Subjects who had experienced olfactory dysfunction or respiratory distress during COVID-19 demonstrate symptoms of balance deficits after COVID-19 recovery, and the analysis using rambling-trembling decomposition method might point at less efficient peripheral control. Monitoring for neurological sequelae of COVID-19 should be considered.
1. Introduction
Some respiratory viruses, including coronaviruses, demonstrate neurotropic capacities and ability to trigger immune response in the nervous system in vulnerable populations
[1]. The neurological manifestations are usually related to the “cytokine storm,” which results from the immune reaction to the infection of the central nervous system (CNS). Neuroinvasive properties of severe acute respiratory syndrome coronavirus (SARS-CoV) have already been demonstrated in human and animal models
[2]. SARS-CoV-2, a virus structurally similar to SARS-CoV, has recently taken over the globe, causing coronavirus disease 2019 (COVID-19) pandemic. Neurological manifestations have also been reported in the course of COVID-19 and included encephalopathy, cerebrovascular events, encephalitis, acute myelitis, and Guillain–Barre syndrome
[1]. Some authors also highlight potential persistence of coronaviruses in the nervous system
[3]. Long-term complications of SARS-CoV-2 infection are increasingly recognized and have recently become major concerns. These sequelae include not only pulmonary fibrosis but also direct or indirect damage to the neurons in the CNS or peripheral nervous system (PNS).
Clinical measurements of balance, including Berg Balance Scale, Timed Up and Go test (TUG), Tinetti test, and Functional Reach Test (FRT), are commonly used in everyday practice to assess balance, walking ability, limits of stability, and fall risk. However, these tests often fail to detect discrete balance deficits in early stages of neurological conditions
[4]. The evaluation of the static balance may be also performed in an objective, non-invasive, and quick way by monitoring the migration of center of pressure (COP) by means of force platforms
[5][6][5,6]. The main limitation of assessments based only on COP analysis is the fact that numerous components have impact on balance control, including sensory systems, dynamic control of muscles, and passive properties of musculoskeletal system and ligaments
[7]. In
thisour research,
the authors uswe used the rambling-trembling decomposition method to assess the COP trajectory in subjects who underwent SARS-CoV-2 infection. This method was developed by Zatsiorsky and Duarte, who distinguished two components making up the stabilogram
[8]. The trembling component (TREMB) reveals body oscillation around reference point trajectory, while the rambling component (RAMB) reflects the movement of a reference point with respect to which the balance of the body is promptly kept
[9]. Prior studies demonstrated that RAMB reflects predominantly the contribution of the supraspinal centers to the postural control and the processes of the CNS, while TREMB trajectory is largely dependent on peripheral components, including spinal reflexes and mechanical properties of the musculoskeletal system
[10][11][10,11]. Some authors also postulated that TREMB reflects the differences between motor planning and output
[11].
2. Detailed Analysis
2.1. The Course of COVID-19
Anosmia or partial loss of smell (hyposmia) was the most common symptom of COVID-19 and was reported by 24 (72.7%) subjects in the study group. Six (18.2%) patients suffered from shortness of breath during the infection. None of the subjects experienced severe course of the disease or were hospitalized due to COVID-19. Detailed demographic and clinical characteristics of study participants are presented in
Table 1.
Table 1.
Clinical characteristics of study participants (SD, standard deviation).
Characteristics |
Subjects Who Underwent COVID-19
| n | = 33 |
Healthy Controls
| n | = 35 |
p | -Value |
Age, years |
p < 0.001), lenRAMB (
p < 0.001), vRAMB (
p < 0.001), raTREMB (
p = 0.002), rmsTREMB (
p = 0.002), lenTREMB (
p = 0.002), and vTREMB (
p = 0.002) as well as entropy of COP (
p = 0.038) were significantly increased in the sagittal plane in subjects with respiratory problems during the course of SARS-CoV-2 infection. In addition, raTREMB and rmsTREMS were also significantly increased in the frontal plane (ML direction) for trials with closed eyes in patients who suffered from dyspnea (
p = 0.047 and
p = 0.042, respectively). Details are presented in
Table 2 and
Table 3. COP migrations in subjects who suffered from dyspnea during COVID-19 and those who did not report this symptom are demonstrated in
Figure 3.
