Table 1. Main biopolymers with their origins and chemical structures
[16].
Biopolymers |
Sources |
Structure |
Reference (Ref.) |
Chitin |
Corals, horseshoe worms, lamp shells, sponges, squid, cuttlefish, and clams are examples of aquatic species |
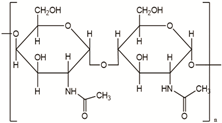 |
[17][18] |
Chitosan |
Fungi, mollusks, algae, crustaceans, and insects |
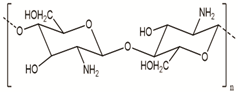 |
[17][19] |
Cellulose |
Agricultural trashes, such as Seaweed, rice husk, and sugarcane bagasse. Plant sources like wood, bamboo, sugarbeet, banana rachis, potato
tubers, cotton, fique, kapok, agave, jute, kenaf, flax, hemp, vine, sisal, coconut, grass, wheat, rice, and barley |
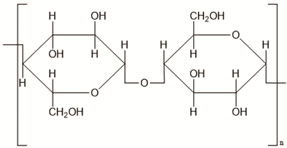 |
[20] |
Alginate |
Seawood |
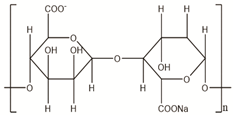 |
[21] |
Starch |
Potatoes, maize, cassava, rice, sorghum, banana wheat, yams |
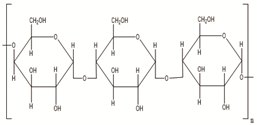 |
[22] |
Cyclodextrin |
Starch sources like tapioca, potato, wheat, rice, and corn |
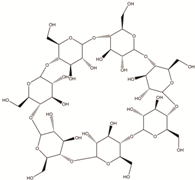 |
[23] |
Polycaprolactone |
Polycondensation of ε
-caprolactone |
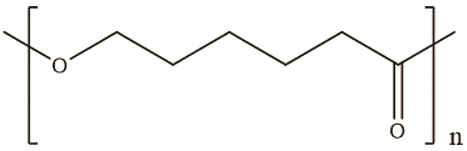 |
[24] |
2. Biopolymers for Medical Applications
Science and technology are essential factors in extending life expectancy. In this regard, various creative approaches and new equipment have been created, resulting in lower morbidity and death rates. The utilisation of drug delivery methods to increase the effectiveness of bioactive molecules is an essential technique for treating illnesses, and development in this area has been substantial. Synthetic, semi-synthetic, and natural polymers are commonly employed in the development of drug delivery systems in this context
[25]. Suturing, fixing, adhesion, covering, occlusion, isolation, contact inhibition, cell proliferation, tissue guiding, and controlled drug administration
[26][27][28][29] are just some of the medicinal applications that biopolymers could be used for. Several biomaterial production techniques and processes have been developed in recent decades to address and examine natural tissues’ important functional architectural and compositional characteristics
[30]. The hunt for better and more tissue-oriented implantable units has increased the understanding of biomaterials’ potential and heightened interest in multimodal scaffolds with unique shapes and physical-chemical properties
[31]. These scaffolds have multifunctional or multimodal qualities due to the integration of diverse topographies not generally present in each material, which boosts their potential relevance in regenerative medical techniques
[32]. Polymers have a critical role in developing three-dimensional templates and the creation of synthetic extracellular matrix (ECM) habitats for tissue regeneration
[33]. Biopolymers could be synthesised synthetically or from natural assets
[34]. A range of composite materials and interpenetrating networks have been used to attain the required objectives since each group has specific benefits and limits. Due to the adaptability of synthetic polymers, which enables them to be adjusted to a broad range of degradation efficiency, structural traits, and mechanical characteristics, they are a reliable source of innovative materials. The synthetic polymer composition may be tailored to reduce immune response and combine the most significant features. Owing to its superior biodegradability and lack of cytotoxicity, natural polymers have been proposed as a viable alternative to commonly utilised synthetic materials. They are derived from natural sources, resemble soft tissues, and are formed through enzyme-catalysed chain-growth polymerisation processes of activated monomers, often created within cells during metabolic activities. Collagen, gelatine, dextran, agarose/alginate, hyaluronic acid, cellulose, and fibrin gels are all included in this category (
Table 2). Despite being cleansed to prevent a foreign body reaction after implantation, natural polymers are commonly employed in regenerative medicine. Biodegradable polymers were chosen for the drug delivery system because they do not need surgery to remove once the medications have been released and may be excreted by the body. Some of the famous examples of biomedical applications that use biopolymers include soft-tissue replacement vascular grafts, breast implants, intraocular lenses, artificial hearts, components of extracorporeal oxygenators, contact lenses, plasmapheresis units, sutures, adhesives, and blood substitutes, dialyzers, liver, pancreas, bladder, kidney, bone cement, catheters, external and internal ear repairs, coatings for pharmaceutical tablets and capsules, cardiac assist devices, implantable pumps, pacemaker, encapsulations, heart valves, artificial blood vessels, joint replacements artificial skin, dentistry, drug delivery, and targeting sites of tumors or inflammation
