1. Introduction
Cucumber (
Cucumis sativus L.) is an important and popular vegetable of the Cucurbitaceae family. It is reported to be one of the earliest vegetables to be domesticated from wild Indian
C. s var.
Hardwickii [1] and its site of domestication by man could be dated back as far as 5000 years ago
[2][3][2,3]. Cucumber was first cultivated in India and then later spread eastwards to China and westwards to Europe about 2000 and between 700 and 1500 years ago, respectively. The Europeans eventually spread cucumber to North America and Africa in the 1400s
[3][4][3,4]. Cucumber is mostly grown for its soft fruits and is eaten in raw fresh form, cooked as a vegetable or pickled when immature
[5][6][5,6]. Consumed fresh or processed, cucumber is regarded for its special flavor and its high-water content (95% water). Its high-water content (about 95%) makes it ideal to promote hydration during hot sunny days. The nutrient availability per 100 g fresh weight of cucumber contains energy (12–15 cal), protein (0.6–0.65 g), total lipids (0.1–0.11 g), carbohydrates (2.2–3.63 g), calcium (14–16 mg), iron (0.28 mg–0.3), vitamin C (2.8–12 mg), and vitamin A (β-carotene, 1.125–5 μg), as well as other vitamins such as vitamin K, B6, B12, thiamine, niacin, flavonoids, and triterpenes, which provide antioxidants and anti-inflammatory substances
[7][8][9][7,8,9].
The semi-wild Xishuangbanna cultivar (
C. sativus var. xishuangbannesis, XIS hereafter) is unique based on their pedigree exhibiting orange-fleshed (endocarp) fruit at full seed maturity, signifying an abundance of β-carotenes just like melon, squash, and pumpkin
[10][11][10,11]. The XIS cucumber is highly outcrossing, and thus is readily intercrossed to the common cultivated cucumber, sharing the same chromosome number (2n = 2x = 14). Its fruits are eaten boiled or raw during different stages of maturity
[12][13][12,13]. β-carotene not only acts as a precursor to pro-vitamin A synthesis, which is essential for human health, but also contributes to the fruit flesh color. Flesh color is one of the most important traits of fruits and vegetables that impacts positively on consumer’s choice of food products. The flesh color type is attributed to different chlorophyll and carotenoid accumulations in fruits
[14]. Low uptake of carotenoid-rich food results in vitamin A deficiency. Vitamin A deficiency is one of the most serious health issues in many countries, resulting in irreversible blindness. It is among the key pillars in the fight against malnutrition, commonly referred to as ‘hidden hunger”, ravaging the ever-increasing world population
[15]. This situation has prompted researchers to attempt the biofortification of plant-derived foods including pro-vitamin A carotenoids (mainly β-carotene), which led to the birth of “golden” crops. Additionally, a variety of vegetables such as carrot and orange-fleshed sweet potato are naturally rich in β-carotene content and their improvement contributes to the pool of nutritional status. While high β-carotene cucumber may not contribute significantly to the vitamin A status among the consumers globally owing to its limited growing localities, consumption of carotenoid-rich XIS cucumber in regions where it is grown and marketed can contribute to the reduction in vitamin A deficiency and provision of health benefits required in the human body system
[16].
The objective of this review is to provide research progress and the current status of the novel orange-fleshed cucumber and pinpoint bottlenecks undermining diversifying selection for genetic improvement. We also highlighted possible research undertakings for the traits [16]of unknown regulatory mechanisms. Through the findings of the previous studies, involving XIS cucumber and the XIS derived lines, this review thus provides insights into orange-fleshed cucumbers and future research potentials. XIS cucumber, which originated in Yunnan province in China, was first brought to the attention of horticulturists in 1983 and has since been under investigations. Quantitative trait loci conferring fruit quality characteristics including β-carotene content, fruit size, fruit number, and fruit weight have been molecularly tagged. Based on the findings, XIS cucumber provides novel alleles that can be used for cucumber genetic improvement. We hope that this review coupled with the highlighted key research directions proposed herein will shape the genetic research improvement program of orange-fleshed cucumber.
2. Chronological Perspective of Orange-Fleshed XIS Cucumber Discovery and Development
XIS is a Chinese landrace, an accession from mountainous Xishuangbanna region of Yunnan Province in Southwest China, the area bordering Myanmar
[12]. XIS cucumber was first described in 1983
[17]; thereafter, it drew the attention of the researchers, especially those interested in cucurbitaceous crops owing to its unique characteristics. Growing in a tropical warm and humid climate above 1000 m altitude, this form of cucumber has long been cultivated by the Jinuo, Hani, and Aini ethnic groups of China, Laos, and Vietnam, who call it ‘Shihuo’
[10].
A preliminary investigation of the orange flesh trait of XIS cucumber in 1991showed that a dominant genetic factor influences the orange-flesh color
[18]. However, three years later (1994) Navazio
[19] used a recessive two gene model to explain segregation in F
2 progeny (
n = 46) resulting from a cross between ‘SMR18’ and XIS. In 1997, Simon and Navazio
[16] developed XIS derivatives in U.S. pickling backgrounds that express orange fruit flesh pigmentation at maturity. This consisted of the development of early orange mass (EOM) 400, 402, and late orange mass (LOM) 404 from crosses between U.S. pickling cucumber and orange fruited XIS cucumber. Internal (mature and immature) fruit color was orange in 70–90 percent of the EOM 400 and EOM 402 plants in 1994, with yellow in the remaining samples. Plants with dark orange mature fruit color were slightly more prevalent in EOM 402 (15%) than in EOM 400 (5%); however, yellow immature fruit color was also slightly more common in EOM 402 (30%) than in EOM 400 (5%). However, the molecular mechanisms linked to early β-carotene accumulation of orange mass in EOM 402 or EOM400 remains unknown.