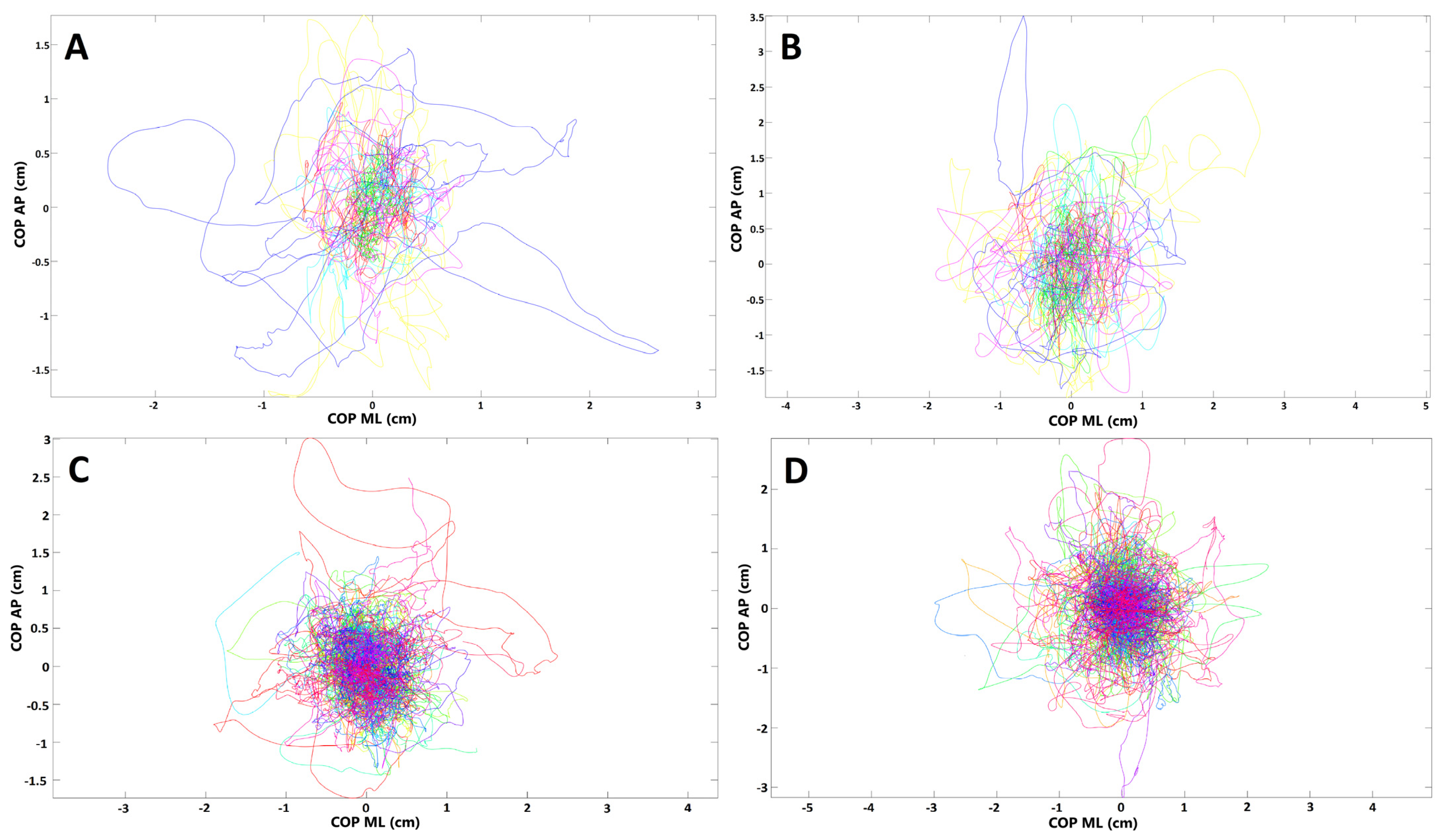
Figure 3. Presentation of COP (center of pressure) migrations in (
AB) subjects who had respiratory distress during COVID-19; (
CD) subjects without such ailments (each line represents single participant; AP, anterior–posterior (frontal) plane; ML, medial–lateral (sagittal) plane). (
AC) Trials with eyes open; (
BD) trials with eyes closed.) trials with eyes closed.
