The advancements in power electronics have shown considerable improvement in satisfying the need for voltage stability and power quality improvement by introducing Flexible AC Transmission Systems (FACTS) technology. The main functions of these devices are reactive power compensation, voltage control, and power flow control to enhance better power quality in modern power systems.
1. Introduction
The first generation of FACTS devices were mechanically controlled capacitors, inductors, and phase-shifting transformers with mechanical on-load tap changers [1]. The second generation was developed such that thyristor valves replace the mechanical switches. This gave a significant improvement in the speed of the devices. The third generation was designed using voltage source converter (VSC) based devices [1]. These devices provide multiple and total control of the power system parameters [2]. To further extend the application of FACTS devices to a distribution network, there are custom power (CP) devices similar to FACTS devices, except that they are used only in distribution networks. An example is the distributed synchronous static compensators D-STATCOM [3]. The modification of FACTS to CP to be used in distributed networks can be considered as the foundation for the fourth generation of FACTS technologies. This advancement expands the application of FACTS controllers from being used only in transmission networks to deregulated CPPS networks.
2. Benefits of FACTS Technology in Power System
FACTS devices are used in power systems for economic and technical benefits. Most existing power systems are old and operate under full capacity due, amongst other things, to infrastructure or components worn-out. Building a new power grid or replacing existing components will be an expensive venture; FACTS devices are, therefore, used to optimize the system performance capacity at a lower cost. Furthermore, in a deregulated power system network and competitive market, FACTS devices can be used to maximize consumers’ social welfare and utility’s profit in the face of imbalance cost due to RES uncertainty
[4][5]. Moreover, when optimally placed, FACTS devices can reduce congestion, curtailment and price volatility
[6][7].
Research has shown that FACTS technologies can be used to tackle many power system quality and reliability challenges such as: optimizing line power transfer capacity and loadability
[8][9][10]; limiting short circuit currents
[11]; enhancing power system transient stability state and system security
[12][13][14]; compensate reactive power and load for optimal performance
[15]; reducing sub-synchronous resonance and enhancing system damping
[16][17]; and improving voltage stability and general power system quality
[18][19].
3. Classification of FACTS Controllers
According to Rath et al.
[6], FACTS controllers can be divided into four categories according to how they are connected: shunt controllers, series controllers, series-series controllers, and series-shunt controllers.
3.1. Series Controllers
Series Controllers inject voltage in series with the line. They are used to reduce the transfer reactance of a power line and hence increase transmission line capacity and improve system stability. Examples of series controllers are Thyristor Controlled Series Compensator (TCSC) and Static Synchronous Series Compensator (SSSC)
[20].
Figure 1 shows the structure and form of TCSC designed with two-directional thyristors.
Figure 1.
Schematic diagram of TCSC
.
While the TCSC can be modelled as a series impedance, the SSSC is a series voltage source
[20].
Figure 2 shows the Schematic diagram of SSSC designed based on solid-state voltage source converter and series connected to a transmission line through a transformer.
Figure 2.
Schematic diagram of SSSC
.
3.2. Shunt Controllers
Shunt controllers are mainly used in high voltage systems to improve voltage profiles by supplying reactive power as they inject current into the system at the point of connection. The shunt controller only provides or absorbs variable reactive power when the injected current is in the 900 phase with the line voltage. Any other phase relationship will also control real power as well. Static Var Compensator (SVC) and Static Synchronous Compensator (STATCOM) are such controllers
[22].
The SVC is designed with Thyristor Switched Capacitor (TSC) and Thyristor Controlled Reactor (TCR) or Thyristor Switched Reactor (TSR), as shown in
Figure 3. The TSR is used to absorb reactive power, while the TSC is used to supply reactive power
[23].
Figure 3.
Static Var Compensator
.
STATCOM is an advanced SVC designed with a VSC which has a Gate turn off thyristor and d.c capacitor linked with a transformer connected to a transmission line, as shown in
Figure 4. The conversion of dc input voltage into ac outputs to compensate for the real and reactive power of the system is performed by STATCOM
[23].
Figure 4.
Schematic diagram of STATCOM
.