In 2009, a genetic study involving two genetic linkage maps constructed with the markers of random amplified polymorphic DNA (RAPD), sequenced characterized amplified region (SCAR), simple sequence repeats (SSR), Expressed sequence tag (EST), single nucleotide polymorphism (SNP), amplified fragment length polymorphism (AFLP), and (sequence specific amplification polymorphism (SSAP) was reported
[20].These genetic linkage maps defined a common collinear region containing four molecular markers (3 dominant and 1 codominant) on linkage group (LG) LG6 in Map 1 and LG3 in Map 2. The regions contained QTL associated with orange mesocarp (mc6.1/ec6.1) and endocarp (mc3.1/ec3.1) in Map1 and Map2, respectively. Two pairs of allelic genes were found to be responsible for the yellow mesocarp, while a single recessive gene controls β-carotene in the endocarp as reported in 2010
[21] However, there are limited findings on cucumber flesh color at the molecular level. Through the United States department of Agriculture, a high β-carotene (7.5 μg/g) cucumber line EOM 402-10, was released in 2011 but it was still maintained for research purposes only
[22].
In 2012, an inheritance and mapping of the gene controlling quantity of β-carotene at mature fruit stage was reported
[23]. To identify the genetic locus controlling β-carotene content in XIS cucumber, bulk segregant analysis (of 124 RIL-F
7 population was applied and orange endocarp (Ore) gene controlling quantity of β-carotene identified to be located between SSR07706 and SSR23231 in the short arm of chromosome 3. Further investigation in 2013 revealed that the Ore locus is defined by the putative β-carotene hydroxylase 1; CsaBCH1 (Csa3G183920) gene. The Ore mutant contains a SNP in the conserved fatty acid hydroxylase domain of CsaBCH1 that disrupts β-carotene turnover, which is a substitution of alanine to asparagine at position 257 of the
BCH1 gene, encoding a β-carotene hydroxylase, and causes an accumulation of β-carotene in the Xishuangbanna group. This mutation results in a non-functional carotene hydroxylase, which is unable to convert carotene to zeaxanthin, resulting in a build-up of carotene
[1][24][1,24].
Many other attempts have been made to study the regulations of β-carotene in orange-fleshed cucumber cultivars. Other variants of cucumber with yellow flesh significantly contribute to β-carotene content. In 2015, an investigation of the yellow flesh color of cucumbers (line PI200815) discovered that the yellow mesocarp flesh color is controlled by a single recessive gene, yf, which is located on cucumber chromosome 7
[25]. A total of 12 SSR primers and 5 Indel markers were used to build a molecular marker linkage group and the closest flanking markers linked to yf were yf SSR108 and yf Indel29 with genetic distances of 0.6 and 0.3 cM, respectively. The physical distance between
yf and the area containing 21 projected candidate genes was 149.0 kb. The latest study of XIS cucumber in 2019 on β-carotene content reported an identification of SNP in the homolog of the cauliflower Ore gene. This study however concluded that the Ore gene is not the only factor affecting β-carotene accumulation in orange flesh cucumber
[26]. Other than β-carotene content, the QTL of the flowering and fruit physical qualitative traits have recently have also been reported
[27][28][27,28]. A study involving a combination of genomics and transcriptomic further gave insights into photoperiod regulation of flowering in XIS cucumber
[29].
4. Future Prospects in Molecular Breeding of β-Carotene Content in Cucumber
Based on the foregoing review, it is apparent that previous research on β-carotene accumulation in orange-fleshed cucumber has been explored through introgression of XIS with other cultivated cucumber species for the identification of QTL and related markers. However, more approaches for further insights into XIS cucumber could be tested, as summarized in this section (
Figure 2). Rajasree and Pugalendhi
[54][55] highlighted the modern nutritional breeding techniques with wider application in vegetable crops, and these include the following: mutation breeding
[55][56], polyploidy breeding
[56][57], biotechnological approaches, advanced backcross QTL analysis, and transgenic approaches
[57][58]. Each approach has distinct or shared objectives in XIS cucumber. Given the relatively few QTL associated with XIS traits and their comparatively high allied LOD values, backcross MAS could be advantageous in the development of orange-colored, high β-carotene containing cucumber germplasm and other associated traits
[20].
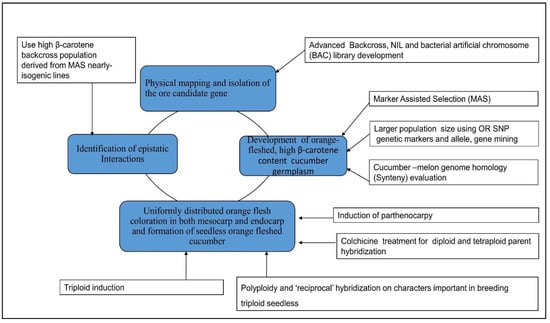
Figure 2. A summary of the proposed approaches to unravel the genetic basis of β-carotene accumulation in orange-/yellow-fleshed cucumber. The boxes highlighted in blue are the proposed main objectives, while the arrow points to the applicable methodology. Advanced backcross
[21]; MAS
[21][22][25][21,22,25]; OR SNP genetic markers
[26]; Triploid induction
[56][57]; cucumber-melon synteny
[58][59][59,60]; parthenocarpy
[60][61]; colchicine treatment
[61][62]; polyploidy hy-bridization
[62][63].
