Plant responses to nutritional aspects, as well as environmental considerations, are discussed. Nutrient deficiency impairs production, whereas over-fertilization may reduce yields and oil quality, and increase environmental hazards and production costs. The effect of irrigation on nutrient availability and uptake is very significant. Application of organic matter (e.g., manure, compost) and cover crops can serve as substitutes for mineral fertilization with additional benefits to soil properties. Recycling of the pruned orchard material, olive pomace and olive mill wastewater, as well as the use of recycled wastewater for irrigation, are all potentially beneficial to olive orchard sustainability, but present the risk of environmental pollution.
1. Nitrogen, Phosphorus, and Potassium in Olive Orchards
Intensification of olive orchards might very easily lead to over-fertilization and to environmental pollution. There are large differences in potential nutrient removal from olive orchards between extensive, non-irrigated vs. intensive, irrigated systems. Angelo-Rodriguez et al.
[1[1]1] ] measured nutrient removal from a traditional, non-irrigated orchard by both fruit and pruned material; annual amounts of removed N, P, and K were 9.7, 1.8, and 9.8 kg ha
−1, respectively, while local fertilization recommendations were more than 20-fold higher, up to 130 and 240 kg ha
−1 of N and K, respectively. Erel et al.
[3[2]]] estimated fertilization rates of N and K under intensive cultivation in Israel to be about 10-fold higher than the amounts removed by the fruit. If the pruned material forms roughly 50% of the total biomass (fruit and vegetative parts) removed from the orchard
[8[3]]], these fertilization rates are still fivefold higher than the total amounts removed from orchards by both fruit and vegetative material. In an experimental platform described by Haberman et al.
[14[4]]], control trees received annual rates of 150, 30, and 250 kg ha
−1 N, P, and K, respectively.
This fertilization efficiency is much higher than that described by Angelo-Rodriguez et al.
[11[1]]], probably due to the high efficiency of the fertigation system used to supply the nutrient needs of the trees. In the case of N, most of the unconsumed applied N will leach below the root zone
[9[5]] ] and part of it will reach the atmosphere as N
2O
[16[6]]]. In the case of K, most of the unconsumed K will find its way to the adsorbing complex of the soil, unless the cation-exchange capacity of the soil is very low, in which case it will also be leached below the root zone
[17[7],18][8]]]. Excessive soil K load may lead to soil dispersion and reduced infiltration rate, as described for sodicity
[19[9],20][10]]]. Unconsumed P will be partly fixed and remain in the upper soil layer, where it might be transported by water runoff and, consequently, translocated to undesirable locations such as water bodies
[21[11]]]. In fertigated (fertilized via the drip-irrigation system) orchards, P can also migrate below the root zone and find its way into groundwater, especially in very sandy or low pH soils. However, since olives are generally irrigated using a deficit-irrigation strategy, this risk is lower than for other crops.
2. Rain-Fed and Irrigated Olive Orchards
Olive cultivation occurs in two major and distinct systems: (i) Traditional, extensive, rain-fed orchards and (ii) modern, high-density, intensive, irrigated orchards. Most of the world’s olive orchards are rain-fed
[2[12]]] but their relative contribution to production is low
[4[13]]]. Plants have to be fertilized in both cultivation systems, but fertilization considerations and risks of potential environmental pollution differ for each. In rain-fed orchards, growth rates and yield levels are much lower than in irrigated systems
[22[14],23][15],24][16]]] and therefore, fertilizer application rates are generally lower than for irrigated orchards
[3[2],25][17]]]. In rain-fed orchards, water is very often the limiting factor for nutrient availability and uptake, since during the long dry summer, availability is reduced
[3[18],13][19]]. Timing of fertilization is unique for each category. In rain-fed orchards, fertilizer is applied to the soil, and the mobilization of nutrients to tree roots is highly dependent on rainfall events. In the Mediterranean climate typical of olive-cultivation regions, organic substances or mineral fertilizers are commonly applied in late winter or early spring, designed to take advantage of rainfall events to transport minerals into the root zone. Insufficient rainfall will leave nutrients out of reach of active roots, and excessive rainfall may lead to significant N losses by leaching or release of gaseous N forms (denitrification). Fertilization during the dry season is usually done by foliar application
[25[17],26][20]]]. Ferreira et al.
[27[21]]] found that repeated foliar applications of N can maintain adequate leaf N levels throughout the growing season. However, foliar spraying generally fails to meet the macro-element requirements of fruit trees
[28[22]]]. In an experiment carried out by Toscano et al.
[29[23]]], four annual foliar applications proved to be useful as a complementary activity to soil application, but could not satisfy the full nutritional requirement of the trees over the long run. Nutrient uptake from foliar applications is also affected by tree water status, such that the uptake by water-stressed trees is lower than that by non-stressed ones
[30[24],31][25]]]. In irrigated orchards, fertilizers are usually applied simultaneously with irrigation (fertigation)
[3[18]]], which enables better control of fertilization levels and timing and thus, may enhance fertilizer-use efficiency compared to broadcast application
[32[26],33][27]]]. The two different systems also imply differences in potential environmental effects. On the one hand, the amount of nutrients that need to be applied to rain-fed orchards is lower than for irrigated ones. On the other, the grower has less means of controlling the unused residual nutrients, which can contribute to environmental pollution.
