restored by appropriate training (re)uptake within a couple of weeks.
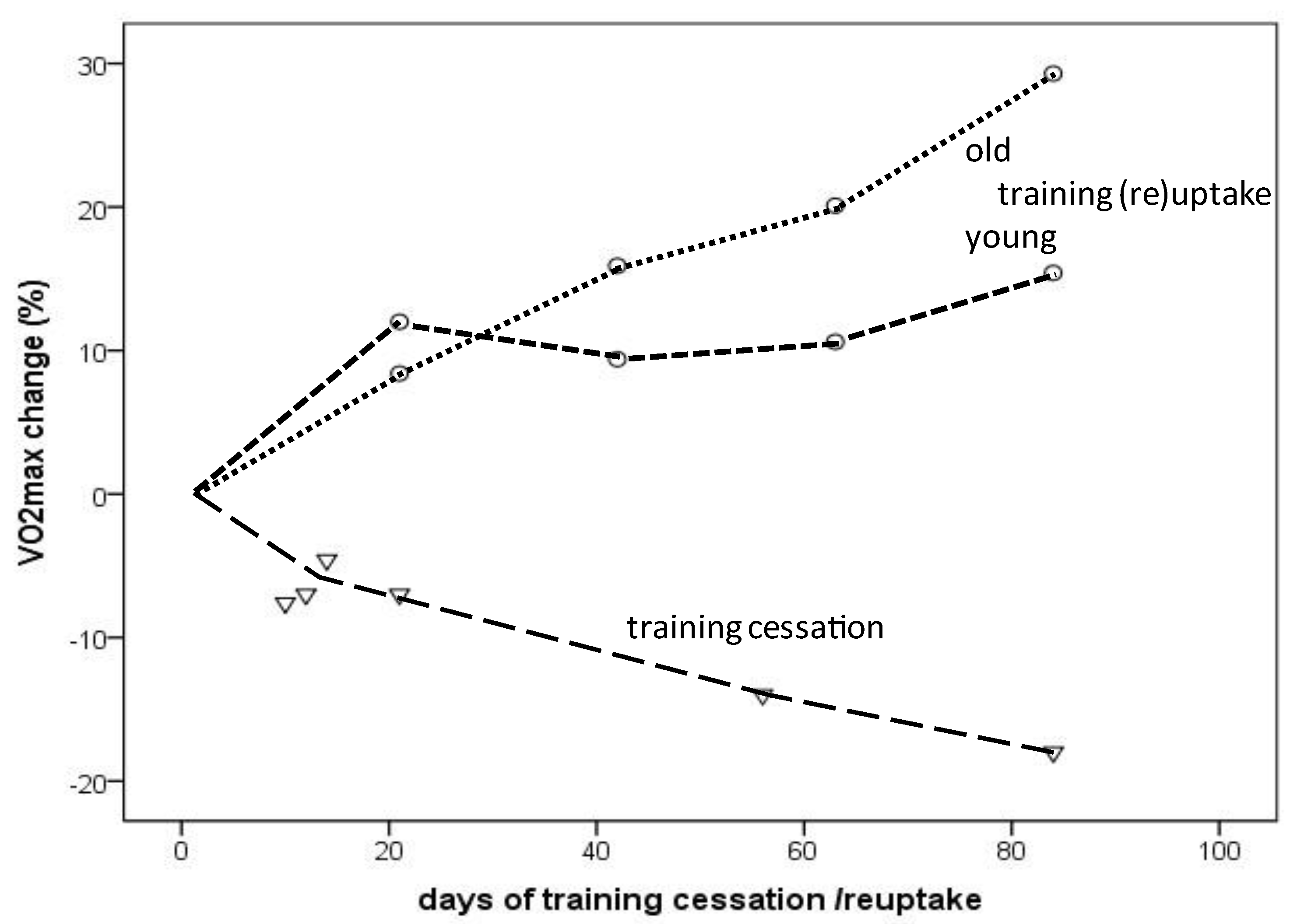
Figure 2. VO2max change depending on the amount (days) of training cessation (triangles) or training (re)uptake (circles: dotted line indicates older individuals; dashed line indicates young individuals).
4. Aging-Related VO2max Loss despite Maintenance of High Training Volume and Intensity: The Slow Component
The age-related decline in endurance performance and its physiological determinants are largely mediated by a reduction in the volume and intensity of training commonly observed in masters endurance athletes
[17][47]. However, as demonstrated, a VO
2max loss of between −5% and −6.5% per decade occurred even in those who continued to engage in regular vigorous endurance exercise training and competitions
[9][10][11][12][17,18,19,20]. The question arises as to which aging effects that are largely independent of training habits are responsible for this decline. In contrast to the decrease in lung function
[38][62], both cross-sectional and longitudinal studies indicate that the decreases in maximal heart rate and stroke volume are the major contributors to the observed decline in VO
2max in masters endurance athletes
[4][17][18][39][40][40,47,48,63,64]. Alteration of the intrinsic heart rate may primarily account for the reduction in maximal heart rate, but diminished chronotropic responsiveness to β-adrenergic stimulation probably adds to this reduction
[41][42][8,65]. In addition, lower ejection fractions at maximal exercise volume have been reported in both older sedentary and older endurance-trained athletes compared with their young peers, which is apparently related to reduced β-adrenergic responsiveness
[43][66]. Whereas left ventricular filling pressure and arteriovenous oxygen difference remain almost unchanged at peak exercise, chronotropic and inotropic reserve (and probably Frank–Starling reserve) seem to decline even with healthy aging
[44][67]. Whereas longitudinal and radial contributions to stroke volume do not seem to differ between groups of varying ages and training status, differences in longitudinal pumping were observed between young sedentary and old highly trained athletes compared to old sedentary individuals; the incidence of left ventricular atrioventricular plane displacement was higher in the former groups
[45][68]. Moreover, slight macrovascular and microvascular dysfunctions accompanied by stiffening of central elastic arteries and impaired peripheral endothelial function are aging-related effects that negatively affect blood flow and oxygen delivery to exercising skeletal muscles
[46][69]. Although endothelial function may be preserved in the leg muscles of life-long physically active people due to elevated systemic nitric oxide bioavailability, this did not restore the aging-related decline in hyperemic response
[47][48][70,71]. No or only negligible impairments were reported in masters athletes with regard to muscle fiber morphology and capillary supply of the muscle tissue
[49][72].