4.1. Combined Omics and Gene Editing Technology for Carotenoid Improvement
Combined ‘omics’ presents a powerful tool for molecular diagnosis of important qualitative and quantitative traits. Whereas the cucumber genome has been sequenced and released for the emergence of next generation sequencing technologies
[63][64], genomics alone may not meet the demand of the researchers. With the rapid development of sequencing technology, many omics technologies, such as transcriptomics, proteomics, and metabolomics, have been developed
[64][65] and applied in cucumber research. A large amount of data generated from these studies provides a potential reference for mining cucumber functional genes controlling carotenoid accumulation. The combination of genomics and classical genetics has become a highly strong technique for identifying candidate genes influencing essential features such as carotenoid accumulation.
Combined ‘omics’ involving transcriptomics, proteomics, and metabolomics has been used to further clarify the complex signal conversion mechanism of key regulatory genes in carotenoid accumulations
[65][66]. Combining metabolite profiling with high-throughput RNA-sequencing analysis as well as proteomics established a correlation network between metabolites and genes involved in a number of important traits such as fruit flavor, fruit pigments, and other sensory characteristics in a number of crops. However, such studies are still lacking in dissecting the molecular mechanism of carotenoid biosynthesis in XIS cucumber. A number of regulators may be involved in those processes. Future studies should address the identification of key regulatory factors controlling the carotenoid metabolic pathway and chromoplast biogenesis. Genome editing research is currently being employed for plant research and crop improvement program
[66][67]. By analyzing the differences between mutant and control plants, CRISPR/CAS9-mediated deletion and siRNA-mediated knockdown technologies can be utilized to identify the function of candidate genes
[67][68]. Targeting of regulatory regions has been shown to alter gene expression without complete knockout of genes, leading to the development of useful traits. This is a current trend application for nutritional molecular breeding. For example, a comparison of the effects of mutations in the coding region to that of the 5′ untranslated region of CRTISO gene in tomato demonstrated that fruit quality changes can be achieved by CRISPR-Cas9-based editing of CRTISO coding region
[68][69] Further, a controlled knockdown of genes can be achieved by targeting the regulatory regions. In a similar study it was reported that color-related chlorophyll and carotenoid concentrations of Chinese kale can be altered through CRISPR/Cas9-targeted editing of the carotenoid isomerase gene, BoaCRTISO
[69][70]. More specific and accurate modifications could be created at specific regulatory elements that are part of the untranslated sections or promoter regions of genes using modern technologies like “prime-editing”, resulting in desired phenotypic features. These precise approaches could be used in cucumber improvement initiatives to increase β-carotene content.
4.2. Candidate Gene Approach and Association Mapping Applications in Carotenoid Nutritional Improvement
The candidate gene approach can systematically examine the relationship between structural genes in carotenoid biosynthetic pathway genes (PSY, PDS, ZDS, LCYB/LCYE, CHYB, VDE, ZEP, and NSY) and related phenotypic variability by using a comparative genetic system
[70][71][71,72]. Comparative genetic maps, non-overlapping sets of mutants generated across plant species, could be more informative about the function and evolution of that locus than the allelic variability available in one species, which can be used to extend the candidate gene method to these features
[72][73]. Furthermore, candidate genes for phenotypically defined quantitative and qualitative loci may be readily apparent for a wide range of species, providing new insights into cucumber β-carotene accumulation.
Association mapping targeting candidate genes has proven successful in many instances
[73][74]. Therefore, this approach might bring new insights into carotenoid biosynthesis as the genetic pathway has already been dissected through forward and reverse genetics in many organisms. It addresses the relationship between marker-based polymorphism and phenotypic variation in a diversified population. In a diverse population, it examines the link between marker-based polymorphism and phenotypic variation. Using a diverse population and all ancestral recombination events may improve the resolution of such a study. In comparison with bi-parental cross, one of the key advantages of association mapping population is the ability to examine a large number of alleles
[73][74].
4.3. Manipulation of Hormonal and Environmental Cues Involved in the Regulation of Carotenoid Biosynthesis
Molecular diagnosis and manipulation of hormonal and environmental factors might bring new insights into the mechanisms of carotenoid biosynthesis. Hormonal and environmental factors have been shown to influence carotenoid accumulation either directly on carotenoid synthesis genes or indirectly by acting on transcription factors involved in carotenoid biosynthesis
[74][75][75,76]. Light and temperature are the key environmental factors regulating carotenoid biosynthesis
[76][77]. The effects of plant hormones on carotenoid accumulation are well studied in tomato fruits. In addition, ethylene is an important hormone that plays a key role in regulating ripening-associated processes including carotenoid accumulation. Ripening inhibitor a master regulator of ethylene regulated fruit ripening, enhances lycopene accumulation during fruit ripening by positively regulating expression of PSY, PDS, ZDS, and CRTISO, and negatively regulating expression of LCYB and LCYE
[77][78]. Therefore, studies elucidating the impact of these environmental factors on carotenoid accumulation in cucumber, particularly XIS cucumber, need future investigations.
Structurally, fruits of XIS cucumber develop hollowness at the center of the endocarp, and this further limits the quantity of β-carotene derived from it by consumers. Moreover, it is the mesocarp that constitutes the greater percentage of the edible portion. Thus, developing a uniformly distributed orange-fleshed (mesocarp and endocarp) cucumber variety would be a greater milestone in carotenoid improvement. Such a novel cucumber variety would translate into the formation of a seedless cucumber similar to seedless watermelon. The known techniques of forming a seedless fruit comprise establishment of a parthenocarpic fruit or triploid development, as proposed herein, and hormonal or chemical treatments such auxins, ethylene, gibberellins or colchicine, among others
[60][61][62][78][61,62,63,79].