Table 2.
Comparison of the stabilographic parameters (opened eyes) in subjects who reported respiratory problems (n
= 6) in the course of COVID-19 and those who did not suffer from dyspnea during the infection (n = 27) (AP, anterio-posterior direction; ML, medio-lateral direction; COP, center of pressure; ra, range; rms, root mean square; len, length of trajectory; v, velocity; RAMB, rambling; TREMB, trembling) (* statistically significant).
|
Sagittal Plane (AP) |
|
| Frontal Plane (ML) |
|
Sagittal Plane (AP) |
|
Frontal Plane (ML) |
|
|
COVID-19 with Respiratory Problems n = 6 |
COVID-19 without Respiratory Problems n = 27 |
|
|
COVID-19 with Respiratory Problems n = 6 | |
COVID-19 without Respiratory Problems n = 27COVID-19 with Respiratory Problems |
n = 6 |
|
COVID-19 with Respiratory Problems nCOVID-19 without Respiratory Problems n = 27 |
= 6 |
COVID-19 without Respiratory Problems n |
= 27 |
|
|
Parameter |
Median (Min–Max) |
Median (Min–Max) |
p |
Effect Size |
Median (Min–Max) |
Parameter |
Median (Min–Max) |
Median
| Median (Min–Max) |
p |
Effect Size |
(Min–Max) |
---|
p |
Effect Size |
Median | (Min–Max) |
Median (Min–Max) |
p |
Effect Size |
|
|
|
Entropy COP |
0.09 (0.04–0.17) |
0.07 (0.04–0.13) |
0.234 |
0.432 |
0.09 (0.05–0.16) |
Entropy COP |
0.12 (0.05–0.17) |
0.07 (0.03–0.13) | 0.06 (0.04–0.11) |
0.098 |
0.612 |
0.038 * |
0.786 |
0.08 (0.05–0.18) |
0.07 (0.03–0.14) |
0.591 |
0.196 |
|
- -
-
Mean ± SD (range)
|
|
40.0 ± 12.8 (22–71) |
38.9 ± 14.4 (21–61) |
0.7 |
raCOP, cm |
2.15 (1.21–2.99) |
1.77 (1.05–3.29) |
0.234 |
0.432 |
1.68 (0.79–2.90) |
1.68 (0.69–3.11) |
raCOP, cm |
3.23 (2.24–4.32) |
2.35
| 2.22
| 0.981 |
(1.20–4.85) |
0.053 |
0.726 |
2.79 (0.74–4.08) |
0.3160.016 |
|
|
(1.37–4.00) |
0.363 |
- -
-
Median
|
|
39 |
rmsCOP, cm |
0.38
| 35 |
(0.23–0.62) |
0.38 | |
| (0.21–065) |
0.797 |
0.098 |
rmsCOP, cm |
0.61 (0.43–0.84) | 0.33 |