3. Recycling of Olive Pomace and OMW
The olive oil extraction industry produces large amounts of olive pomace and OMW, which are potential environmental pollutants. Olive pomace is easier to handle than OMW, with various options for recycling, the most common being its incorporation in compost, together with organic manure and some bulking agents when required
[102[28]]]. Pomace can also be dried and burned with a high energetic value or serve as livestock feed after appropriate treatment
[103[29]]]. OMW is more difficult to handle. This byproduct, produced in large amounts over a relatively short time, cannot be introduced into sewage treatment plants shared with municipal or industrial players due its high biological oxygen demand (BOD), fat and polyphenol concentrations. In some cases, OMW is stored in large pools and left to dry in the sun until the solid leftovers can be incorporated into composts, but this approach requires large storage and evaporation ponds and tends to be an environmental nuisance due to unpleasant odors. The most common solution today is to spread OMW on the soil surface in olive orchards (
Figure 81), in limited amounts of 50–80 m
3 ha
−1 year
−1 [18[8]]]. Potential risks of in-situ orchard application of OMW include negative effects on soil characteristics
[104[30]]] or soil fauna due to phytotoxicity
[105[31]]] and transport out of the orchard and into natural water sources during erosion events. Nevertheless, recent studies
[17[8][32],18[8]]] have shown that controlled application of OMW to olive orchards over several years has no long-term adverse effects on soil properties or tree performance. Saadi et al.
[106[33]] ] found quick recovery of soil microbial activity after OMW application and recommended this procedure as safe. In addition, OMW can provide significant amounts of K and P to the soil and partially replace fertilization with these nutrients.
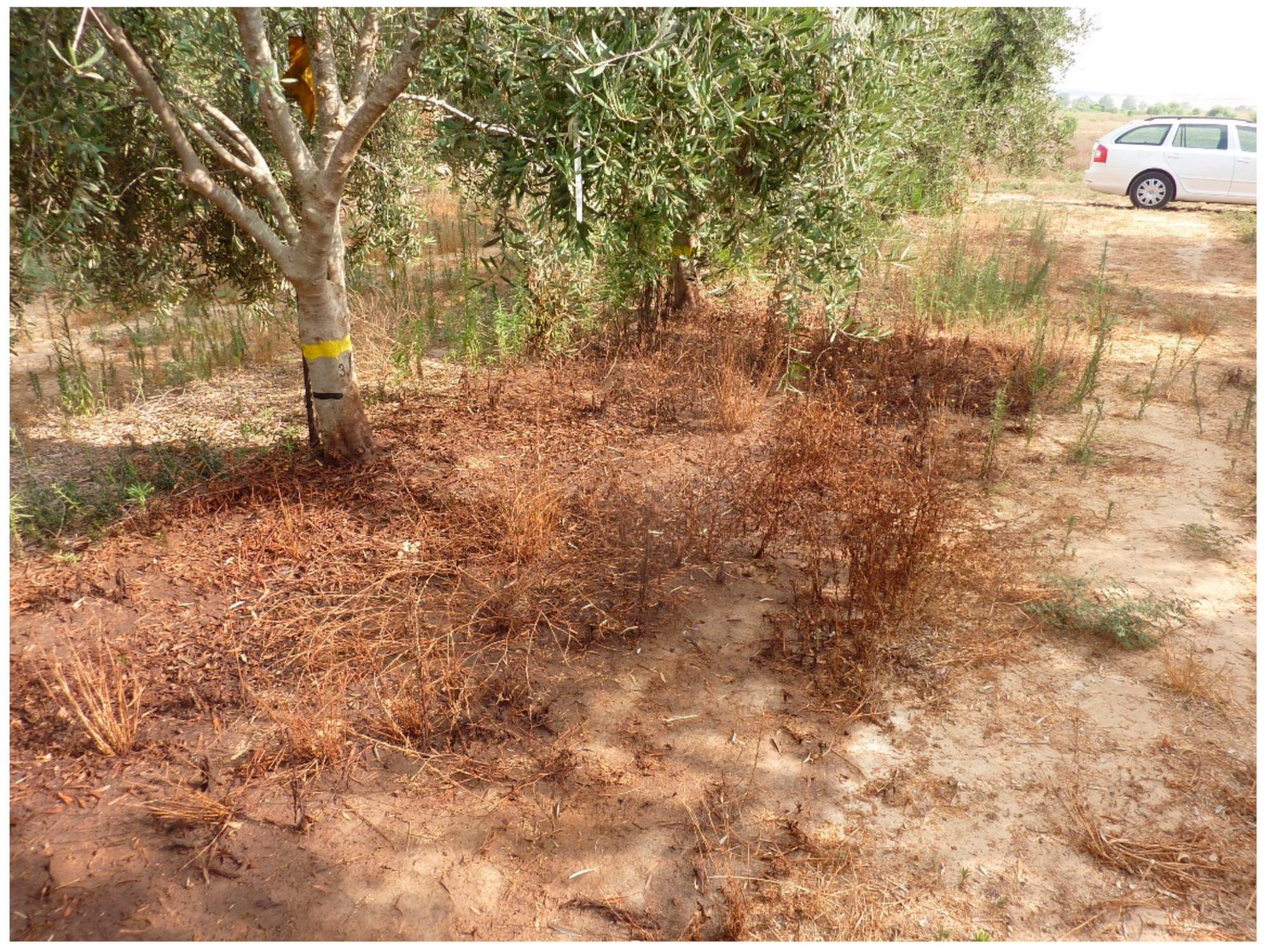
Figure 81. An olive plot, 6 months after olive mill wastewater (OMW) application (50 m
3 ha
−1). Gilat Research Center, Israel.
