Figure 9. The schematic diagram of the side-polished POF.
Banerjee et al.
[98][35] described the experimental results on RI sensing by a low-cost plastic-cladded POF and a silica fiber with a plastic coating that forms a protective layer on the silica cladding. The cladding of these two fibers were stripped to different thickness to make the fibers sensitive to RI of the environment. The results showed that the sensitivity of the sensor to RI change was nonlinear and was dependent on cladding thickness, and the sensitivity would reach a maximum at an intermediate thickness value. L. Bilro et al.
[99][36] presented a cure monitoring system based on a side-polished interface on POF, and they also presented the modeling of a side-polished POF as a sensor for RI and curvature measurement by using a geometric optic approach
[100][37]. The model considered different details such as the geometric description of the sensor, the intensity profile of the emitter, and the possibility of a multireflection for a light ray at the sensitive area. Teng et al.
[101][38] investigated the RI sensing performances of the straight and macro-bending side-polished POFs. Results showed that the macro-bending probe had an enhanced RI sensing performance, and when the polished depth was 400 μm, the polished length was 10 mm and the curvature radius was 2 mm, and a sensitivity of 864%/RIU and a resolution of 3.3 × 10
−4/RIU with a standard deviation of 0.16 were obtained.
Feng et al.
[102][39] made a RI sensor based on a D-shaped POF, and different depths of the D-shaped groove and a different curvature radius of the fiber probe were used to research the influence for the sensor sensitivity. Experiment results showed that the proposed sensor had a good linear response for the measured RI ranging from 1.333 to 1.455, and the highest sensitivity of the sensor was obtained when the depth of the D-shaped fiber was 500 μm. They also simulated the energy distribution of the D-type structure by using the Finite Element Method
[103][40], and the experiment results showed that the normalized transmittance intensity decreased 13.4% with the RI increasing from 1.333 to 1.455 when the depth and length were 500 µm and 2 cm, respectively, with an excurvature radius of 5 cm and optical source wavelength of 652 nm.
In addition, Sequeira et al.
[104][41] reported the optimization of the length for a D-shaped POF sensor for RI sensing from a numerical and experimental point of view. Results showed that, in the RI range of 1.33–1.39, the sensitivity and the resolution of the sensor were strongly dependent on the sensing region length, and the highest sensitivity resolution of 6.48 × 10
−3 RIU was obtained with a 6 cm sensing length. While in the RI range of 1.41–1.47, the length of the sensing region was not a critical aspect to obtain the best resolution. Besides, Zhong et al.
[105][42] investigated the mechanism of the effect of heat treatments on physical and optical properties of D-shape POF-based EW sensors.
2.4. Grating Structure-Based RI Sensors
The fiber gratings usually refer to the fiber Bragg gratings (FBG), the long-period gratings (LPG), and the titled Bragg gratings, which are fabricated on the single-mode silica fibers
[106][43] or the single-mode polymer fibers
[48][44]. They often work on the wavelength modulation method. The grating structures introduced were fabricated on the multimode POF by a simple mechanical die press print method; due to the coupling of the core modes and cladding modes that occurs at all wavelengths, this type of fiber sensors work on the intensity modulation mode.
In 2017, Teng et al.
[107][45] proposed a POF with a multi-notched structure as a long-period grating for RI sensing. The structure was simply made on the surface of the fiber by pressing a thread rod against the POF as shown in
Figure 10. The RI sensing performances for straight and macro-bending POFs with this structure were studied. Results showed that the POF probes with straight multi-notched structures were not sensitive enough for RI measurement. After bending the multi-notched structure into U-shaped probes, the RI sensing performance was improved markedly. The highest sensitivity of 1130%/RIU with a resolution of 8.44 × 10
−4 RIU in the RI range of 1.333–1.410 was obtained.
Figure 10. The schematic diagrams of the fabrication process of multi-notched structure on POF, and the structure of multi-notched POF
[107][45].
In 2019, Xue et al.
[108][46] fabricated the LPGs on the POFs with different diameters and investigated their RI sensing performances. The results showed that a higher RI sensitivity can be obtained when the LPG structure was imprinted on a thin POF (with a diameter of 0.25 mm), and the optimum sensitivity of 2815%/RIU with a resolution of 1.39 × 10
−4 RIU was achieved in the RI range of 1.33–1.45 when the grating period, the groove depth, and the tilted angle were 100 µm, 65 µm, and 20
°, respectively. Later, the same group proposed a D-shaped POF assisted by an LPG structure for RI sensing
[109][47], as shown in
Figure 11. The results showed that the LPG structure could achieve an enhanced RI sensitivity. When this structure was fabricated on POF with a thin diameter of 0.25 mm, the high sensitivities of 2676 %/RIU and 9786 %/RIU could be obtained in the RI ranges of 1.33–1.40 and 1.40–1.45, respectively.