|
0.46 (0.23–0.90)(0.15–0.51) |
0.34 |
0.141 |
0.539 |
0.56 (0.23–0.72) (0.15–0.59) |
0.944 |
0.033 |
0.44 (0.15–0.80) |
0.316 |
0.363 |
Gender, | n | ( | % | ) |
|
|
|
lenCOP, cm |
27.49 (18.24–37.32) |
19.03 (15.56–35.40) |
0.042 * |
0.766 |
19.98 (15.67–29.14) |
17.35 (8.94–29.9) |
0.216 |
0.450 |
lenCOP, cm |
51.69 (29.41–62.82) |
26.75 (19.53–50.30) |
0.001 * |
1.383 |
27.67
|
|
- -
-
Male
|
|
6 |
9 |
0.45 |
|
|
vCOP, cm/s |
0.93 (0.62–1.26) |
0.64 (0.53–1.20) |
0.042 * |
0.766 |
0.68 (0.53–0.99) |
0.59 (0.30–1.02) |
0.216 |
0.450 |
- -
-
Female
|
|
27 |
26 |
|
(20.11–62.02) |
24.42 |
| (10.79–45.93) |
0.072 |
0.668 |
vCOP, cm/s |
1.75 (1.00–2.13) |
0.91 (0.66–1.70) |
0.001 * |
1.383 |
0.94 (0.68–2.10) |
0.83 (0.37–1.56) |
0.072 |
0.668 |
raRAMB, cm |
1.99 (1.15–2.71) |
raRAMB, cm |
2.79 1.69 (1.06–3.11) |
0.363 |
(2.12–3.79)0.329 |
1.54 |
2.22 (1.14–5.25) |
0.118 (0.79–2.49) |
1.61 (0.67–2.97) |
0.981 |
0.575 |
2.43 (1.27–3.45) |
2.04 (0.71–3.95) |
0.4690.016 |
0.262 |
Height, cm |
|
|
|
rmsRAMB, cm |
0.37 (0.22–0.57) |
0.37 (0.21–0.64) |
0.981 |
0.016 |
0.31 |
rmsRAMB, cm |
0.57
|
| (0.15–0.47) |
0.33 (0.14–0.56) |
0.907 |
0.049 |
(0.39–0.78) |
0.45 (0.21–0.90) |
0.155 |
0.521 |
0.53 (0.20–0.67) |
0.41 (0.14–0.76) |
0.363 |
0.329 |
|
- -
-
Mean ± SD (range)
|
|
167.1 ± 6.8 (152–180) |
167.4 ± 8.6 (155–190) |
0.75 |
lenRAMB, cm |
23.43 (17.02–31.21) |
17.70 (14.60–30.65) |
0.038 * |
0.786 |
17.47 (14.81–26.13) |
16.46 (8.16–26.18) |
0.253 |
0.415 |
|
- -
-
Median
|
|
168 |
165 |
lenRAMB, cm |
41.70 (25.07–45.69) |
24.42 (17.82–38.70) |
0.001 * |
1.354 |
23.47 (18.24–49.60) |
21.86 (9.69–37.99) |
0.129 |
0.557 |
vRAMB, cm/s |
0.79 (0.58–1.06) |
0.60 |
vRAMB, cm/s |
| |
(0.49–1.04) |
0.038 * |
1.410.786 |
(0.85–1.55) |
0.83 (0.60–1.31)0.59 (0.50–0.89) |
0.56 |
0.001 * |
1.354 |
0.80 (0.62–1.68) (0.28–0.89) |
0.253 |
0.415 |
0.74 (0.33–1.29) |