4.4. Application of Marker-Assisted Selection for β-Carotene Improvement and Development of Immature Orange-Fleshed Cucumber Variety
Previously, the diallel analysis of immature fruit sizes demonstrated significant positive genetic combining ability for P101 (derived from XIS ‘Addis’). Because orange color in immature cucumbers has not previously been detected in the most generally consumed stage of cucumbers, P101’s capacity to express fruit pigmentation in size 2 (27–38 mm) fruit was a fortuitous and unexpected breakthrough in the breeding for carotenoid pigmentation
[11]. The diallel study revealed that the parents had a high fruit carotene level. However, most diallel hybrids had a lower carotenoid content than their parents, indicating both low carotenoid content and a lack of genetic complementation among parents to improve fruit color. The genetic regulation of pigmentation in fruit with a diameter of 27–38 mm appeared to be separate from that of fruits with a diameter of 51–54 mm. Although the carotene concentration in immature fruit was not quantified, the accumulation of β-carotene at this stage is regulated by many genes that have additive effects
[11]. This allows for increased carotene content (orange color) through family selection. EOM 402-10 could be used to generate high-carotene pickled cucumbers with an orange endocarp/mesocarp that are appealing to customers thanks to their nutritional content. However, until now, no studies have further explored the genetic mechanism of orange coloration in immature EOM402-10 cucumber.
Genomics and marker assisted selection (MAS) technology has unlocked new pathways for improvement of complex quality traits
[59][79][60,80]. Therefore, fully defined high-carotene BC
2S
3 IBL produced by MAS and phenotypic selection could be used directly in epistatic interaction detection
[62][63]. Additionally, the identification of XIS progeny line segregating for both ore and fft1.1 loci (‘OreFt’), being β-carotene donor, can be used to foster β-carotene breeding initiatives. The β-carotene donor can then be instituted in the MAS by back crossing with inbred line segregating for fft1.1 locus (FFT), as outlined in
Figure 3. One NIL study might look into the differences in fruit color orange hue and its dispersion observed in fruit developing in the field and greenhouse under long-day and short-day growth settings
[10].
Figure 3. Marker assisted selection (MAS) strategy for utilization of ore recessive genes for development of an early flowering cucumber with enhanced β-carotene content. The phenotype description of above genes is based on the previous studies. OreFt, orange endocarp and early female flowering; fft, first female flowering time; P1, parent 1; P2, parent 2, Selfing, ⨂.
5. Conclusions
Xishuangbanna cucumber provides a useful genetic resource for cucumber genetic improvement. XIS cucumber is predominantly recognized because of its high β-carotene content. β-carotene accumulation and regulation in fruits are affected by QTL, environmental factors, hormones, and transcription factor, among others. Beyond the nutritional benefits of β-carotene content, the striking orange flesh appearance of this commodity could attract consumer attention as a specialty crop either in processed or fresh state. Plant nutritional quality trait improvement has become a global necessity owing to the ever-increasing world overpopulation and the demand for quality food One of the main goals of modern breeding projects is to produce agricultural species with improved nutrients and health-promoting properties, thus XIS cucumber could contribute in nutritional improvement programs. About half a century since the orange-fleshed cucumber was brought to the attention of horticulturists, many traits of agronomic importance other than β-carotene have been investigated. The quantitative trait loci related to flowering time, both female and male flowers, photoperiod response to flowering, and fruit quality characteristics (fruit size, weight, and shape) have been reported. However, fine mapping of the candidate genes regulating these traits remains a potential research area. Nevertheless, the identified QTL provide important clues for future fine mapping and cloning of the candidate genes. A significant molecular and germplasm improvement program can be used for rapid progress in cucumber nutritional breeding, including multiple germplasm and genetic collections, genetic engineering, marker assisted selection, and gene editing. We expect this review will appeal to the cucurbitaceous crop researchers and provide a reference for basic and applied research into XIS cucumber in the future.
Author Contributions
Conceptualization, J.C., J.O.O. and H.O.O.; methodology, H.O.O.; investigation, H.O.O., C.C. and Z.T.; resources, Q.L; writing—original draft preparation, H.O.O.; writing—review and editing, C.C., Z.T., J.L. and X.Y.; supervision, J.C., Q.L. and J.O.O.; project administration, Y.W.; funding acquisition, J.C. All authors have read and agreed to the published version of the manuscript.
Funding
This research was funded by National Key Research and Development Program of China (2020YFE0202900,2021YFD1200201), Asia Cooperation Fund Project-2021, the National Natural Science Foundation of China (31902007, 31902006), Jiangsu Belt and Road innovation cooperation project (BZ2019012), Jiangsu Agricultural Innovation of New Cultivars (PZCZ201719), and A Project Funded by the Priority Academic Program Development of Jiangsu Higher Education Institutions.
Institutional Review Board Statement
Not applicable.
Informed Consent Statement
Not applicable.
Data Availability Statement
Not applicable.
Acknowledgments
We are grateful for the technical support provided by the staff and members in the laboratory of cucurbit genetics and germplasm enhancement, College of Horticulture, Nanjing Agricultural University, China.
Conflicts of Interest
The authors declare no conflict of interest and The funders had no role in the design of the study; in the collection, analyses, or interpretation of data; in the writing of the manuscript; or in the decision to publish the results.
