The proteasome system is a large and complex molecular machinery responsible for the degradation of misfolded, damaged, and redundant cellular proteins. When proteasome function is impaired, unwanted proteins accumulate, which can lead to several diseases including age-related and neurodegenerative diseases. Enhancing proteasome-mediated substrate degradation with small molecules may therefore be a valuable strategy for the treatment of various neurodegenerative diseases such as Parkinson’s, Alzheimer’s, and Huntington’s diseases.
1. Introduction
The degradation of proteins is a continual process that is highly regulated by the two major proteolysis systems, the lysosomal degradation pathway and the proteasome-mediated pathway. Protein degradation helps maintain biological homeostasis in cells which are needed for all cell functions and for maintaining optimal conditions for enzyme function
[1]. The proteasome pathway is the major pathway for the degradation of misfolded, oxidatively damaged, and redundant proteins. Dysregulation of proteasome function has been identified in the pathogenesis of several neurodegenerative diseases including Parkinson’s disease (PD)
[2], Alzheimer’s disease (AD), and other neurodegenerative diseases
[3]. The proteasome pathway is also involved in the regulation of several other cellular processes such as cell cycle, stress signaling, gene expression regulation, inflammatory response, cell differentiation, and apoptosis, which makes it an appealing target in the treatment of other types of diseases, including cancer
[4]. Due to the critical role of the proteasome-mediated degradation pathway in cell regulation, the modulation of proteasome proteolytic activity has become a valuable strategy in the pursuit of new therapeutics to treat several neurodegenerative diseases
[5][6][7][8].
1.1. The Human Proteasome
The human proteasome is a large complex protein responsible for the intracellular degradation of unwanted and damaged proteins via a ubiquitin-dependent and ubiquitin-independent degradation pathway. The most common proteolytic clearance of proteins proceeds by tagging the protein with polyubiquitin, after which it is degraded into small peptides of seven to eight amino acids by the 26S proteasome
[9]. Highly disordered proteins can also be degraded in a ubiquitin-independent manner by the 20S proteasome
[10].
1.2. Ubiquitin-Proteasome System
1.2.1. Ubiquitin
Ubiquitin (Ub) is a small protein (approximately 8600 Da) with 76 amino acid residues responsible for tagging a wide range of cellular proteins for proteolytic degradation. In the ubiquitin-proteasome system (UPS) (
Figure 1), proteins are tagged for proteolysis by covalent ligation to ubiquitin
[11]. Ubiquitination of proteins requires three enzymes in chronological order (see
Figure 1a). The E1 ubiquitin-activating enzyme, just like its name, activates the C-terminal glycine residue of the ubiquitin in an ATP-dependent manner. The binding of the ubiquitin to a cysteine residue of E1 forms a Ub-E1 complex via a thioester linkage. The E2 ubiquitin-conjugating enzymes transfer the ubiquitin from the Ub-E1 complex to itself via a trans-thioesterification to form the Ub-E2 complex and release the E1 enzyme from the system. Lastly, the ubiquitin ligases E3s are responsible for selecting proteins for ubiquitin-mediated proteolysis. Humans have two E1 enzymes, about 40 E2 enzymes, and are estimated to have about 500–1000 E3s
[12].
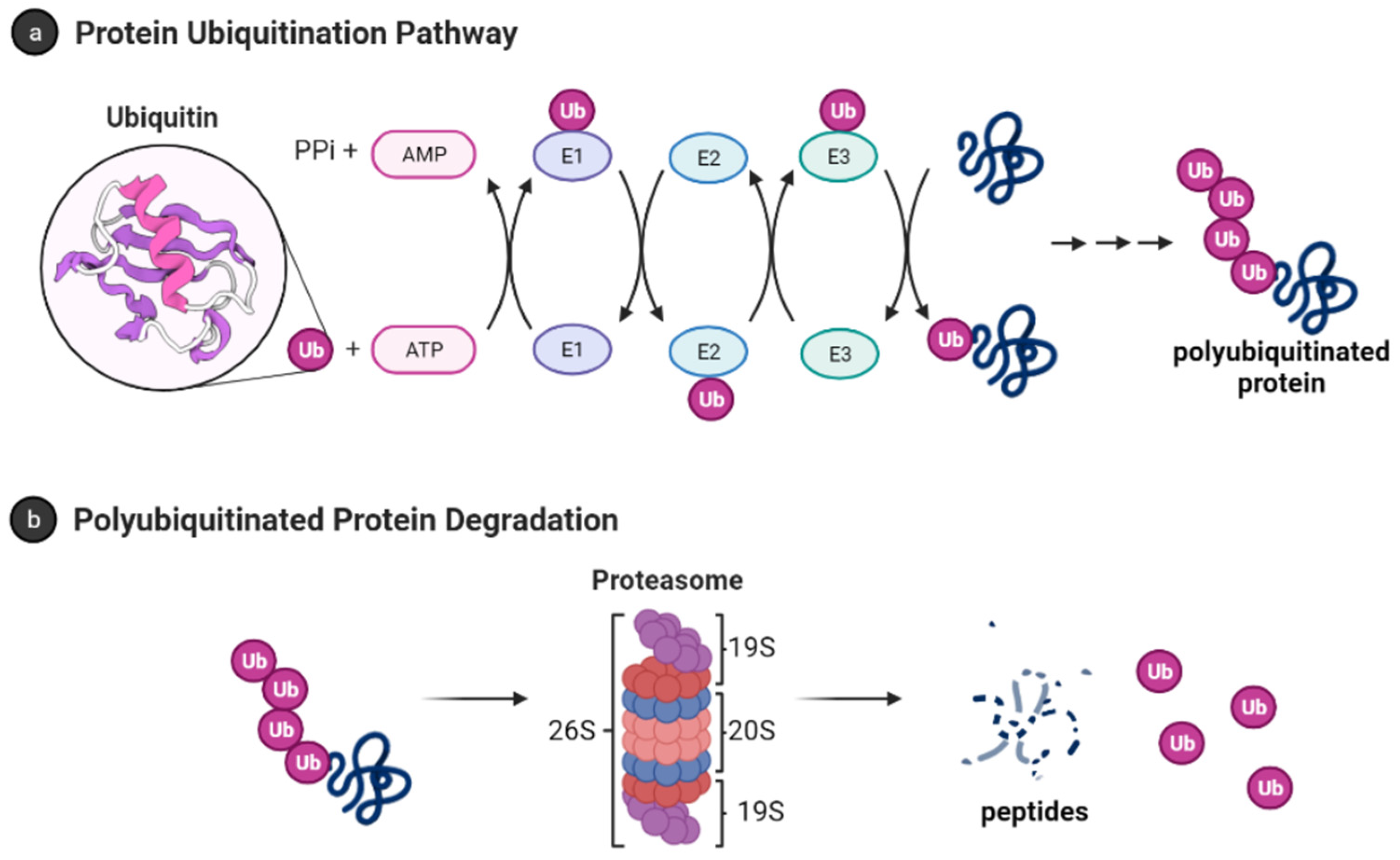