0.129 |
Weight, kg |
|
|
|
0.557 |
raTREMB, cm |
0.59 (0.21–1.21) |
0.26 (0.12–0.87) |
0.030 * |
0.826 |
0.47 (0.18–0.56) |
0.28
|
raTREMB, cm | (0.08–0.66) |
0.169 |
0.503 |
1.25 0.77–1.74) |
0.47 (0.20–2.31) |
0.002 * |
1.271 |
0.76 (0.32–1.30) |
0.43 (0.09–1.69) |
0.047 * |
0.746 |
|
- -
-
Mean ± SD (range)
|
|
68.6 ± 16.4 (45–121) |
68.4 ± 16.3 (46–110) |
0.99 |
rmsTREMB, cm |
0.05 (0.01–0.11) |
0.02 (0.01–0.08) |
0.038 * |
0.786 |
0.04 (0.01 -0.05) |
rmsTREMB, cm |
0.11 (0.06–0.20) |
0.04
| 0.02 (0.00–0.06) |
0.129 |
(0.01–0.21) |
0.002 * |
1.2710.557 |
0.07 (0.02–0.14) |
0.03 (0.00–0.18) |
0.042 * |
0.766 |
|
- -
-
Median
|
|
62 |
65 |
|
lenTREMB, cm |
9.77 (4.33–16.17) |
4.86 (2.57–14.52) |
0.042 * |
0.766 |
5.39 (2.89–7.85) |
3.75 (1.46–9.34) |
0.141 |
0.539 |
lenTREMB, cm |
23.53 (10.05–35.43) |
7.92 (3.75–30.06) |
0.002 * |
1.298 |
9.28 (5.51–27.90) |
6.52 (1.77–24.01) |
0.053 |
0.726 |
Symptoms of COVID-19, | n | (%) |
|
- |
- |
vTREMB, cm/s |
0.33 (0.15–0.55) |
0.16 (0.09–0.49) |
0.042 * |
0.766 |
vTREMB, cm/s |
0.80 (0.34–1.20) | 0.18 |
|
0.27 (0.13–1.02)(0.10–0.27) |
0.13 |
0.002 * |
1.298 |
0.31 (0.19–0.95) (0.05–0.32) |
0.141 |
0.539 |
0.22 (0.06–0.81) |
0.053 |
0.726 |
|
- -
-
Malaise
|
|
26 (78.8) |
|
- -
-
Anosmia/hyposmia
|
|
24 (72.7) |
|
- -
-
Arthralgia/myalgia
|
|
21 (63.6) |
|
- -
-
Headache
|
|
21 (63.6) |
|
- -
-
Fever
|
|
16 (48.5) |
|
- -
-
Cough
|
|
16 (48.5) |
|
- -
-
Rhinitis
|
|
13 (39.4) |
|
- -
-
Sore throat
|
|
11 (33.3) |
|
- -
-
Chest pain
|
|
10 (30.3) |
|
- -
-
Chills
|
|
10 (30.3) |
|
- -
-
Diarrhea
|
|
8 (24.2) |
|
- -
-
Dyspnea/shortness of breath
|
|
6 (18.2) |
|
- -
-
Nausea/vomiting
|
|
6 (18.2) |
|
- -
-
Conjunctivitis
|
|
1 (3.0) |
|
- -
-
Skin lesions
|
|
0 (0) |
2.2. Postural Control in Subjects Who Underwent COVID-19 versus Healthy Controls