References
- Qi, J.; Liu, X.; Shen, D.; Miao, H.; Xie, B.; Li, X.; Zeng, P.; Wang, S.; Shang, Y.; Gu, X.; et al. A genomic variation map provides insights into the genetic basis of cucumber domestication diversity. Nat. Genet. 2013, 45, 1510–1515. [Google Scholar] [CrossRef] [PubMed]
- Chomicki, G.; Schaefer, H.; Renner, S.S. Origin domestication of Cucurbitaceae crops: Insights from phylogenies, genomics archaeology. New Phytol. 2020, 22, 1240–1255. [Google Scholar] [CrossRef] [PubMed]
- Weng, Y. Cucumis sativus Chromosome Evolution, Domestication, Genetic Diversity: Implications for Cucumber Breeding. Plant Breed. Rev. 2021, 44, 79–111. [Google Scholar] [CrossRef]
- Lv, J.; Qi, J.; Shi, Q.; Shen, D.; Zhang, S.; Shao, G.; Li, H.; Sun, Z.; Weng, Y.; Shang, Y.; et al. Genetic diversity population structure of cucumber (Cucumis sativus L.). PLoS ONE 2012, 7, e46919. [Google Scholar] [CrossRef]
- Sharma, S.; Kumar, R.; Sharma, H.R.; Sharma, A.; Gautam, N. Divergence Studies for Different Horticultural Traits in Cucumber (Cucumis sativus L.). Int. J. Curr. Microbiol. Appl. Sci. 2018, 7, 1733–1741. [Google Scholar] [CrossRef]
- Priyadarshini, M.; Das, S.; Muduli, K.C.; Mohanty, S.; Sahoo, S.; Pradhan, B.R. Characterization of cucumber genotypes through seed morphological characters. J. Pharm. Phytochem. 2012, 10, 2158–2161. [Google Scholar]
- Butnariu, M.; Butu, A. Chemical composition of Vegetables their products. Handb. Food Chem. 2015, 20, 627–692. [Google Scholar]
- Aderinola, T.A.; Abaire, K.E. Quality acceptability, nutritional composition antioxidant properties of carrot-cucumber juice. Beverages 2019, 5, 15. [Google Scholar] [CrossRef]
- Available online: https://www.haifa-group.com/files/Guides/Cucumber.pdf (accessed on 11 January 2022).
- Chen, J.F.; Zhang, S.L.; Zhang, X.G. The Xishuangbanna gourd (Cucumis sativus var. xishuangbannesis Qi et Yuan), a traditionally cultivated plant of the Hanai People, Xishuangbanna, Yunnan, China. Cucurbit Genet. Coop. Rpt. 1994, 17, 18–20. [Google Scholar]
- Navazio, J.P.; Simon, P.W. Diallel analysis of high carotenoid content in cucumbers. J. Am. Soc. Hortic. Sci. 2001, 126, 100–104. [Google Scholar] [CrossRef]
- Ranjan, P.; Pey, A.; Munshi, A.D.; Bhardwaj, R.; Gangopadhyay, K.K.; Malav, P.K.; Kumar, A. Orange-fleshed cucumber (Cucumis sativus var. sativus L.) germplasm from North-East India: Agro-morphological, biochemical evolutionary studies. Genet. Resour. Crop Evol. 2019, 66, 1217–1230. [Google Scholar] [CrossRef]
- Renner, S.S. A valid name for the Xishuangbanna gourd, a cucumber with carotene-rich fruits. PhytoKeys 2017, 85, 87. [Google Scholar] [CrossRef]
- Burger, Y.; Paris, H.S.; Cohen, R.; Katzir, N.; Tadmor, Y.; Lewinsohn, E.; Schaffer, A.A. 3 Genetic Diversity of Cucumis melo. Hort. Rev. 2010, 36, 165–198. [Google Scholar]
- Wiseman, E.M.; Bar-El Dadon, S.; Reifen, R. The vicious cycle of vitamin a deficiency: A review. Crit. Rev. Food Sci. Nutri. 2017, 57, 3703–3714. [Google Scholar] [CrossRef]
- Simon, P.W.; Navazio, J.P. Early orange mass 400, early orange mass 402, late orange mass 404: High β-carotene cucumber germplasm. Hort. Sci. 1997, 32, 144–145. [Google Scholar] [CrossRef]
- Qi, C.Z.; Yuan, Z.Z.; Li, Y.X. A new type of cucumber-Xishuangbanna cucumber. Acta Hortic. Sin. 1983, 10, 259–264. [Google Scholar]
- Yang, S.L.; Pu, H.; Liu, P.Y. Preliminary studies on Cucumis sativus var. xishuangbannanesis. Cucurbit Genet. Coop. Rpt. 1991, 14, 29–31. [Google Scholar]
- Navazio, J.P. Utilization of High β-Carotene Cucumber Germplasm for Genetic Improvement of Nutritional Quality. Ph.D. Thesis, University of Wisconsin, Madison, WI, USA, 1994. [Google Scholar]
- Staub, J. QTL analyses of orange color carotenoid content mapping of carotenoid biosynthesis genes in cucumber (Cucumis sativus L.). In Proceedings of the IV International Symposium on Cucurbits, Changsha, China, 21–26 September 2009; Volume 871, pp. 607–614. [Google Scholar]
- Cuevas, H.E.; Song, H.; Staub, J.E.; Simon, P.W. Inheritance of β-carotene-associated flesh color in cucumber (Cucumis sativus L.) fruit. Euphytica 2010, 17, 301–311. [Google Scholar] [CrossRef]
- Staub, J.E.; Simon, P.W.; Cuevas, H.E. Usda, Ars Eom 402-10 high β-carotene cucumber. Hortic. Sci. 2011, 46, 1426–1427. [Google Scholar] [CrossRef]