3.3. Combined Series-Series Controllers
This is a combination of separate series controllers, which are coordinately controlled, in more than one transmission line system. It could also be a unified controller, in which series controllers provide independent series reactive compensation for each line and also transfer real power among the lines through the power link. Interline Power Flow Controller (IPFC)
[22].
IPFC has two series converters connected to two different transmission lines, as shown in Figure 5. It provides a very good power flow control for more than one transmission line, with each of the two SSSC giving series power addition for its own transmission line. The two converters are joined through a DC capacitor and attached to the AC network through transformers directly connected. By this, it not only provides reactive power addition, but also any of the converters can be manipulated to inject real power to the dc joint from its own Transmission line.
Figure 5.
Schematic diagram of IPFC.
3.4. Combined Series-Shunt Controllers
These controllers have combined shunt and series controllers, which have sophisticated control. A real power exchange can occur through their shared DC link when the shunt and series controllers are jointly used. The Unified power flow controller (UPFC) is a series-shunt controller. It is considered one of the most versatile and powerful FACTS devices in the power system today
[24]. It is primarily used for flexible control of powers for better voltage stability. It allows concurrent or independent control of these parameters with transfer from one control scheme to another in real-time.
Figure 6 depicts the schematic diagram of UPFC.
Figure 6.
Schematic diagram of UPFC
.
UPFC, as shown in Figure 6, is designed using STATCOM and SSSC linked together with a d.c. The converters are connected to the line with transformers. The unique combination of this device allows for flexibility of operation when connected to a power system network.
The dynamic flow controller (DFC) is a hybrid device combining a Phase Shifting Transformer (PST) and switched series compensator, such as TSC and TSR, as shown in Figure 7.
Figure 7.
Schematic diagram of DFC
.
3.5. The Merit of the Voltage Source Converter Based FACTS over Thyristor Controlled Devices
- They consist of voltage source converters designed with an insulated-gate bipolar transistor or integrated gate-commutated thyristor, making them capable of controlling their output voltage;
- With the voltage source converters, there is no risk of shunt or series resonant with the inductive line impedance that may initiate sub-synchronous oscillation;
- They can control their output voltage over the whole VA rating independent of the AC system parameters;
- They exchange controllable real power with AC system.
They consist of voltage source converters designed with an insulated-gate bipolar transistor or integrated gate-commutated thyristor, making them capable of controlling their output voltage;
-
With the voltage source converters, there is no risk of shunt or series resonant with the inductive line impedance that may initiate sub-synchronous oscillation;
-
They can control their output voltage over the whole VA rating independent of the AC system parameters;
-
They exchange controllable real power with AC system.
Figure 8 depicts an overview of major FACTS devices classified according to their connection and different generations.
Figure 8.
Overview of major FACTS devices.
3.6. Distribution-FACTS Controllers
D-FACTS technologies are advanced FACTS controllers mainly deployed in distribution networks. Due to the increasing introduction of DGs to the power grid, D-FACTS are becoming more promising than convectional FACTS devices, gaining more recent traction in power grid deployment
[26]. D-FACTS devices are smaller and, hence, less complex and cheaper than traditional FACTS devices
[27]. Beyond the merit of flexibility and cost-effectiveness, D-FACTS controllers are suitable for easy configuration with the sensing and communication system of the modern smart grid for advanced functionality. Therefore, D-FACTS devices are considered an alternative solution to the limitations of traditional FACTS devices.
The similarity of D-FACTS devices to other convection FACTS devices extends beyond functionality to technical configuration; hence, most D-FACTS devices assumed the name of the specific FACTS controller with a similar configuration. D- STATCOM, for instance, is designed to perform a similar function as STATCOM; therefore, the operational configuration and connection in the power grid are the same. Moreover, D-SSSC is the distribution version of the SSSC controller. Both are connected in series along power lines and provide dynamic control for power quality and security improvement.
Pinheiro et al.