The spatiotemporal parameters of the COP (raCOP, rmsCOP, lenCOP, vCOP, entropy); RAMB (raRAMB, rmsRAMB, lenRAMB, vRAMB); and TREMB (raTREMB, rmsTREMB, lenTREMB, vTREMB) in the sagittal (AP) and frontal (ML) plane, for the trials performed with opened and closed eyes, were compared between the study and control group. No statistically significant differences in any of the stabilographic parameters were found between subjects who underwent COVID-19 and healthy controls (
p > 0.05). COP migrations in subjects who recovered from COVID-19 and healthy controls are presented in
Figure 1.
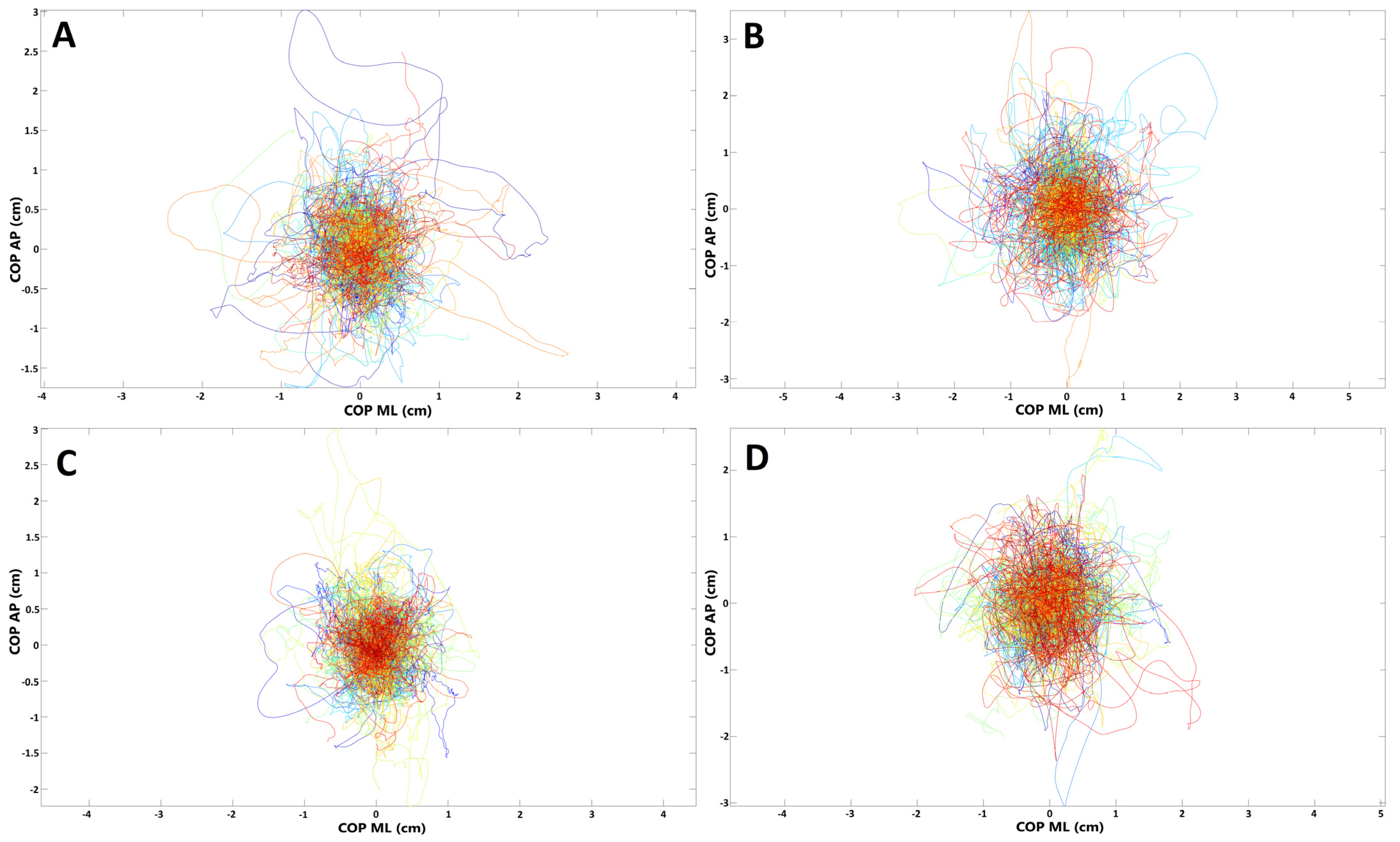
Figure 1. Presentation of COP (center of pressure) migrations in (
AB) subjects who recovered from COVID-19; (
CD) healthy controls (each line represents single participant; AP, anterior–posterior (frontal) plane; ML, medial–lateral (sagittal) plane). (
AC) Trials with eyes open; (
BD) trials with eyes closed.) trials with eyes closed.
For further analysis, the study group was divided into subgroups depending on the presence of selected symptoms of COVID-19.
2.3. Postural Control in Subjects with Olfactory Abnormalities
To evaluate if there are any balance disturbances in patients who had olfactory abnormalities during the SARS-CoV-2 infection,
thwe
authors compared the stabilographic parameters between subjects who reported anosmia/hyposmia during COVID-19 (
n = 24) and those who did not (
n = 9) have olfactory abnormalities. There were no statistically significant differences in sagittal and frontal plane for the measurements with opened eyes (
p > 0.05). However, raTREMB and rmsTREMB for sagittal plane were significantly increased in subjects with olfactory abnormalities when their eyes were closed (
p = 0.03 and
p = 0.04, respectively). COP migrations in subjects who suffered from olfactory abnormalities during COVID-19 and those who did not report such ailments are presented in
Figure 2.
