1. Introduction
Zika virus (ZIKV) was initially isolated from a rhesus macaque in the Zika forest of Uganda in 1947
[1]. It is an arbovirus from the
Flaviviridae family and, together with other Flaviviruses like dengue (DENV) and yellow fever (YFV), poses as one of the major public health problems in Latin America. Other important flaviviruses of public health concerns include West Nile virus (WNV) and Japanese encephalitis virus (JEV), which together with DENV and ZIKV, are considered emerging tropical viruses. ZIKV is transmitted by the female
Aedes aegypti or
Aedes Albopictus mosquito, both being widely distributed in Latin America
[2]. However, other routes of transmission are also described including blood transfusion, sexual transmission, and transmission via breast milk
[3][4][5][3,4,5].
Sporadic reports of natural Zika and/or serologic evidence of ZIKV infection have been reported since its discovery
[6]. The first major outbreak of ZIKV was reported from the Island of Yap in Micronesia in 2007 where it was estimated that 72.6% of the population ≥3 years of age was infected, demonstrating the rapid transmission of ZIKV in a naïve population
[7]. A second major outbreak occurred in French Polynesia from October of 2013 through early 2014, when it was estimated that 28,000 ZIKV infections occurred (~11% of the population)
[8].
ZIKV began to circulate in Latin America between 2013 and 2014. In March of 2014, Chilean public health authorities confirmed that ZIKV infection was detected in cases reported in February, concurrent with the circulation of the virus in French Polynesia
[9]. Indeed, the strain of ZIKV circulating in Latin America from 2014 to 2016 is related to the French Polynesia strain, which is estimated to have arrived in Latin America in 2013
[10]. Clinical cases of ZIKV started to be reported in Brazil in October 2014 after cases of disease presenting with low-grade fever, exanthema, pruritus, arthralgia, and limb edema tested negative for dengue, yellow fever, measles, rubella, enterovirus and chikungunya in Rio Grande do Norte state. After cases were also reported in Bahia state, the identification of ZIKV as the aetiological agent of the new disease was confirmed in May of 2015
[11]. In response, PAHO issued an epidemiological alert of ZIKV infection with recommendations for clinical management and prevention and control measures. The Brazilian Ministry of Health started to receive notification of increased frequencies of microcephaly in areas where ZIKV was circulating and an epidemiological investigation was started
[12]. In December of 2015, PAHO, together with the Brazilian Ministry of Health, recognized the epidemiological association between ZIKV infection in pregnant women and microcephaly in newborns and released another epidemiological alert
[13]. After confirmation of ZIKV-induced microcephaly, the WHO issued a Public Health Emergency of International Concern (PHEIC) on 1 February 2016, attracting greater attention and scientific resources for this epidemic. Anecdotal evidence of ZIKV and microcephaly began appearing in the literature in January of 2016
[14], and was confirmed by subsequent stronger epidemiological and virological evidence
[15][16][15,16].
Several seroprevalence studies have shown that Zika incidence may have reached up to 70–80% of the population in Latin American countries and that its introduction was silent, especially when introduced in dengue-endemic regions. Recently, a new immunological survey of undergraduate students using humoral and cellular tests has identified ZIKV-positivity in more than 80% of samples that could at least partially differentiate DENV and ZIKV infections
[17]. These numbers are not far from those reported in Brazil, where a serological survey estimated that ZIKV seroprevalence exceeded 60% in Salvador (state of Bahia)
[18]. Likewise, the prevalence of flavivirus infections was estimated to be around 92% in the state of Ceará in 2018, of which only 37% were considered to be associated with DENV
[19].