[35][36][37][38]. Since PHAs are biodegradable, they can be used as a substitute for petrochemical plastics in biomedical applications. The adaptability of poly (3-hydroxyoctanoate) in particular makes it a potentially appropriate choice as a biopolymer for drug delivery formulation as well as features of prospective tissue engineering
[39].
Table 2. Examples of some biopolymers with their medical application.
Biopolymer |
Medical Application |
Ref. |
Collagen |
Surface coating for tissue culture plates |
[40] |
|
Simple gels for cell culture |
|
Alginate |
Regenerative medicine |
[41] |
|
Tissue engineering |
|
Hyaluronic acid |
Treatment and lubrication of damaged joints |
[42] |
|
Cutaneous and corneal wound healing |
|
Fibrin |
Blood clotting, wound healing, and tumor growth |
[43] |
|
Hemostatic agent, sealant, and surgical glue |
|
Silk fibroin |
Regenerative medicine
Treatment of wounds,
bioengineering of tissues |
[44] |
Agarose |
Skeletal tissues regeneration,
kidney and fibroblast encapsulation |
[45] |
Carrageenan |
Skeletal tissues regeneration,
cell delivery system |
[46] |
Fibronectin |
Wound healing,
cardiac repair, bone regeneration |
[47] |
PHAs |
Drug delivery systems,
one tissue regeneration, |
[48] |
Elastin |
soft-tissue reconstruction,
orthopedics and cell encapsulation |
[49] |
Keratin |
Cornea tissue engineering,
skin regeneration |
[50] |
Starch |
Bone and cartilage regeneration,
spinal cord injury treatment |
[51] |
Many natural biomaterials based on biopolymers have been studied for hard and soft-tissue repair. They can be utilised independently or with other synthetic or inorganic components. The specific dressing, nursing care, and decreased grafting time are the key features of these tissue-engineered materials. Many recent in vivo studies contributed to the FDA clearance of innovative biomaterials for clinical use based on natural biopolymers as matrices for cell distribution and scaffolds for cell-free support of native tissues, despite their mechanical fragility and high cost. For cell encapsulation, a variety of naturally occurring and manufactured biopolymers that might be synthesised into diverse physical forms and geometries are used. The biomaterial component of these therapies must offer suitable mass transport characteristics, membranes, or scaffold stability, and acceptable cellular interactions depending on the site and intended function of the implant. Intelligent biopolymer hydrogels that vary their swelling behaviour and other features in response to chemical and physical stimuli, including pH, metabolites or/and ionic variables, temperature, and electric fields, have piqued curiosity. These “smart” hydrogels exhibit single or multiple stimuli-responsive properties. They may be used in a variety of biomedical applications, varying from cell adhesion mediators and controlled drug delivery systems to controllers of gene expression and enzyme function in bioengineering or tissue engineering, in addition to their biocompatibility, biodegradability, and biological processes. Biopolymers may be readily functionalized to improve cell interactions and provide an appropriate platform for cellular and tissue functions. There are two types of peptide-based biopolymers in tissue engineering: self-assembling polypeptides that form gels in response to environmental cues, and polypeptides that create gels by chemical cross-linking. The biopolymer block’s unique bioactivity, biocompatibility, and biodegradability properties enable it to be used in various biomedical applications
[52]. Polymer–biopolymer interactions are becoming more and more capable of being planned and selected, allowing for their intervention in cellular dysfunctions and developing more effective, targeted, and efficacious therapeutics. Due to the degree of control possible via live polymerisation techniques, previously available structure-function connections for bio-macromolecules may now be inferred for fully synthetic materials. Simultaneously, the production and activity of biopolymers may be controlled using molecular biology techniques. Because the ability to control the structure of polymers results in the power to modify their functioning, this synthesis of natural and synthetic macromolecular chemistry inevitably results in biomedical applications. A few examples of the medical application of biopolymers have been presented in
Table 2. Bio-printers can automate the assembly process and allow for complex biopolymer manipulation—from the macromolecular to the living cell level—to achieve architectural and biochemical complexity never before possible, resulting in tissue and organ substitutes that closely resemble their natural counterparts. These many regenerative medicine approaches are likely to transition from ‘bench to bedside’ in the future.