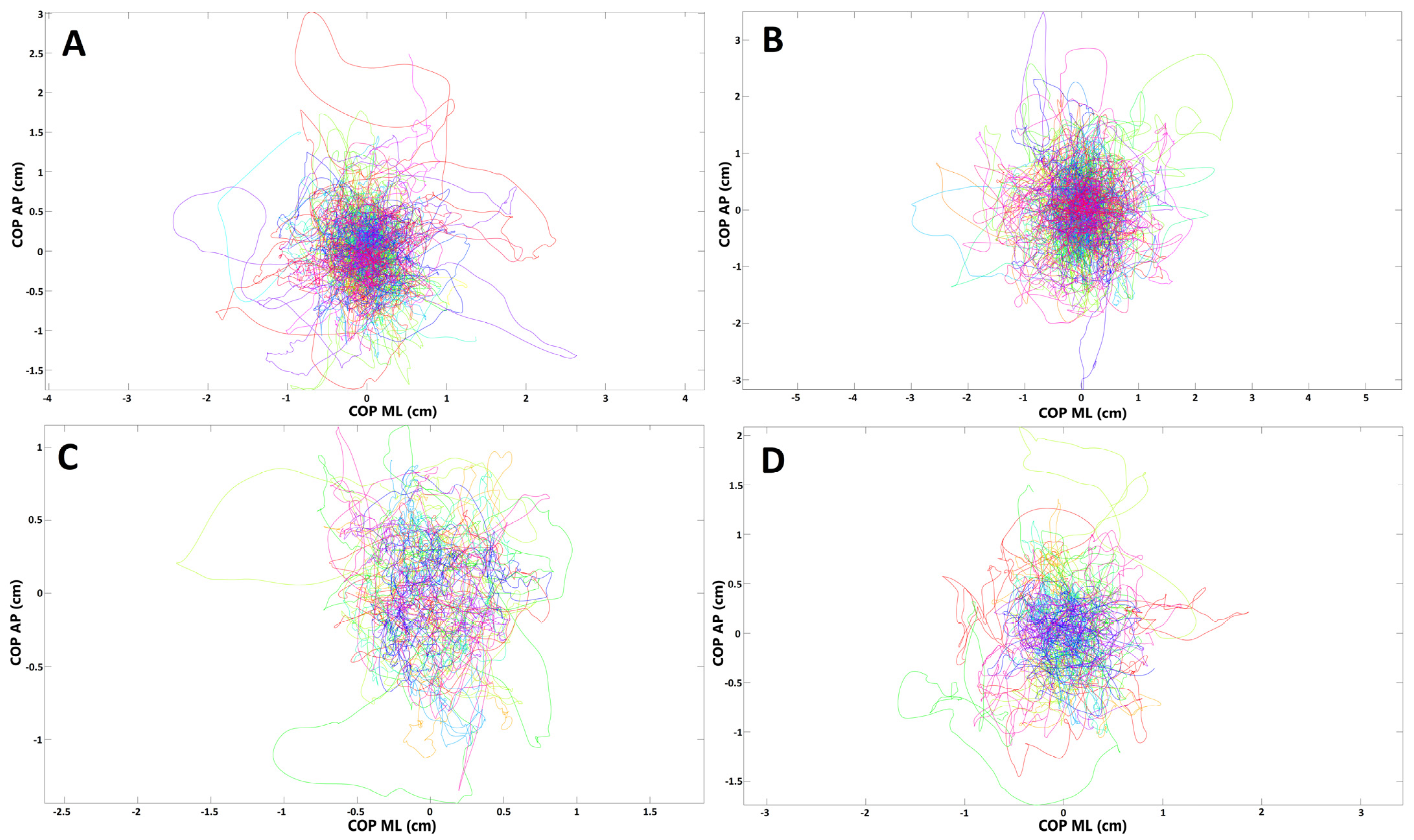
Figure 2. Presentation of COP (center of pressure) migrations in (
AB) subjects who had olfactory abnormalities during COVID-19; (
CD) subjects without such ailments (each line represents single participant; AP, anterior–posterior (frontal) plane; ML, medial–lateral (sagittal) plane). (
AC) Trials with eyes open; (
BD) trials with eyes closed.) trials with eyes closed.
2.4. Postural Control in Subjects with Dyspnoea
In the next step,
thwe
authors compared the stabilographic parameters in convalescents with positive history of dyspnea and those who did not report such symptom during the course of SARS-CoV-2 infection. Several statistically significant differences were noted in the stabilographic parameters between the two groups. The differences were observed for assessments with opened and closed eyes but were almost exclusively present in the sagittal plane. For measurements with opened eyes, lenCOP (
p = 0.042), vCOP (
p = 0.042), lenRAMB (
p = 0.038), vRAMB (
p = 0.038), raTREMB (
p = 0.03), rmsTREMB (
p = 0.02), lenTREMB (
p = 0.042), and vTREMB (
p = 0.042) were significantly increased in the sagittal plane in subjects with dyspnea. For trials with closed eyes, lenCOP (
p < 0.001), vCOP (
= 27) (AP, anterio-posterior direction; ML, medio-lateral direction; COP, center of pressure; ra, range; rms, root mean square; len, length of trajectory; v, velocity; RAMB, rambling; TREMB, trembling) (* statistically significant).