ZIKV has been reported in 87 countries with autochthonous transmission of the virus in the Americas, the Caribbean, Asia and Africa
[20]. However, since the Latin American peak of infections in 2016, the number of reported cases has decreased dramatically. While in 2016, Brazil alone reported over 273,000 Zika cases to PAHO, in 2017 the number of cases dropped to 31,000. Since, it has varied from 18,000 to 31,000 cases per year, showing signs of stabilization
[21]. Unfortunately, this means that ZIKV is still circulating and is probably under-reported. Nevertheless, Zika is still causing the devastating effects of Congenital Zika Syndrome (CZS). A serological survey in Recife (Pernambuco, Brazil), the epicenter of CZS, performed in 2018, estimated that while the prevalence of anti-ZIKV IgM in pregnant women in the general population was around 1.6%, therefore higher than expected for official case numbers, ZIKV-associated complicated pregnancies was around 7%
[22], suggesting that health policies to address congenital Zika are still very relevant.
2. Implications of Flaviviruses Immunity on ZIKV Vaccine Development
The interaction of ZIKV immunity with other flaviviruses, and vice versa, may have important implications for vaccine development. The challenges for DENV vaccine development are examples of how difficult it might be to develop a vaccine for ZIKV. A vaccine for DENV must concomitantly induce homotypic neutralizing antibodies to each of the serotypes. If not, partial immunity against one of the serotypes with the presence of non-neutralizing antibodies may increase the risk of ADE and severe dengue. This happened to CYD (Denvaxia©), the dengue vaccine of Sanofi Pasteur, which induced antibodies that were not balanced between the four serotypes in individuals who were not previously exposed to natural DENV infection
[23][97].
CYD tetravalent vaccine is a vaccine combination of four recombinant viral vector vaccines using yellow fever 17DD structure to express the structural proteins (prM and E proteins) of each DENV serotype
[24][98]. This vaccine was able to induce neutralizing antibodies against the four DENV serotypes in more than 90% of vaccinees
[25][99]; however, homotypic neutralizing antibody against DENV-4 component of the vaccine was dominant
[23][97]. The DENV-1 and DENV-2 components were poorly infectious and the majority of DENV-1 and DENV-2 antibodies induced by the vaccine were heterotypic
[23][26][97,100]. The vaccine induced some levels of IFNγ-producing DENV-specific CD4+ T cells directed to DENV E protein
[24][98] and possibly to boost, by cross-reactivity between YFV and DENV antigens, some preexisting levels of DENV NS3 T cells
[27][101]. Despite the capacity to induce neutralizing antibodies and IFNγ against DENV
[28][29][102,103], CYD did not perform well in in phase III clinical efficacy trials. CYD displayed a general efficacy of 50%, 35–42%, 74–78% and 75–77% against DENV 1, 2, 3 and 4, respectively
[25][30][31][99,104,105]. Its protection was better in the dengue-experienced population, in which it reached 70% efficacy, but only 35% in dengue-naïve individuals
[30][104]. Unfortunately, an excess number of dengue hospitalizations occurred in year 3 of the trial in those volunteers who received the CYD vaccine compared to those who received a placebo in the first year of the trial. This was observed more frequently in 9-year-old children and below
[32][106]. Detailed analysis of CYD immunogenicity data demonstrated that: (1) homotypic anti-DENV4 antibodies were dominant, while the other serotypes were neutralized mostly by cross-reactive heterotypic antibodies
[23][97]; (2) individuals with higher titers of neutralizing antibodies were more protected than individuals with lower titers
[33][107]; and (3) most of the CD4+ and CD8+ T cells induced by the vaccine were directed against the yellow fever component
[33][34][35][107,108,109]. Some important conclusions can be drawn from these data: (a) the mere presence of neutralizing antibodies is not a sufficient correlate of protection for DENV, and perhaps for other flaviviruses like ZIKV, as unbalanced homotypic versus heterotypic antibody titers lead to higher titers of heterotypic, non-neutralizing, potentially enhancing antibodies; and (b) T cell mediated immunity seems to play an important role as a mechanism of protection, since this was a major missing mechanism of CYD.