5. Aging-Related VO2max Decrease or Increase with Training Cessation or (Re)Uptake: The Fast Component
Age-related VO
2max decline is determined by multiple factors, including reductions in blood volume, maximal heart rate and cardiac output; increased stiffening of the arterial walls; exercise-induced arterial hypoxemia; and diminished peripheral oxygen extraction. However, both loss and improvement of VO
2max due to recent changes in exercise training seem to be primary consequences of changes (decrease or increase, respectively) in maximal cardiac output
[34][35][50][11,22,31]. In a study on young male athletes, within the first 3 weeks, training cessation elicited a rapid response that involved a decrease in oxygen delivery due to reduced maximal cardiac output, followed by an increasing contribution of elevated arteriovenous oxygen difference
[35][22]. Maximal cardiac output seems to largely be a consequence of the reduction in plasma volume related limiting ventricular filling and maximal stroke volume
[33][51][28,73]. The reduced stroke volume is incompletely compensated by a slight increase in maximal heart rate
[33][51][28,73]. Whereas the arteriovenous oxygen difference may more rapidly recover in young and healthy males during training (re)uptake, increasing maximal cardiac output seems to contribute more importantly in older males
[34][31]. Short-term training in older men and women results in rapid elevation of plasma volume and associated increases in cardiac output and VO
2max
[52][74]. This expansion of plasma volume following short-term exercise training (e.g., 2–4 weeks) has been documented in both cross-sectional and longitudinal studies
[53][54][75,76]. Thermal and non-thermal components have been suggested to contribute to the elevation of plasma levels of electrolytes and proteins
[53][54][75,76].
As shown in the study by Coyle et al., muscle capillarization did not markedly change over the 12-week training cessation (but remained higher than in the sedentary subjects); thus, the authors suggested that the decreasing arteriovenous oxygen difference could be attributed to a loss of mitochondria and/or mitochondrial function
[35][22]. However, as muscle mitochondrial respiration is submaximal at VO
2max, this likely is not the cause of VO
2max impairment after short-term training cessation
[55][56][77,78]. Calculation of the arteriovenous oxygen difference using the Fick equation suggests its reduction may result from elevated mixed venous oxygen content caused by distribution deficits of blood flow, i.e., reduced blood flow within working skeletal muscles. Skeletal muscle blood flow is diminished during dynamic exercise in older and rather sedentary individuals, resulting from a reduced vascular conductance (impaired functional sympatholysis), but improves with exercise training
[57][58][79,80]. A demonstrated improvement in sympatholysis resulting from short-term exercise training was modulated by training intensity and was mediated by a nitric-oxide-dependent mechanism
[59][81]. Moreover, elevated sympathetic vasoconstrictor responsiveness during exercise was demonstrated in older men but blunted leg vasodilator responsiveness in older women
[58][80]. As indicated by prospective training studies, muscle sympathetic nerve activity is reduced after exercise training
[60][82].
Thus, changes in vascular conductance due to short-term training cessation and training (re)uptake may be involved in the difference in arteriovenous oxygen response to VO2max changes, whereas long-term training reduction/cessation may lead to more profound remodeling of capillarization and the loss of mitochondrial content and/or efficiency.
Angiogenesis occurs as a consequence of appropriate exercise stimuli in order to increase oxygen diffusion and to improve the removal of metabolites within the contracting muscles
[61][83]. Aging-related changes in the ultrastructure of the endothelium and the associated impairment of microcirculation are associated with a reduced CRF, but the adaptability of microcirculation to exercise stimuli seems to be maintained in old age
[62][63][84,85]. Aging-related mitochondrial dysfunctions
[64][86] and associated oxidative stress
[65][87] are well-established hallmarks of aging. Conversely, exercise has been shown to preserve mitochondrial health in skeletal muscle
[66][67][68][88,89,90] and slow down aging-related deterioration of antioxidative defense systems
[69][91].
Despite the scarcity of longitudinal studies on the CRF of masters athlete, the available data indicate that reduced oxygen delivery to working muscles, mainly due to diminished cardiac output (and possibly also due to maldistribution of cardiac output), plays a major role until late middle age, and a decline in skeletal muscle oxidative capacity, at least partly due to mitochondrial dysfunction, may become increasingly important in older age (i.e., above 70 years)
[70][92].
Fast (training cessation or (re)uptake) and slow (aging per se) components potentially modulating the VO2max decline in masters endurance athletes are schematically depicted in Figure 3.
Figure 3. Schematic representation of the slow (aging) and the fast (changes in training habits) components of aging in masters endurance athletes. (A) The red line represents the aging-related decline in VO2max of continuously active endurance athletes, and the lower (blue) line represents that of masters athletes who terminated their competitive activities at the age of 40 years. Detraining rapidly enhances the rate of VO2max decline in active athletes, and recovery of VO2max can rapidly be achieved after (re)uptake of training. (B) Decline in maximal cardiac output represents the main explanatory mechanism for both the loss and restoration of VO2max.