[26] demonstrates an application of series VSC in distribution network for effective power flow under variable load disturbances. The study results show VSC as active tool for independent control and transfer of real and reactive powers between distribution feeders. On the other hand, the biggest challenge of using series converter in an electrical power system is its ability to make smart decisions or otherwise be removed and protected in case of a short circuit or power outage
[26]. An efficient coordination of voltage and reactive power in an automated distribution system using distribution SVC (D-SVC) alongside DGs was presented by Shaheen et al.
[28]. This integrated configuration not only achieved optimum distribution system operation and control, but also minimized losses and reduce emissions
[29]. D-FACTS can also be utilized to regulate the oscillatory stability of induction motors or synchronous generator
[30].
4. Research Trends Findings and Future Prospects
the result of a bibliometric analysis conducted using VOSviewer software with data extracted from Scopus shows an overlay visualization of keywords associated with FACTS devices and Cyber-Physical Power Systems (CPPS). The network of these keywords and their clusters reflect different directions of research trend in the application of FACTS devices.
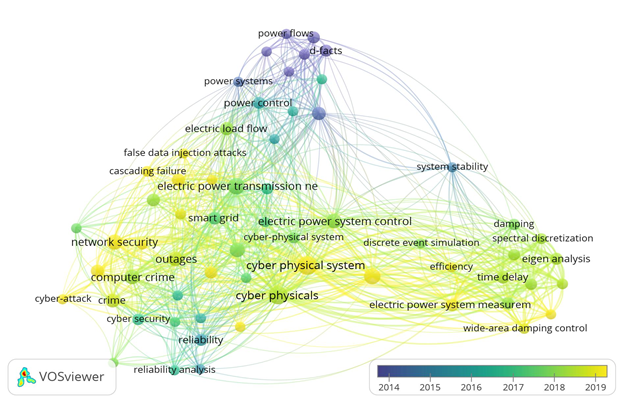
Figure 9. Overlay visualization showing research trend.
The following are key findings about the current application of FACTS devices and future utilization prospects.
- It is predicted that going into the future, say 2050, a very large amount of energy will be generated by RES while there will be a drastic reduction in fossil fuel power generation [31]. This implies that there will be more DGs penetration in power systems, bringing generation closer to distribution centers; hence D-FACTS will be more required than convectional FACTS devices. This is also considering the minimized advantage of D-FACTS devices over FACTS devices.
- CPPS of the future will be built with automated systems to include sensors, smart meters and communication systems to enable attributes such as self-control, self-optimizing and self-healing to guarantee autonomous power systems. Therefore, the future design of FACTS/D-FACTS devices must consider and appreciate interactions with the automated systems of CPPS to enhance effective integration. To this end, design modification of the operational configuration of FACTS/D-FACTS with sensors for real-time synchronized control and interaction with other CPPS technologies is an area that requires more research attention in the future.
- Cyberattack has been identified as the common most feared challenge of future CPPS as it has the potential of causing a total system breakdown and a worldwide blackout. Therefore, the new trend of research toward the use of D-FACTS in an MTD strategy against FDI must be expanded to improve power system security.
- In future, the advancement of optimal control capacity of FACT/D-FACT devices can be explored using cloud computing technology of the CPPS to store adequate data necessary to train the controllers with artificial intelligence required for dynamic control and protection of the system.
- Research and discussion about FACTS/D-FACTS have been extensive and stretch over a long time, but the main focus remained on their optimal location and operation. Extensive research on the actual cost of installing and operating FACTS/D-FACTS devices is limited in the literature. This area requires more detailed research to determine the exact economic implication of the use of FACTS/D-FACTS technologies. This has the potential to enhance proper power system planning in the future.
- Also, the real implementation or utilization of the FACTS/D-FACTS device is still very limited in several regions around the world. Countries that have them installed have only very few in their power grid. This low usage, especially in regions like Africa, is yet to be investigated. Few studies point towards limited production of these devices globally, but extensive research to ascertain the root cause leave room for further research.
- Since CPPS is consumer-centered, it will be interesting for demand-side management to be considered along with optimal placement and operation of DGs and D-FACTS devices in such a deregulated system. This possibly will enhance consumers' participation in microgrid planning and decision-making regarding power system infrastructure, especially considering economic implications.