Considering immunization against ZIKV, the scenario is not less complex. If the implications of DENV-induced ZIKV ADE are uncertain, there is evidence to suggest that cross-reactivity of anti-ZIKV antibodies may cause ADE against DENV, and this could be a problem in vaccination strategies (
Figure 12). It has been demonstrated that ZIKV immunity can precipitate ADE against DENV in vitro
[36][37][110,111] and in the pediatric population
[38][57]. Katzelnick et al. observed in a careful Nicaraguan pediatric cohort that previous exposure to DENV or ZIKV infection in naïve children, or one ZIKV infection in one DENV infection-experience children, increased the risk of severe disease in a subsequent DENV2 infection. Their findings also found that while high titers of anti-DENV antibodies (developed after multiple DENV infections) have protective effects, intermediate anti-DENV titers precipitate ADE in subsequent DENV orZIKV infections
[38][57]. The implication of this finding for vaccine development is clear: immunization against DENV must be addressed in the context of ZIKV immunization. DENV/ZIKV immunization can be achieved by sequential immunization, i.e., vaccination against DENV and then against ZIKV
[39][112], or preferentially by the combination of dengue and Zika vaccines.
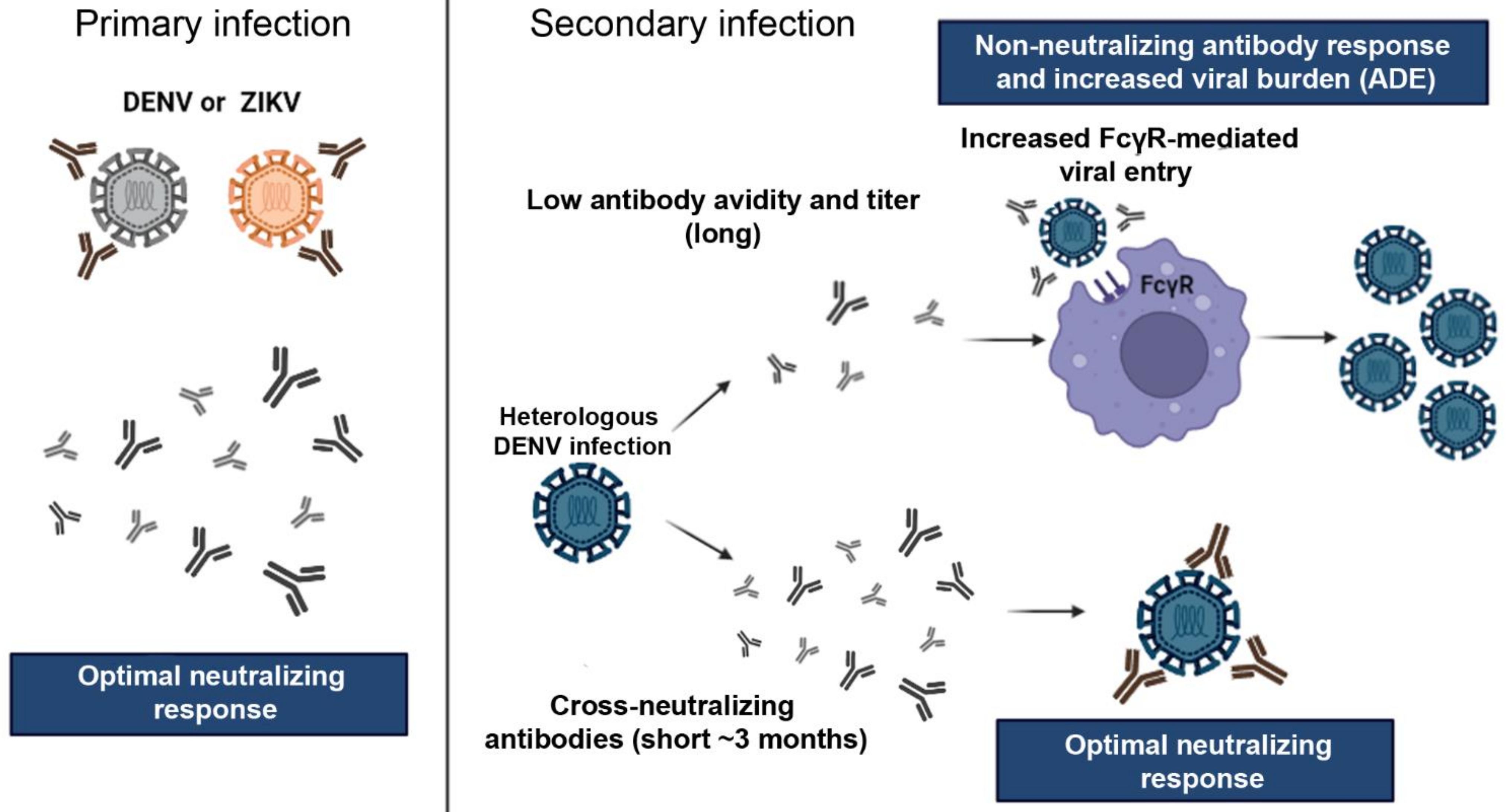
Figure 12.