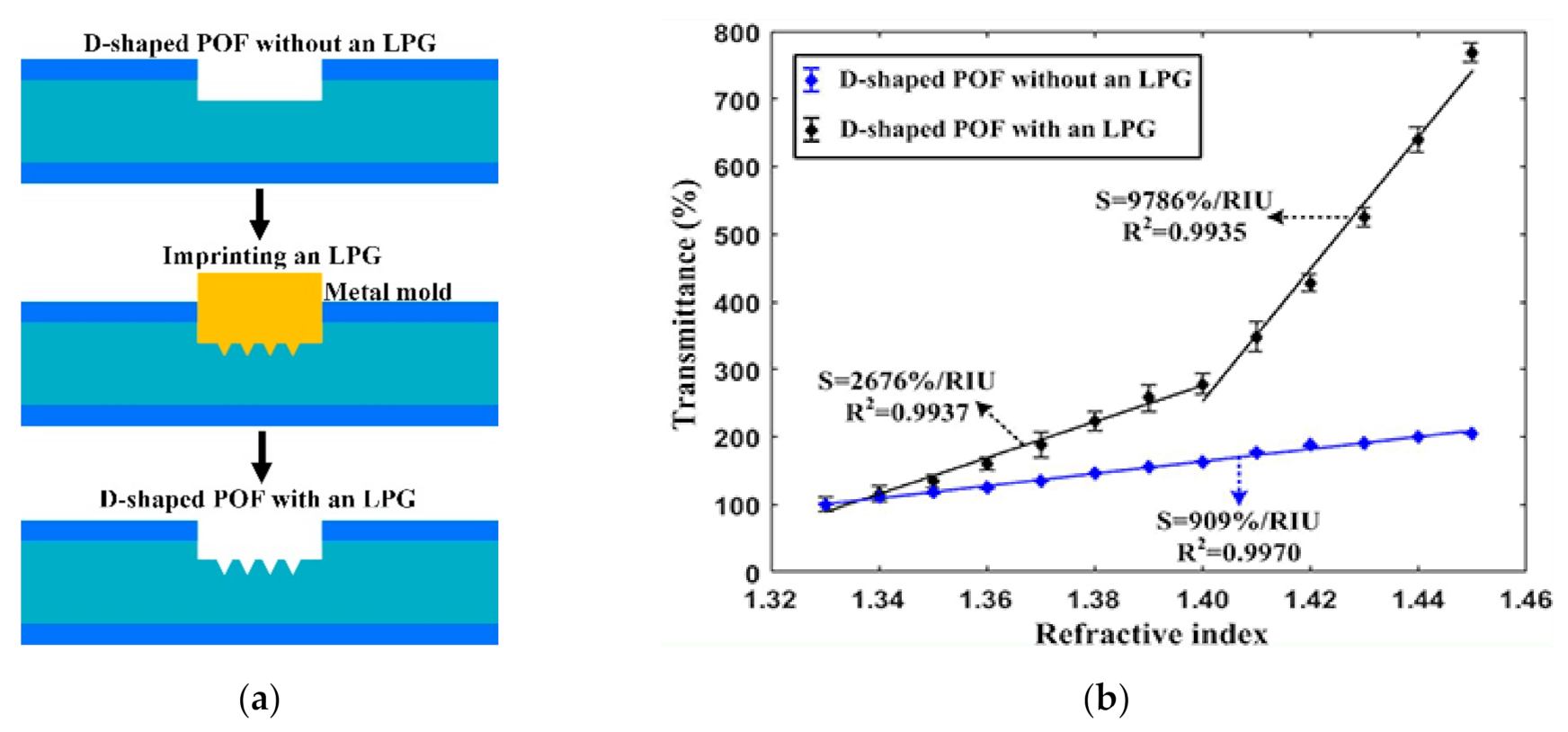
Figure 11. The schemes diagram of fabrication pross of LPG structure on POF (
a), and (
b) is the comparison of the experiment data for the D-shape POF probe with and without LPG structure
[108][46].
In addition, a screw-shaped POF was fabricated through a heat pressing and twisting method and proposed for RI sensing by this group, as shown in
Figure 12 [110][48]. This structure can lead to periodic coupling between core modes and cladding modes, which is similar to the working principle of LPG.
Figure 12. The schematic diagram of the screw-shaped POF
[110][48].