- Bo, K.; Song, H.; Shen, J.; Qian, C.; Staub, J.E.; Simon, P.W.; Chen, J. Inheritance mapping of the Ore gene controlling the quantity of β-carotene in cucumber (Cucumis sativus L.) endocarp. Mol. Breed. 2012, 30, 335–344. [Google Scholar] [CrossRef]
- Che, G.; Zhang, X. Molecular basis of cucumber fruit domestication. Curr. Opin. Plant Biol. 2019, 47, 38–46. [Google Scholar] [CrossRef]
- Lu, H.W.; Miao, H.; Tian, G.L.; Wehner, T.C.; Gu, X.F.; Zhang, S.P. Molecular mapping cidate gene analysis for yellow fruit flesh in cucumber. Mol. Breed. 2015, 35, 64. [Google Scholar] [CrossRef]
- Waters, B.M.; Kim, H.; Amundsen, K. New genetic sources for orange color in cucumber (Cucumis sativus L.) fruit flesh. bioRxiv 2019, 1, 685289. [Google Scholar]
- Bo, K.; Ma, Z.; Chen, J.; Weng, Y. Molecular mapping reveals structural rearrangements quantitative trait loci underlying traits with local adaptation in semi-wild Xishuangbanna cucumber (Cucumis sativus L. var. xishuangbannanesis Qi et Yuan). Theor. Appl. Genet. 2015, 128, 25–39. [Google Scholar] [CrossRef] [PubMed]
- Pan, Y.; Qu, S.; Bo, K.; Gao, M.; Haider, K.R.; Weng, Y. QTL mapping of domestication diversifying selection related traits in round-fruited semi-wild Xishuangbanna cucumber (Cucumis sativus L. var. xishuangbannanesis). Theor. Appl. Genet. 2017, 130, 1531–1548. [Google Scholar] [CrossRef] [PubMed]
- Tian, Z.; Jahn, M.; Qin, X.; Obel, H.O.; Yang, F.; Li, J.; Chen, J. Genetic Transcriptomic Analysis Reveal the Molecular Basis of Photoperiod-Regulated Flowering in Xishuangbanna Cucumber (Cucumis sativus L. var. Xishuangbannesis Qi et Yuan). Genes 2021, 12, 1064. [Google Scholar] [CrossRef] [PubMed]
- Bo, K.; Chen, L.; Qian, C.; Zhang, S.; Chen, J. Short-day treatments induce flowering of Xishuangbanna cucumber. China Cucurbits Veg. 2010, 23, 1–3. [Google Scholar]
- Xu, X.; Lu, L.; Zhu, B.; Xu, Q.; Qi, X.; Chen, X. QTL mapping of cucumber fruit flesh thickness by SLAF-seq. Sci. Rep. 2015, 5, 15829. [Google Scholar] [CrossRef]
- Garrido-Cardenas, J.A.; Mesa-Valle, C.; Manzano-Agugliaro, F. Trends in plant research using molecular markers. Planta 2018, 247, 543–557. [Google Scholar] [CrossRef]
- Kage, U.; Kumar, A.; Dhokane, D.; Karre, S.; Kushalappa, A.C. Functional molecular markers for crop improvement. Crit. Rev. Biotechnol. 2016, 36, 917–930. [Google Scholar] [CrossRef]
- Ahmad, F.; Akram, A.; Farman, K.; Abbas, T.; Bibi, A.; Khalid, S.; Waseem, M. Molecular Markers Marker Assisted Plant Breeding. Current Status their Applications in Agricultural Development. J. Environ. Agric. Sci. 2017, 11, 35–50. [Google Scholar]
- Feng, S.; Zhang, J.; Mu, Z.; Wang, Y.; Wen, C.; Wu, T.; Wang, H. Recent progress on the molecular breeding of (Cucumis sativus L.) in China. Theor. Appl. Genet. 2020, 133, 1777–1790. [Google Scholar] [CrossRef]
- Yan, J.B.; Tang, H.; Huang, Y.Q.; Zheng, Y.L.; Li, J.S. Quantitative trait loci mapping epistatic analysis for grain yield and yield components using molecular markers with an elite maize hybrid. Euphytica 2006, 149, 121–131. [Google Scholar] [CrossRef]
- Hu, J.B.; Zhou, X.Y.; Li, J.W. Development of novel EST-SSR markers for cucumber (Cucumis sativus) their transferability to related species. Sci. Hortic. 2010, 125, 534–538. [Google Scholar] [CrossRef]
- Qu, S.P.; Pan, Y.P.; Weng, Y.I.Q.U.N. QTL Mapping of flowering time and fruit shape in Xishuangbana cucumber (Cucumis sativus L. var. xishuangbannanesis Qi et Yuan). In Proceedings of the Cucurbitaceae, East Lansing, MI, USA, 21–26 October 2014; Volume 2014, pp. 54–56. [Google Scholar]
- Asins, M.J. Present future of quantitative trait locus analysis in plant breeding. Plant Breed. 2002, 121, 281–291. [Google Scholar] [CrossRef]
- Bo, K.; Wei, S.; Wang, W.; Miao, H.; Dong, S.; Zhang, S.; Gu, X. QTL mapping genome-wide association study reveal two novel loci associated with green flesh color in cucumber. BMC Plant Biol. 2019, 19, 243. [Google Scholar] [CrossRef]
- Kooistra, E. Inheritance of fruit flesh skin colors in powdery mildew resistant cucumbers (Cucumis sativus L.). Euphytica 1997, 20, 521–523. [Google Scholar] [CrossRef]
- Cunningham, F.X., Jr.; Gantt, E. Genes enzymes of carotenoid biosynthesis in plants. Annu. Rev. Plant Biol. 1998, 49, 557–583. [Google Scholar] [CrossRef]