Antibody response and cross-reaction effect between DENV and ZIKV. Primary infection by one of the viruses can promote the production of long-lasting neutralizing antibodies the homologous virus and short-lived cross-reactive neutralizing antibodies, i.e., in the first few months (usually 3 months) after infection, neutralizing antibodies also neutralizes heterologous related viruses. However, after 3 few months, cross-neutralization is lost and cross-reactive non-neutralizing antibodies may enhance the infection during a secondary exposure by a heterologous related virus, for example, a second distinct DENV serotype, or a DENV infection following a primary ZIKV infection. Virus particles opsonized with non-neutralizing antibodies have facilitated access to permissive cells via FcγR which causes enhanced virus proliferation and increased viral load. This phenomenon is known as antibody-dependent enhancement (ADE). On the other hand, heterologous infections after short periods between the primary and secondary infections can induce virus neutralization by cross-reactive antibodies. Abbreviations: ADE—antibody-dependent enhancement. Images from Servier Medical Art, licensed under a Creative Common Attribution 3.0 Generic License (
; accessed on 10 Februrary 2022).
A major rationale for the combination of vaccines, when possible, is the simplification of the immunization schedule
[40][113]. The number of shots necessary to cover immunization programs in developed or even Low–Middle Income Countries (LMIC) can decrease compliance and increase costs. On the other hand, the combination of vaccines has been a mechanism to reach adequate levels of immunization to as many as 14 diseases in infants below two years old. For instance, the combination of vaccines into a single shot such as pentavalent DtaP-HepB-IPV and trivalent MMR has contributed significantly to simplification of immunization schedules, increased compliance and cost reduction because of delivery efforts and vaccination campaigns are optimized to many vaccines at once
[40][113].
DENV and ZIKV often co-circulate in endemic countries because the mosquito vector is the same,
A. Aegypti or
A. albopictus [2], and the most affected populations live in developing countries where financial resources for public health are limited. There are strong epidemiological, compliance and cost-bases for the combination of DENV and ZIKV vaccines into one single shot, especially targeting the possibility of a robust immune response to such a combination due to cross-reactivity between ZIKV and DENV. If the immunization against ZIKV poses a threat for severe dengue because of cross-reactive non-neutralizing antibodies, the combination of ZIKV and DENV vaccines may provide further protection against both diseases. Again, a great lesson comes from dengue. The live attenuated dengue vaccine (LADV) TV003 induces antibodies that are mostly homotypic, with little cross-reactivity, for each of the four DENV serotypes
[41][114] and induces T cell responses to highly conserved CD8 epitopes
[42][115]. The addition of a ZIKV vaccine in the mix, or a concomitant immunization, has the potential to induce ZIKV-specific antibodies and to also add ZIKV T cell epitopes to the mix, further selecting highly conserved epitopes and improving both DENV and ZIKV immune responses.