Enantiomers share the same chemical formula but have different chemical structures, i.e., type of isomers. Enantiomers are present in several drugs, perfumes, food, and are a fundamental part of biomolecules. This subject is highly important for pharmaceutical companies. Enantiomeric drugs present different actuation in the human body; depending on the compound, one might combat the symptom, whereas its pair might cause damage. The separation of pairs of enantiomers requires a chiral environment that provokes a structural imbalance that conventional methods cannot provide. Enantioresolution is one of the most promissory studies that benefit several areas, such as pharmaceutical, biotechnology, food industry, and fine chemistry. Its resolution is of great importance, therefore, its main mechanisms of resolution will be explained herein.
Isomers are different compounds that share the same chemical formula but have different chemical structures. They are classified into structural and stereoisomers. Structural isomers might be subclassified into function, chain, position, metamerism and tautomerism, as exemplified in Table 1, the latter only existing in equilibrium. Stereoisomers are subclassified into diastereomers (diastereoisomers) and enantiomers (optical isomers). Isomers have different physicochemical properties such as their melting point, boiling point, solubility, and density. Enantiomers, unlike other isomers, share all these properties, but optical activity. Enantiomers are analogous to a pair of hands, an enantiomer is a specular image of the other.
Table 1. Types of isomers and their structural formula
[1].
Isomerism |
Chemical Formula |
Structural Formula |
Structural |
Function |
C3H6O |
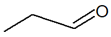 |
 |
Propanal |
Propanone |
Chain |
C4H10 |
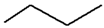 |
 |
n-Butane |
Isobutane |
Position |
C5H10O |
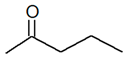 |
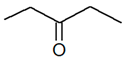 |
2-Pentanone |
3-Pentanone |
Metamerism |
C4H10O |
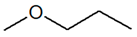 |
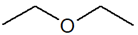 |
1-Methoxypropane |
Ethoxyethane |
Tautomerism |
C6H6O |
 |
 |
 |
Oxepin |
Benzene oxide |
Stereo |
Diastereomer (Geometric) |
C2H2Cl2 |
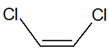 |
 |
cis-1,2-dichloroethene |
trans-1,2-dichloroethene |
Enantiomer (Optical) |
C3H6O3 |
 |
 |
(S)-Lactic acid |
(R)-Lactic acid |
Enantiomerism is present in several drugs, perfumes, food, and even in our own body. The response of human organisms to certain enantiomeric compounds might change dramatically depending on the enantiomer: one enantiomer in a drug might treat the disease whereas the other might cause harmful side effect. Thalidomide’s side effect is, perhaps, the most remarkable and unfortunate known case in the literature. It is a drug that was used by pregnant women in the 1960’s in the United Kingdom in order to combat morning sickness. However, the drug caused several birth defects
[1]. This drug was sold in an equimolar mixture of enantiomer in which only one of them combats the symptom (eutomer), and the other caused side effects (deutomer)
[2].
Large pharmaceutical companies aim to synthesize drugs that are quickly able to combat symptoms or illnesses with the least number of side effects by removing the deutomer. For this reason, enantioresolution is a crucial issue in the pharmaceutical industry. Distillation, decantation, and filtration are common methods of separation for compounds based on boiling point, density, and size, respectively. The resolution of compounds with different physicochemical properties might be simpler in comparison to enantioresolution. The separation of pairs of enantiomers, though, is not straightforward, and conventional methods are nearly ineffective. Crystallization, membrane, or chromatography are examples of processes used to separate enantiomers in a chiral environment, molecules that tend to bind to an enantiomer instead of the other
[3].
Optical activity is the phenomenon of shifting in the direction of the light plane when it passes through a compound. Light is an electromagnetic radiation that, when interacting with electrons in a molecule, slightly diverts its direction. Some molecules, though, do not present optical activity, i.e., for any light shift, either to the left or right, there is a molecule that shifts it in the opposite direction, nulling the optical activity. On the other hand, when light passes through pure there is a shift of the light; when light shifts to left, the enantiomer is called levorotatory (receives the prefix or (−)) and to the right it is called dextrorotatory (receives the prefix or (+)).
A polarimeter is the instrument responsible for reading the light rotation in degrees of a certain enantiomer concentration. This rotation must be converted into a specific rotation to take in account the polarimeter length and sample concentration, as given by:
where is the specific rotation, superscript designates the temperature (usually 20 °C), superscript the light wavelength (usually at 589.6 nm of Na D-lines), is the polarimeter length in dm, is the enantiomer concentration in g·cm−3, and is the observed rotation in degrees in the polarimeter. It means that an enantiomer has a negative specific rotation, a enantiomer a positive one, and a racemate has a
where of zero.
is the specific rotation, superscript T designates the temperature (usually 20 °C), superscript λ the light wavelength (usually at 589.6 nm of Na D-lines), lpol is the polarimeter length in dm, c is the enantiomer concentration in g·cm−3, and α is the observed rotation in degrees in the polarimeter. It means that an l enantiomer has a negative specific rotation, a d enantiomer a positive one, and a racemate has a [α]Tλ of zero.
A pair of enantiomers does not overlap by either transposition or rotation. The non-overlapping of molecules is known in chemistry as chirality and these compounds are therefore called chirals (from the Greek word χέριa [quéria], meaning hands). In order to be considered chiral, the molecule must have at least one tetrahedral carbon bound to four different groups, as shown in
Figure 1. This carbon is known as chiral or asymmetric carbon
[4]. There is also the possibility of chirality involving other atoms such as silicon, nitrogen, sulfur and phosphorus, coordination complexes, allenes and atropisomers, as shown in
Figure 2; however, these are less common
[5].
Figure 1. Chiral carbon (*) bound to four different groups in different representations.
Figure 2. Pairs of enantiomers of (a) chiral silicon, (b) chiral nitrogen, (c) chiral sulfur, (d) chiral phosphorus, (e) cobalt complex, (f) allenes and (g) atropisomers.
where and are the number of enantiomers and chiral carbons, respectively, as exemplified in Figure 3. However, this correlation is not always true; molecules with at least two chiral carbons that present a plane of symmetry are not chiral molecules. One of its carbons shifts the light plan to left, whereas the other nulls its effect, an internal compensation. An example is illustrated in Figure 4, although the tartaric acid has two chiral carbons, it has only two enantiomers and one meso isomer, a diastereomer and a non-optically active stereoisomer (see Table 1).
Figure 3. Number of enantiomers related to the numbers of chiral carbons (*) for (a) lactic acid and (b) isoleucine.
Figure 4. Tartaric acid presents (a) two enantiomers and (b) a meso isomer.