Table 3.
Comparison of the stabilographic parameters (closed eyes) in subjects who reported respiratory problems (n
= 6) in the course of COVID-19 and those who did not suffer from dyspnea during the infection (n = 27) (AP, anterio-posterior direction; ML, medio-lateral direction; COP, center of pressure; ra, range; rms, root mean square; len, length of trajectory; v, velocity; RAMB, rambling; TREMB, trembling) (* statistically significant).
= 27) (AP, anterio-posterior direction; ML, medio-lateral direction; COP, center of pressure; ra, range; rms, root mean square; len, length of trajectory; v, velocity; RAMB, rambling; TREMB, trembling) (* statistically significant).
2.5. Stabilographic Measurements and Other Symptoms of COVID-19
Further subgroup analysis considering various clinical symptoms of SARS-CoV-2 infection (
Table 1) did not reveal any further significant differences regarding the stabilographic parameters between convalescents with and without particular ailments (data not shown).
3. Current Insights
Post-COVID-19, also referred to as chronic COVID-19, is increasingly recognized in patients who were initially believed to have completely recovered from the infection. It may result in gradual loss of lung function and long-term damage of the CNS and PNS
[12][13][13,14]. One of the major concerns is also the putative increased risk of development of neurodegenerative conditions, including Alzheimer’s disease, Parkinson’s disease, and multiple sclerosis, following the SARS-CoV-2 infection.
Respiratory viruses may enter the nervous system through hematogenous route, e.g., by infecting the cells of the circulatory system and crossing the blood-brain barrier (BBB), or through neuronal retrograde routes by infecting directly nerve endings and using the axonal transport to reach the CNS
[14][15]. Several recent findings favor the neurotropic and neuroinvasive capacities of SARS-CoV-2. The receptor that binds to S protein, ACE2, is highly expressed in the endothelial cells of the brain microvasculature
[15][16][16,17]. Immune cells, e.g., macrophages, also express ACE2 and may carry SARS-CoV-2 inside their cytoplasm across the BBB
[17][18]. In addition, high expression of ACE2 in various sites of the brain constitutes potential target for SARS-CoV-2 and may not only facilitate acute brain damage, but it may also provide a basis for long-term neurodegeneration
[18][19].
The glial cells constitute the first line of defense once the virus enters the CNS, and the activated microglia are considered a marker of neuropathology and neuroinflammation
[19][20]. The virus itself may trigger neurodegeneration either directly using its cytolytic effects or indirectly through secondary immune responses
[20][21]. Some authors postulate that the alterations in the expression of proteins responsible for axonal transport and synaptic transmission that occur during the viral infection are similar to the changes observed in early neurodegenerative conditions
[21][22][23][22,23,24]. SARS-CoV-2 infection may accelerate processes such as neuroinflammation, synaptic pruning, and neuronal loss, which also constitute the structural basis of Alzheimer’s disease
[24][25]. Moreover, SARS-CoV-2 has recently been shown to cause demyelination of the brain and spinal cord and has been linked to signs similar to those of multiple sclerosis
[25][26][26,27].
Some respiratory viruses, including SARS-CoV-1 and MERS-CoV, may reach the brain using the olfactory nerve
[15][27][16,28]. Anosmia or partial loss of smell are commonly reported in association with SARS-CoV-2 infection. Olfactory dysfunction in patients with COVID-19 is suggested to result from direct damage of the receptor neurons in the olfactory epithelium. One of the potential theories is that SARS-CoV-2 in the initial phase enters high-ACE2-expressing nonneuronal cells in the olfactory epithelium and subsequently passes to low-ACE2-expressing neurons, from where it is anterogradely transported along axons to the brain
[1]. In addition, sustentacular cells and stem cells in the olfactory epithelium widely express transmembrane serine protease 2 (TMPRSS2), another binding protein for SARS-CoV-2
[16][17].
Some authors also hypothesize that SARS-CoV-2 may enter the CNS through the vagus nerve and subsequently invade the respiratory control center located in close proximity to the vagal nucleus in the brainstem. As a result, the respiratory distress related to the infection in the lungs may be aggravated when the virus reaches the brainstem
[1][28][1,29].