- Cazzonelli, C.I.; Pogson, B.J. Source to sink: Regulation of carotenoid biosynthesis in plants. Trends Plant Sci. 2010, 15, 266–274. [Google Scholar] [CrossRef]
- Lado, J.; Zacarías, L.; Rodrigo, M.J. Regulation of carotenoid biosynthesis during fruit development. Carotenoids Nat. 2016, 79, 161–198. [Google Scholar] [CrossRef]
- Sun, T.; Li, L. Toward the ‘golden’ era: The status in uncovering the regulatory control of carotenoid accumulation in plants. Plant Sci. 2020, 290, 110331. [Google Scholar] [CrossRef]
- Lado, J.; Zacarías, L.; Gurrea, A.; Page, A.; Stead, A.; Rodrigo, M.J. Exploring the diversity in Citrus fruit coloration to decipher the relationship between plastid ultrastructure carotenoid composition. Planta 2015, 242, 645–661. [Google Scholar] [CrossRef]
- Li, L.; Yuan, H. Chromoplast biogenesis carotenoid accumulation. Arch. Biochem. Biophys. 2013, 539, 102–109. [Google Scholar] [CrossRef]
- Giuliano, G.; Tavazza, R.; Diretto, G.; Beyer, P.; Taylor, M.A. Metabolic engineering of carotenoid biosynthesis in plants. Trends Biotechnol. 2008, 26, 139–145. [Google Scholar] [CrossRef]
- Zhou, X.; Welsch, R.; Yang, Y.; Álvarez, D.; Riediger, M.; Yuan, H.; Li, L. Arabidopsis OR proteins are the major posttranscriptional regulators of phytoene synthase in controlling carotenoid biosynthesis. Proc. Natl. Acad. Sci. USA 2015, 11, 3558–3563. [Google Scholar] [CrossRef]
- Yuan, H.; Owsiany, K.; Sheeja, T.; Zhou, X.; Rodriguez, C.; Li, Y.; Welsch, R.; Chayut, N.; Yang, Y.; Thannhauser, T.W.; et al. A single amino acid substitution in an ORANGE protein promotes carotenoid overaccumulation in Arabidopsis. Plant Physiol. 2015, 169, 421–431. [Google Scholar] [CrossRef]
- Lu, S.; Van Eck, J.; Zhou, X.; Lopez, A.B.; O’Halloran, D.M.; Cosman, K.M.; Li, L. The cauliflower or gene encodes a DnaJ cysteine-rich domain-containing protein that mediates high levels of β-carotene accumulation. Plant Cell 2006, 18, 3594–3605. [Google Scholar] [CrossRef]
- Tzuri, G.; Zhou, X.; Chayut, N.; Yuan, H.; Portnoy, V.; Meir, A.; Sa’Ar, U.; Baumkoler, F.; Mazourek, M.; Lewinsohn, E.; et al. A ‘golden’ SNP in CmOr governs the fruit flesh color of melon (Cucumis melo). Plant J. 2015, 82, 267–279. [Google Scholar] [CrossRef]
- Chayut, N.; Yuan, H.; Ohali, S.; Meir, A.; Sa’ar, U.; Tzuri, G.; Tadmor, Y. Distinct mechanisms of the ORANGE protein in controlling carotenoid flux. Plant Physiol. 2017, 173, 376–389. [Google Scholar] [CrossRef]
- Chayut, N.; Yuan, H.; Ohali, S.; Meir, A.; Sa’Ar, U.; Tzuri, G.; Zheng, Y.; Mazourek, M.; Gepstein, S.; Zhou, X.; et al. Syntenic relationships between cucumber (Cucumis sativus L.) melon (C. melo L.) chromosomes as revealed by comparative genetic mapping. BMC Genom. 2011, 12, 376–389. [Google Scholar] [CrossRef]
- Rajasree, V.; Pugalendhi, L. Breeding Vegetables for Nutritional Security. In Veganism—A Fashion Trend or Food as a Medicine; IntechOpen: London, UK, 2021. [Google Scholar]
- Sapir, M.; Oren-Shamir, M.; Ovadia, R.; Reuveni, M.; Evenor, D.; Tadmor, Y.; Nahon, S.; Shlomo, H.; Chen, L.; Meir, A.; et al. Molecular aspects of Anthocyanin fruit tomato in relation to high pigment-1. J. Hered. 2008, 99, 292–303. [Google Scholar] [CrossRef] [PubMed]
- Zhang, W.; Hao, H.; Ma, L.; Zhao, C.; Yu, X. Tetraploid muskmelon alters morphological characteristics improves fruit quality. Sci. Hortic. 2010, 125, 396–400. [Google Scholar] [CrossRef]
- Singh, J.; Sharma, S.; Kaur, A.; Vikal, Y.; Cheema, A.K.; Bains, B.K.; Hossain, F. Marker-assisted pyramiding of lycopene-ε-cyclase, β-carotenehydroxylase1 opaque2 genes for development of biofortified maize hybrids. Sci. Rep. 2021, 11, 12642. [Google Scholar] [CrossRef] [PubMed]
- Robbins, M.D.; Casler, M.; Staub, J.E. Pyramiding QTL for multiple lateral branching in cucumber using nearly isogenic lines. Mol. Breed. 2008, 22, 131–139. [Google Scholar] [CrossRef]
- Staub, J.E.; Sun, Z.; Chung, S.M.; Lower, R.L. Evidence for collinearity among genetic linkage maps in cucumber. Hortic. Sci. 2007, 42, 20–27. [Google Scholar]
- Kim, J.S.; Ezura, K.; Lee, J.; Ariizumi, T.; Ezura, H. Genetic engineering of parthenocarpic tomato plants using transient SlIAA9 knockdown by novel tissue-specific promoters. Sci. Rep. 2019, 9, 18871. [Google Scholar] [CrossRef]