Zipori et al.
[18[8]] ] found that at an annual OMW application of 50 m
3 ha
−1, the whole K requirement and 30% of the P requirement of the orchard were satisfied, suggesting substantial savings on chemical fertilizers. Controlled application of OMW in olive orchards seems to be a sustainable practice, especially if it is followed by shallow tillage to reduce soil hydrophobicity and improve water infiltration
[17[32]]]. However, there is always the risk of a negative impact of OMW on soil properties. If an intensive olive orchard produces an average yield of 13 t ha
−1 year
−1 and the amount of OMW generated during the oil-extraction process is 1.5 m
3 t
−1 [106[33]]], then the total annual amount of OMW generated is 20 m
3 ha
−1. In most countries, the permissible annual amount of applied OMW is 50–80 m
3 ha
−1 [107[34]]]. This means that a given plot will be exposed to OMW once every 3–4 years, thus further reducing possible negative effects but being less effective in terms of nutrient supply and fertilizer replacement.
4. Irrigation with Recycled Wastewater as a Source of Nutrients
Global demand for water for domestic, industrial and agriculture uses is continuously rising, as a result of population growth and standard of living. Competition for high-quality water resources is prominent in water-scarce regions, where irrigation is essential to agricultural expansion and success. The need to treat and dispose of increasing quantities of sewage on the one hand, and the rising demand for irrigation water on the other, stress the importance of effective and sustainable use of recycled wastewater (RWW)
[108[35],109][36]]]. In regions where no fresh water is available for olive orchard cultivation, irrigation with RWW has been shown to provide a sustainable alternative and improve yields
[110[37]]].
While the use of RWW in agriculture can help meet the increasing requirement for water across the agricultural, domestic and industrial sectors
[111[38]]], irrigation with RWW carries both agronomic and environmental risks that require special consideration
[9[39]]]. On the positive side, use of RWW allows recycling of both water and nutrients that would otherwise be disposed of in the environment, subsequently contaminating natural water bodies. On the downside, RWW tends to contain high and potentially problematic concentrations of plant growth-inhibiting ions such as Na, B, and Cl
[112[40],113][41]]]. High concentration of Na in RWW, relative to those of Ca and Mg, has added potentially hazardous effects due to its contribution to elevated SAR values. Nevertheless, in an 8-year experiment
[114[42]]], no negative effect on tree performance was detected as a result of irrigation with RWW in comparison to irrigation with fresh water. Moreover, even when no fertilization was applied for 5 years, irrigation with RWW was sufficient to satisfy the nutritional requirements of the trees, as the annual amounts of N, P, and K supplied by the RWW alone were 124, 34, and 193 kg ha
−1, respectively. This calculation was based on an analysis of the soluble inorganic constituents of the RWW. However, RWW also contains significant amounts of organic matter. Mineralization rates of this organic matter and its effect on the soil microbiological population are difficult to assess quantitatively. When irrigating with RWW, under low levels of BOD and chemical oxygen demand (COD) (20 and 70 mg L
−1 O
2, respectively), soil microbiological composition and activity were not affected
[115[43]]]; under high BOD and COD levels (80 and 173 mg L
−1 O
2, respectively), both the composition and activity of the soil’s microbiological population were affected
[116[44]]]. The activity of ammonium-oxidizing bacteria was significantly affected even at the low BOD and COD levels
[115[43]]]. This elevated microbiological activity transforms organic N into mineral N and thus contributes even more to the potential N supply from RWW. Similar processes probably occur in the transformation of organic P into mineral P. The actual contribution of RWW to olive nutrition is site-specific, depending on the actual concentrations of the individual minerals in the water and the amount of water irrigated. Obviously, when irrigating with RWW, the contribution of nutrients delivered with the water to the trees has to be taken into account and subtracted from the fertilization scheme.
In a study on intensive hedgerow olive cultivation, irrigation with RWW caused salt accumulation during the summer due to the deficit-irrigation policy employed, but the salts were leached each year with the winter rains
[9[39],114][42]]]. A negative trend was identified for SAR, which increased slowly and steadily during the 8 years of the experiment in the plots irrigated with RWW, compared to plots irrigated with fresh water. Continuous irrigation with RWW might impair the soil’s physical properties
[110[37],114][42]] ] and measures, such as enrichment with Ca ions by liming, should be considered.