The potential of biodegradable nanoparticles has been explored by various researchers for a wide range of biomedical applications due to their properties, such as biocompatibility and bio-safety. Depending on their size, nature, and design, various nanoparticles have distinct therapeutic efficacy, bioavailability, and delivery components. Nanotechnology-assisted imaging is advantageous not only in the field of cancer but also in the field of cardiovascular disease
[53][54]. Micelles are helpful in cancer treatment by focusing on malignant cells using tumor molecular probes
[55].
2.1. Biomedical Applications of Protein-Based Biopolymers
Protein-based biopolymers play a vital role in medical applications. Biopolymers have biocompatible, non-toxic, and biodegradable properties. Their application to implantable devices is expanding rapidly and has great potential
[56]. As the molecular sizes of proteins are governed by their secondary structures, the production of nanoparticles proteins permits the formation of precisely constructed nanoparticles
[57].
Regenerative tissue engineering aims to use the body’s inherent healing potential to improve injured or damaged tissue function. Synthetic polymers and biopolymers are used as scaffolding to establish a favorable environment for cell proliferation and tissue repair. Collagen, keratin, gelatine, sericin, and fibroin are used to make films, pickering emulsions, hydrogels, nanogels, nanofibers, linked porous scaffolds, and 3D-printed scaffolds
[29][58][59][60][61][62][63]. Tissue engineering and therapeutics molecules (gene delivery, drug delivery, protein delivery) are delivered via collagen-based nanospheres, nanoparticles, sponges, electrospun fiber, and hydrogel
[64].
Gelatine is a non-toxic, biodegradable, and FDA-approved substance. Acid (type A gelatine) or essential (type B gelatine) hydrolysis is used to extract from bovine or porcine sources. Gelatine has been extracted from a variety of sources, including jellyfish, fins, bones, and sea urchins
[65]. Gel formation, thickening, emulsification, and foaming are characteristics of gelatine
[66].
2.2. Chitosan in Medical Applications
Chitosan is also widely used in medical uses because of its biodegradable and biocompatible properties. It has a variety of uses (gels, films, particles, membranes, or scaffolding, etc.) from biomedical to industrial fields. In addition, due to its properties, chitosan plays an essential role in cell attachment and growth. It is widely used as a tissue engineering matrix when manufactured in a porous structure. The main uses of chitosan in implants are bone, ligament, cartilage, tendon, liver, nerve, stent, and skin regeneration. Several examples of chitosan-based matrices for bone applications have been reported. However, due to its mechanical weakness
[67], there is a need to combine chitosan with other materials in applications for bone tissue engineering
[68]. Nanoparticles formed using chitosan display interesting properties, such as low toxicity; good absorbability, permeability, and moisture retention; and is easily degradable. However, they suffer from poor long-term stability and are very sensitive to environmental temperature and pH, which affects the degradation rate
[69].