- Marzougui, N.; Boubaya, A.; Elfalleh, W.; Ferchichi, A.; Beji, M. Polyploidy induction in Trigonella foenum-graecum L.: Morphological chemical comparison between diploids induced autotetraploids cultivars. Acta Bot. Gall. 2009, 156, 379–389. [Google Scholar] [CrossRef]
- Mason, A.S. Polyploidy Hybridization for Crop Improvement; CRC Press: Boca Raton, FL, USA, 2017. [Google Scholar]
- Huang, S.; Li, R.; Zhang, Z.; Li, L.; Gu, X.; Fan, W.; Lucas, W.J.; Wang, X.; Xie, B.; Ni, P.; et al. The genome of the cucumber, Cucumis sativus L. Nat. Genet. 2009, 41, 1275–1281. [Google Scholar] [CrossRef]
- Kumar, A.; Anju, T.; Kumar, S.; Chhapekar, S.; Sreedharan, S.; Singh, S.; Choi, S.; Ramchiary, N.; Lim, Y.P. Integrating Omics Gene Editing Tools for Rapid Improvement of Traditional Food Plants for Diversified Sustainable Food Security. Int. J. Mol. Sci. 2021, 22, 8093. [Google Scholar] [CrossRef]
- Brunetti, A.E.; Neto, F.C.; Vera, M.C.; Taboada, C.; Pavarini, D.P.; Bauermeister, A.; Lopes, N.P. An integrative omics perspective for the analysis of chemical signals in ecological interactions. Chem. Soc. Rev. 2018, 47, 1574–1591. [Google Scholar] [CrossRef]
- Perez-De-Castro, A.M.; Vilanova, S.; Canizares, J.; Pascual, L.; Blanca, J.; Diez, M.J.; Prohens, J.; Pico, B. Application of genomic tools in plant breeding. Curr. Genom. 2012, 13, 179–195. [Google Scholar] [CrossRef]
- Saha, S.K.; Saikot, F.K.; Rahman, M.S.; Jamal, M.A.H.M.; Rahman, S.K.; Islam, S.R.; Kim, K.H. Programmable molecular scissors: Applications of a new tool for genome editing in biotech. Mol. Ther. Nucleic Acids 2019, 14, 212–238. [Google Scholar] [CrossRef]
- Jayaraj, K.L.; Thulasidharan, N.; Antony, A.; John, M.; Augustine, R.; Chakravartty, N.; Sukumaran, S.; Maheswari, M.U.; Abraham, S.; Thomas, G.; et al. Targeted editing of tomato carotenoid isomerase reveals the role of 5′ UTR region in gene expression regulation. Plant Cell Rep. 2021, 40, 621–635. [Google Scholar] [CrossRef]
- Sun, B.; Jiang, M.; Zheng, H.; Jian, Y.; Huang, W.L.; Yuan, Q.; Tang, H.R. Color-related chlorophyll carotenoid concentrations of Chinese kale can be altered through CRISPR/Cas9 targeted editing of the carotenoid isomerase gene BoaCRTISO. Hortic. Res. 2020, 7, 161. [Google Scholar] [CrossRef]
- Thorup, T.A.; Tanyolac, B.; Livingstone, K.D.; Popovsky, S.; Paran, I.; Jahn, M. Cidate gene analysis of organ pigmentation loci in the Solanaceae. Proc. Natl. Acad. Sci. USA 2000, 97, 11192–11197. [Google Scholar] [CrossRef]
- Guzman, I.; Hamby, S.; Romero, J.; Bosl, P.W.; O’Connell, M.A. Variability of carotenoid biosynthesis in orange colored Capsicum spp. Plant Sci. 2010, 179, 49–59. [Google Scholar] [CrossRef]
- Cao, J.; Schneeberger, K.; Ossowski, S.; Günther, T.; Bender, S.; Fitz, J.; Weigel, D. Whole-genome sequencing of multiple Arabidopsis thaliana populations. Nat. Genet. 2011, 43, 956–963. [Google Scholar] [CrossRef]
- Weber, A.; Clark, R.M.; Vaughn, L.; Sánchez-Gonzalez, J.D.J.; Yu, J.; Yandell, B.S.; Bradbury, P.; Doebley, J. Major regulatory genes in maize contribute to sting variation in teosinte (Zea mays ssp. parviglumis). Genetics 2011, 177, 2349–2359. [Google Scholar] [CrossRef]
- Ahmed, F.; Fanning, K.; Netzel, M.; Schenk, P.M. Induced carotenoid accumulation in Dunaliella salina Tetraselmis suecica by plant hormones UV-C radiation. Appl. Microbiol. Biotechnol. 2015, 99, 9407–9416. [Google Scholar] [CrossRef]
- Stanley, L.; Yuan, Y.W. Transcriptional regulation of carotenoid biosynthesis in plants: So many regulators, so little consensus. Front. Plant Sci. 2019, 10, 1017. [Google Scholar] [CrossRef]
- Pizarro, L.; Stange, C. Light-dependent regulation of carotenoid biosynthesis in plants. Cienc. E Investig. Agrar. 2009, 36, 143–162. [Google Scholar] [CrossRef]
- Martel, C.; Vrebalov, J.; Tafelmeyer, P.; Giovannoni, J.J. The tomato MADS-box transcription factor Ripening Inhibitor interacts with promoters involved in numerous ripening processes in a Colorless Non-ripening dependent manner. Plant Physiol. 2011, 157, 1568–1579. [Google Scholar] [CrossRef]
- Pandolfini, T. Seedless fruit production by hormonal regulation of fruit set. Nutrients 2009, 1, 168–177. [Google Scholar] [CrossRef]
- Hasan, N.; Choudhary, S.; Naaz, N.; Sharma, N.; Laskar, R.A. Recent advancements in molecular marker-assisted selection applications in plant breeding programmes. J. Genet. Eng. Biotechnol. 2021, 19, 128. [Google Scholar] [CrossRef]